ABSTRACT
The volatile profiles of broiled minced pork cutlets with different beef additions (0–15%) and a commercial product (i.e. containing also nonmeat ingredients) were investigated after separation and freezing of the inner part. Specific volatile compounds were used to distinguish the meat products from the product containing nonmeat ingredients. The volatile compounds were not correlated with the pH of the raw material. The fat oxidation predominated over nonenzymic reactions: pyrazines, pyrroles, oxazoles, thiophenes, and thiazoles, which are characteristic products of the Maillard reaction, were absent. The addition of 15% beef to the pork did not influence the profile of volatile compounds.
Introduction
Broiled, roasted, baked, and fried meat is attractive for consumers because of its specific sensory features. Its attractiveness is related to a complex mixture of components and their interaction, such as the formation of a fingerprint of both nonvolatile compounds (brown pigments) and volatile compounds during broiling.[Citation1,Citation2] All of these compounds are produced as a result of the reaction of nonenzymatic browning (Maillard reaction) with the degradation of fat, protein, carbohydrates, and other compounds.[Citation3–Citation6] A good way for assessing the meat quality traits is to study the thermally modified profile of volatile compounds, which reflects the extent of nonenzymatic browning reactions as well as the properties of the raw material, such as the degree of fat oxidation and the presence of nonmeat ingredients.[Citation7,Citation8]
The volatile compounds can be classified into lipid- and phospholipid-derived, formed as a result of the Maillard reactions, as well as those derived from reactions between both the groups mentioned above.[Citation4,Citation5] Volatile substances derived from fat oxidation in meat are aldehydes, ketones, alcohols, carboxylic acids, and esters. However, these compounds can also be produced in the Maillard reaction.[Citation9] The composition of volatile compounds characteristic for the broiled surfaces of meat was strongly correlated with the degree of nonenzymic browning, but not in the internal areas of the samples.[Citation10] This shows that the reactions taking place in the inner parts of the broiled meat were different from those occurring on the surface due to the thermic difference between the two layers. During broiling, roasting, baking, and frying of meat cutlets, the surface is heated to even more than 200°C, but the inner parts reach only 70–80°C.[Citation5] Therefore, the profile of volatile compounds present on the surface is different compared to the inner layers of the product. Furthermore, the same author[Citation5] demonstrated that the amount of volatile compounds formed on the surface layers of the meat was significantly influenced by the pH. This was an additional proof that the dominating surface reaction is the nonenzymic browning, because pH is a major factor affecting the extent of the Maillard reaction and the formation of the aroma compounds.[Citation9–Citation11]
To analyze volatile compounds in the meat products, most researchers prepare representative samples containing the sum of surface and inner parts.[Citation12] However, separate analysis of the inners or surfaces could give more accurate information on the chemical reactions occurring in the meat upon heating. The aim of this study was to characterize the profile of volatile compounds being formed in the layers under the surface of broiled and frozen meat cutlets; to identify significant compounds characterizing the quality of the inner parts of broiled and then frozen meat cutlets; to investigate the influence of the initial pH of the fresh meat on the types and amount of significant volatile compounds formed after broiling under the surface layers; and to analyze the influence of the meat composition on the formation of these substances. Meat samples were prepared with different concentrations of minced pork and beef and were compared with a commercial product containing meat and nonmeat ingredients.
Materials and methods
The meat samples used in the present study were pork (porkneck) and beef (haunch). The raw meat was obtained from a slaughterhouse the previous day. The visible fat was removed from the meat. Inter-tissue fat present in the beef was left intact. Pork neck contained some inter-muscular fat, which was not removed. The meat was divided into portions with a weight of about 0.5 kg each and packed into wrapping films. Most of the portions were later frozen after the removal of air from the package. About 1 kg of the meat was left nonfrozen and kept in the refrigerator at +4°C for two days. Successively, this aliquot was minced and used for the preparation of the first series of samples (containing 0, 5, 10, and 15% w/w beef addition to pork). Another two aliquots of frozen meat samples were kept at −28°C and later thawed at 4°C during 12 h and minced just before formulation. In this way, two more series of samples were obtained with different initial pH, and were then formulated from the same type of raw material as the first series of samples.
Therefore, three different series of cutlets were prepared in total: samples with only pork meat (with a pH of 5.60, 5.65, and 5.31, respectively), pork samples with 5% beef (with a pH of 6.05, 5.42, and 5.51, respectively), samples of pork with 10% beef (pH 6.34, 5.66, and 5.70, respectively), and pork samples with 15% beef (pH 6.00, 5.43, and 6.20, respectively). The weight and height of each cutlet were 80 g and 3 cm, respectively. No other ingredients were added to the meat.
In addition, a market product (with the commercial name of “Rib Burger”) was purchased from a local shop. This product was marked as “hamburger” (H, with a pH 5.80). This commercial hamburger was a ready-made frozen product prepared from a roll and cutlet with the addition of sauces and vegetables. The roll, sauces, and vegetables were discarded and the cutlet was washed with water, dried with blotting paper, and later subjected to analysis. The composition of the cutlet was as follows: 87% cooked beef (partially finely minced), wheat flour used for coating, onion, salt, whole egg in powder, lactose, dextrose, maltodextrin, sugar, spices, aroma, ascorbic acid as antioxidant, and citric acid as acidity regulator. In this experimentation, this cutlet was regarded as a meat product containing nonmeat ingredients. The pH of the raw material was measured with a pH meter mod. CP-411 (Elmetron, Poland). Each sample was investigated in triplicate.
Thermal treatment
The cutlets were subjected to broiling inside the chamber of a gastronomic roaster from Philips (mod. HD4454/A). The roaster was preheated at 185 ± 5°C. The temperature was controlled with a thermocouple. No additional fat was used during the broiling process. The meat was broiled for 30 min (15 min for each side of the cutlet). The inner part of the cutlet reached a final temperature of about 70°C. The “Rib Burger” was regenerated in the above-mentioned roaster at a temperature of 185 ± 5°C to attain the temperature of 70°C in the inner part of the product.
Sample preparation for the analysis
After cooling the cutlets and hamburgers, the layers of the broiled surface as well as the internal parts (with a thickness of about 1 mm) were removed with a scalpel. Both layers were weighed (RadWag WPA 180). The broiled surface as well as the internal parts was separately minced, portioned into samples, and packed into wrapping films and later frozen at −28°C for further analysis. The same procedure was performed for the hamburgers.
Analysis of volatile compounds
The minced samples obtained from the inner parts were thawed prior to analysis. Samples were weighed to 0.5 g in vials. The optimal amount of weight of the samples was determined experimentally. A methanol solution of an internal standard (IS) (1,2-dichlorobenzene with 99% purity, Fluka) was added to the samples. The total amount of the added IS was 1.306 µg. The samples were kept thermostatically for 20 min prior to the determination of volatile compounds by means of a solid-phase microextraction (SPME) fiber (75 µm CAR/PDMS – carboxen/polydimethylsiloxane) for 30 min at 60°C. The manual holder was used during the extraction process. The determination of volatile compounds was carried out with a GC/MS Mod. GCMS-QP 2010 from Shimadzu equipped with a ZB-WAX Plus column (30 m × 0.25 µm × 0.25 mm) and an injector set at 200°C. The oven temperature increased from 40 to 220°C at the rate of 4°C/min. The helium pressure was set at 35.2 kPa with a flow rate of 22.8 ml/min. The temperature of the ion source was 170°C. The samples were prepared and measured in triplicates.
The identification of volatiles substances was performed by comparing the acquired mass spectrum (MS) with the mass spectra from authentic compounds previously analyzed and with spectra from different libraries (NIST 147, PAL 600, and NIST 02). The linear retention index (LRI) was calculated on the basis of the standard hydrocarbons C8–C20 (Fluka) and the recent specific literature.[Citation13–Citation16] The content of volatile compounds was calculated semiquantitatively in relation to the surface of the peak of the IS, whose value was assumed as 1000. All the determinations of the volatile compounds were carried out in triplicate.
Statistical analysis
The raw data were subjected to analysis of variance (ANOVA), factor analysis, and principal components analysis (PCA) with the use of the statistical software Statistica ver. 9.0 (Statistica, Tulsa, USA).
Results
Pork neck was chosen for two distinct reasons. First, it is a very popular thermally treated meat in Europe, and second, because of scarcity of data in the scientific literature regarding the processes taking place in this meat during broiling. In contrast, pork loin is the most tested meat. Beef was added to pork in different proportions to obtain a product with better sensory features, but with a lower amount of fat. This allowed investigating the influence of the meat composition on the profile of the volatile compounds. The commercial product (hamburger, containing 100% beef meat without the addition of pork) was investigated to note similarities and differences in the volatile profile between laboratory-made and commercially made cutlets containing spices and other ingredients.
An average proportion of 86.2 ± 1.8% and 16.0 ± 2.5% (w/w) for the inner parts and the surface of the samples was experimentally measured, respectively. In the layers under the surface of broiled samples of meat and hamburgers (H), the following groups of volatile compounds were identified: aldehydes, ketones, alcohols, carboxylic acids, esters, furan derivatives, sulfur derivatives, and nitrogen derivatives (the two last groups of compounds were found in one sample) as well as other, mainly sensory-active compounds. The list of retention indexes is presented in . Each compound was confirmed by the MS and LRI.
Table 1. List of volatile compounds identified in meat products under the layers of the broiled surface.
Aldehydes
The amount (calculated as a part of the IS area) of individual aldehydes differed significantly among the samples (p = 0). All the aldehydes identified in the layers under the surface of the samples are reported in . Hexanal was the most detected aldehyde, followed by pentanal. The amount of hexanal was significantly higher than all other aldehydes (p < 0.05). Its amount was statistically lower in H samples with respect to the other products. Hexanal, heptanal, nonanal, and pentanal were found in all the examined samples. Octanal was not detected in samples of hamburger (H). Benzaldehyde was not found in one sample of meat containing 10% w/w of the beef, but it was detected in all the other samples, including hamburgers. Propanal was not identified in only one sample of meat, containing 5% (w/w) of beef as well as in the sample of hamburger. (Z)-Hept-2-enal was found in most of the samples (8 out of the 13 samples), but the remaining other aldehydes were detected only in less than 50% of the meat samples. Butanal and (E)-oct-2-enal were found in four samples, 3-methylbutanal in two samples, while (E)-hex-2-enal in only one sample. The average total amount of all aldehydes and the types of aldehydes in the layers under the surface did not depend on the pH of the samples (p > 0.05).
Figure 1. Types and index of amount, in relation to the IS, of the aldehydes (a), ketones (b), alcohols (c), carboxylic acids (d), esters (e), furan derivatives (f), and other compounds (g) identified in the inner parts of the broiled cutlets. Sample codes: 0% beef, 5% beef, 10% beef, 15% beef – pork cutlets, containing different concentrations of beef haunch added to the pork neck (0, 5%, 10%, and 15% (w/w), respectively); H, hamburger.
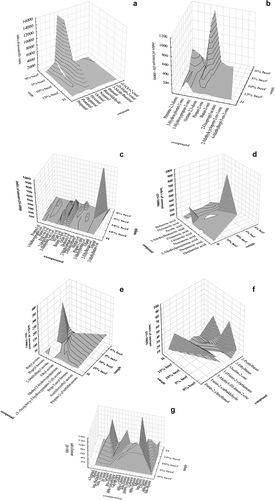
Ketones
Ketones found in the layers under the surface of broiled samples of meat and hamburger are presented in . Octane-2,3-dione was the most dominant ketone (p < 0.05 in comparison to other volatile compounds) in meat model samples. Octane-2,3-dione was followed by 3-hydroxybutan-2-one and pentane-2,3-dione. The ANOVA of the sum of ketones indicated that a significantly lower amount of ketones (p < 0.05) was present in the hamburgers. The dominant ketone in these samples was 3-hydroxybutan-2-one. Also in this case, the average amount of ketones as well as the individual amount did not depend on the pH (p > 0.05).
Alcohols
A total of 19 different alcohols were determined. The significant highest amount (p < 0.05) of alcohols was in pork cutlets without the addition of beef, and the lowest amount was detected in the H sample. Pentan-1-ol was the main alcohol, followed by ethanol (only in two samples), pent-1-en-3-ol, and oct-1-en-3-ol. The sum of alcohols in the layers under the surface of the broiled samples as well as the individual amount was not related to the pH level (p > 0.05). The range of alcohols in the layers under the surface of the broiled samples is presented in .
Carboxylic acids
The average amount of carboxylic acids in the layers under the surface was independent of the pH (p > 0.05). Acetic acid was the main carboxylic acid, but since it was not detected in most of the samples, it was not significant in ANOVA. The types and the amount of carboxylic acids in the layers under the broiled surface of samples are presented in . Butanoic acid and hexanoic acid were detected in most of the samples. In contrast, the other carboxylic acids were found in the hamburger. This may be related to the use of nonmeat ingredients and/or to higher fat content than in the other products containing only meat (which were not added with fat).
The factor distinguishing the types of individual carboxylic acids formed was the type of sample (p < 0.05). This result was obvious, because the composition of carboxylic acids in the hamburgers was entirely different from the other samples containing only meat. This hypothesis was confirmed with post hoc analysis, which revealed differences only in the hamburger samples. In other words, nonmeat ingredients played an important role in the qualitative and quantitative changes of the volatile compounds formed in meat.
Esters
The amount of esters in the layers under the meat surface did not depend on the composition of raw materials, but depended on the initial pH of the samples. However, post hoc analysis revealed differences in only two samples. Therefore, it was impossible to unequivocally state the relationship between the amount of esters and the pH of the raw material. The types of esters are presented in .
Nine different esters were identified in the internal areas of the broiled meat, but they were not found in six out of the 14 samples analyzed. Five out of the nine detected esters were found only in the hamburger. Only four esters were found in meat samples and they were as follows: butyl acetate, heptyl formate, 2-methylbutyl acetate, and propan-2-yl tetradecanoate. Identification of more esters in the hamburger was probably due to the addition of nonmeat ingredients, mainly spices.
Furan derivatives
The total amount of furan compounds did not depend on the pH (p > 0.05). The range of furan derivatives identified in the layers under the surfaces of broiled samples of meat is presented in . The types of furan derivatives differed significantly between individual samples (p < 0.05), but post hoc analysis showed that only hamburgers differed from the other samples. There were no differences in the content of furan derivatives between meat samples without any added ingredients; 2-pentylfuran was the only furan detected in all the samples. The amount of 2-pentylfuran in all meat samples was similar. Other furan compounds identified in the meats were oxolan-2-one and 2-ethylfuran. They were identified in two different samples.
Sulfur derivatives
Only two sulfur derivatives, namely butane-2-thiol and (methyldisulfanyl)methane, were identified in the layers under the surface of the hamburgers. Sulfur derivatives were under the detection limit in the meat samples without nonmeat ingredients.
Other compounds
Volatile compounds that mostly contribute to the sensory profile of meat were identified in the inner layers of the samples. Most of them were detected in the hamburger () and only two were found in meat, namely 7-methyl-3-methylideneocta-1,6-diene (beta-myrcene) and 2-methyl-5-propan-2-ylphenol (carvacrol). The source of these compounds in meat was probably the animal diet,[Citation17] but their presence in hamburgers could be attributed to nonmeat ingredients, especially spices.
Statistical elaboration
Overall, 61 different volatile compounds were identified under the surface of broiled samples. The preliminary statistical analysis could not explain the relationships between the types of samples and the amount as well as the types of identified volatile compounds and pH. Therefore, it was necessary to verify whether it was feasible to distinguish significant and nonsignificant variables from the total pool of variables. For this reason, a factor analysis was performed (). Fourteen samples and 69 variables (all volatile compounds and types of samples, pH, beef, and pork composition) were used in the analysis. One variable, namely propan-2-yl tetradecanoate, was eliminated because it was not significant according to the statistical elaboration. As a result of the analysis, four factors were chosen; all of them accounted for 84% of the total variance. Some variables did not correlate with the factors. These were: sample types, pH as well as beef and pork content, (Z)-hept-2-enal, nonanoic acid, propan-2-ol, dodecan-1-ol, 2-hexoxyethanol, heptyl formate, and 2-ethylfuran. These variables were eliminated and a further statistical analysis was performed. Four factors accounted for 94.5% of the total variance. It was not possible to reduce the number of factors to only three, because this could have led to the loss of highly correlated variables (the highest correlation coefficient accounted for 0.73).
Twenty-eight variables correlated strongly with factor 1 (). These variables were: butanal, 3-methylbutanal, hexanal, heptanal, nonanal, octanal, benzaldehyde, propanal, pentanal, butan-2-one, pentane-2,3-dione, 3-hydroxybutan-2-one, 1-hydroxypropan-2-one, octane-2,3-dione, propan-2-one, 2-oxopropyl acetate, butanoic acid, hexanoic acid, butan-1-ol, pent-1-en-3-ol, pentan-1-ol, hexan-1-ol, octan-1-ol, oct-1-en-3-ol, heptan-1-ol, butyl acetate, 2-methylbutyl acetate, and 2-pentylfuran. Thirteen variables highly correlated with factor 2; these were 6-methylhept-5-en-2-one, 2-methylcyclopent-2-en-1-one, pentanoic acid, 3-methylbutanoic acid, ethanol, 2-methylpropan-1-ol, 3-methylbutan-1-ol, cyclopentanol, ethyl acetate, (1-acetyloxy-3-hydroxypropan-2-yl) acetate, furan-2-carbaldehyde, 1-(furan-2-yl)ethanone, and 2-methyl-2H-furan-5-one. Factors 3 and 4 correlated with eight and seven variables, respectively: acetic acid, heptanoic acid, octanoic acid, 2-methylpropanoic acid, propanoic acid, prop-2-enyl formate, acetyloxyethyl acetate, and furan-2-ylmethanol; and (E)-oct-2-enal, (E)-pent-2-en-1-ol, 2,3-butanediol, (E)-oct-2-en-1-ol, 2-butoxyethanol, 2-ethylhexan-1-ol, and oxolan-2-one, respectively ().
The compounds correlating with factor 1 were characteristic for several meat products and some of them were also identified in the hamburger. Variables that correlated with factor 2 were typical only for hamburger samples. In the hamburger, all the following compounds were identified: 6-methylhept-5-en-2-one, 2-methylcyclopent-2-en-1-one, pentanoic acid, 3-methylbutanoic acid, 2-methylpropan-1-ol, cyclopentanol, ethyl acetate, (1-acetyloxy-3-hydroxypropan-2-yl) acetate, furan-2-carbaldehyde, 1-(furan-2-yl)ethanone, and 2-methyl-2H-furan-5-one.
Factors 3 and 4 correlated with compounds that were found only in some samples. Therefore, it was assumed that they were not typical for the inner parts of broiled meat. All the significant compounds, which correlated with all four factors, were subjected to PCA (). PCA enabled one to distinguish the meat products from the hamburgers. One sample of meat (containing 15% of beef) differed from the others (outlier). The ANOVA showed that the cause of this difference was the presence of butane-2,3-diol, which was present in the sample containing 15% of beef only in traces.
Discussion
Most of the volatile compounds identified in the layers under the surface of broiled samples of meat were distinctive for pork and beef. The results of the present study are in agreement with earlier data regarding the presence of volatile compounds in meat[Citation16,Citation18] and derivatives of lipid oxidation.[Citation4,Citation5] The main volatiles detected were aldehydes and ketones while alcohols, carboxylic acids, and esters were present in smaller amounts. Hexanal was the most abundant aldehyde found in the meat samples. This aldehyde is distinctive for pork and arises as a result of linoleic acid oxidation.[Citation19] Both hexanal and pentanal can be markers of the chemical oxidation of fat.[Citation18,Citation19] Heptanal can arise as the result of oxidation of linoleic acid.[Citation20] Octanal and nonanal are products of oleic acid oxidation,[Citation19] while oct-2-enal is produced during the degradation of linolenic and arachidonic acids.[Citation21] Benzaldehyde is a compound formed from the oxidation of fat during the thermal treatment of beef.[Citation22] It arises mainly from the breakdown of linolenic acid contained in green plants.[Citation23,Citation24] The only typical aldehyde resulting from Strecker degradation was 3-methylbutanal, which is formed from the amino acid leucine.[Citation22] Nine out of 11 aldehydes were significant as the variables characterizing the quality of the inner parts of meat and hamburger samples.
Ketones, such as octane-2,3-dione, which was found in the highest amount as well as 3-hydroxybutan-2-one and pentane-2,3-dione, were also typical for meat. Octane-2,3-dione is present in the tissues of ruminants-fed fresh green grass,[Citation17] but it is also formed in the Maillard reaction.[Citation25] Hydroxybutan-2-one and 1-hydroxypropan-2-one are distinctive for the early stages of nonenzymatic browning reactions.[Citation5] Although both are typical Maillard reaction products, their amount in the layers under the surface was not pH-related. This meant that in the inner parts of the meat, nonenzymic browning reactions were not intensive, but the fat oxidation was dominant.
6-Methylhept-5-en-2-one and 2-methylcyclopent-2-en-1-one were not characteristic for the meat. Both were identified in the H sample only. Thus, the presence of these ketones in the inner parts of any meat can be ascribed to the addition of nonmeat ingredients to the meat. Most of all significant compounds were alcohols. All of these alcohols could be the result of fat degradation,[Citation21] but some of them (pentan-1-ol, butan-1-ol, hexan-1-ol, and octan-1-ol) can be formed during the Maillard reaction, as the final products of the reaction of lysine with ribose.[Citation10] However, in the meat, the amount of these alcohols was pH independent, and therefore their presence must be the effect of fat oxidation. The characteristic alcohol in meat is oct-1-en-3-ol, which is the typical derivative of the linoleic and/or arachidonic acids oxidation.[Citation19,Citation21]
All carboxylic acids detected in the broiled samples were typical for meat too.[Citation26] The total amount of carboxylic acids was definitely lower than aldehydes, ketones, or alcohols. Most probably the relatively low heating temperature in the internal areas did not favor the progressive transformation of aldehydes and alcohols into other compounds during broiling and thus led to their accumulation in the layers under the meat surface. Aldehydes and alcohols can be formed simultaneously during the decomposition of hydroxyperoxides.[Citation27] This explains the higher content of aldehydes and alcohols with respect to other compounds. Carboxylic acids are tertiary oxidation products of fat oxidation, which are produced as a result of the oxidation of aldehydes.[Citation27] This explains the occurrence of organic acids in a lower amount compared to aldehydes in the layers under the surface of broiled samples. The same explanation applies to esters, which are a result of the interaction between carboxylic acids and alcohols.[Citation28]
Typical volatile compounds produced during the Maillard reactions in the broiled meat are furan derivatives. However, only 2-pentylfuran was detected in all the samples. Many authors[Citation18,Citation29] have identified this compound. 2-Pentylfuran is also typically derived from the oxidation of linoleic acid,[Citation19,Citation23] and because its amount was not pH-dependent, most probably, carboxylic acids were its precursors.
Pyrazines, pyrroles, oxazoles, thiophenes, and thiazoles, which are characteristic products of the Maillard reaction, were not found in the internal layers at all. This result confirms that fat oxidation dominates the Maillard reactions in the layers under the surface; thus, both layers (surface and inner) should be analyzed separately.
As reported in , the initial pH of raw meat, as well as the addition of maximum 15% w/w beef to pork did not have any significant influence on the amount and types of volatile compounds (p > 0.05). Therefore, some other variables influenced the type and amount of volatile substances, most probably variables related with fat oxidation reactions.
Table 2. Results of the ANOVA of the relationships between sample type (grouping variable) and amount (content) of volatile compounds, type of compounds (component) and pH (pH*100); p < 0.05. SS, sum of squares; MS, mean squares; df, degrees of freedom.
Conclusion
There is a wide variety of literature data regarding the profile of volatile compounds produced in meat during thermal treatment. However, most authors determine these compounds mainly in the averaged samples (containing surface and internal layers) of heated meat and pay no attention to the separate zones of the product. Although the inner parts of broiled/roasted/heated meat are characterized by lower heat treatment than the surface – the percentage contribution of the internal areas was 86.2 ± 1.8% with only 16.0 ± 2.5% (w/w) for the broiled surfaces. Therefore, the internal areas contain much more edible matter than the surface. There were statistically significant differences between the amount of individual compounds, based on the analysis of volatile compounds being formed in the inner layers of broiled mixed pork-beef cutlets and commercial hamburgers. The addition of nonmeat ingredients to meat caused the presence of volatile compounds nonspecific for meat in the layers sampled under the surface of a commercial hamburger. 6-Methylhept-5-en-2-one, 2-methylcyclopent-2-en-1-one, pentanoic acid, 3-methylbutanoic acid, 2-methylpropan-1-ol, cyclopentanol, ethyl acetate, (1-acetyloxy-3-hydroxypropan-2-yl) acetate, furan-2-carbaldehyde, 1-(furan-2-yl)ethanone, and 2-methyl-2H-furan-5-one were among them. These compounds can be a marker of the addition of nonmeat ingredients to meat products. The amount of any volatile compound formed in the internal part of the meat did not depend on the pH of the raw material. Therefore, pH had no significant influence on the thermal changes of the inner parts of broiled meat cutlets. In the layers under the surface probably fat oxidation reactions, not nonenzymic browning reactions, were predominant. The reason for the phenomenon was the different distribution of heat distribution on the surfaces and the inner layers of the cutlets during thermal treatment. Aldehydes and ketones were the predominant groups of volatile compounds in the inner layers of broiled meat cutlets. The main source of each of them was most probably the fat deterioration process. The amounts of carboxylic acids, esters, furan derivatives as well as sulfur and nitrogen derivatives (found only in one sample) were lower. In the internal part of meat, no specific Maillard compounds such as pyrazines and pyrroles were detected at all.
References
- Morales, F.J.; van Boekel, M.A.J.S. A Study on Advanced Maillard Reaction in Heated Casein/Sugar Solution: Colour Formation. International Dairy Journal 1998, 8, 907–915.
- Jing, H.; Kitts, D.D. Chemical Characterization of Different Sugar-casein Maillard Reaction Products and Protective Effect on Chemical-induced Cytotoxicity of Caco-2 Cells. Food and Chemical Toxicology 2004, 42, 1833–1844.
- Meynier, A.; Mottram, D.S. The effect of pH on the Formation of Volatile Compounds in Meat-related Model System. Food Chemistry 1995, 52, 361–366.
- Mottram, D.S. Flavour Formation in Meat and Meat Products: A Review. Food Chemistry 1998a, 4, 415–424.
- Mottram, D.S. The Chemistry of Meat Flavor. In Flavor of Meat, Meat Products and Seafoods, 2nd edN.; Shahidi, F.; Ed.; Blackie Academic & Professional: London, 1998b; 5–26.
- Tkacz, K.; Wiek, A.; Kubiak, M. S. Influence of Marinades on the Level of PAHs in Grilled Meat Products. Italian Journal of Food Science 2012, 24, 270–278.
- Warren, H.E.; Scollan, N.D.; Nute, G.R.; Hughes, S.I.; Wood, J.D.; Richardson, R.I. Effects of Breed and a Concentrate or Grass Silage Diet on Beef Quality in Cattle of 3 Ages. II: Meat stability and Flavour. Meat Science 2008, 78, 270–278.
- Stetzer, A.J.; Cadwallader, K.; Singh, T.K.; Mckeith, F.K.; Brewer, M.S. Effect of Enhancement and Ageing on Flavor and Volatile Compounds in Various Beef Muscles. Meat Science 2008, 79, 13–19.
- Yaylayan, V.A. Classification of the Maillard Reaction: A Conceptual Approach. Trends in Food Science and Technology 1997, 1, 13–18.
- Biller, E.; Boselli, E.; Obiedziński, M.; Karpiński, P.; Waszkiewicz-Robak, B. The Profile of Volatile Compounds in the Outer and Inner Parts of Broiled Pork Neck is Strongly Influenced by the Acetic-acid Marination Conditions. Meat Science 2016, 121, 292–301.
- Bell, L.N. Kinetics of Non-enzymatic Browning in Amorphous Solid Systems: Distinguishing the Effects of Water Activity and Glass Transition. Food Research International 1996, 6, 591–597.
- Xie, J.; Sun, B.; Zheng, F.; Wang, Sh. Volatile Flavor Constituents in Roasted Pork of Mini-pig. Food Chemistry 2008, 109, 506–514.
- Mottram, R. The LRI and Odour Database. Flavour Research Group, School of Food Biosciences; University of Reading: UK. http://www.odour.org.uk ( accessed December 2015).
- Boulanger, R.; Crouzet, J. Identification of the Aroma Components of Acerola (Malphigia glabra L.): Free and Bound Flavor Compounds. Food Chemistry 2001, 74, 209–216.
- Kocsis, N.; Amtmann, M.; Mednyánszky, Z.; Korány K. GC-MS Investigation of the Aroma Compounds of Hungarian Red Paprika (Capsicum annuum) cultivars. Journal of Food Composition and Analysis 2002, 15, 195–203.
- Meinert, L.; Andersen, L.T.; Briede, W.L.P.; Bjergegaard, Ch.; Aaslyng, M.D. Chemical and Sensory Characterization of Pan-fried Flavor: Interactions between Raw Meat Quality, Ageing and Frying Temperature. Meat Science 2007, 75, 229–242.
- Vasta, V.; Priolo, A. Ruminant Fat Volatiles as Affected by Diet. A Review. Meat Science 2006, 73, 218–228.
- Tikk, K.; Haugen, J-E.; Andersen, H.J.; Aaslyng, M.D. Monitoring of Warmed-over Flavor in Pork Using the Electronic Nose – Correlation to Sensory Attributes and Secondary Lipid Oxidation Products. Meat Science 2008, 80, 1254–1263.
- Chen, J.; Ho, C.-T. The Flavor of Pork. In Flavor of Meat, Meat Products and Seafoods, 2nd edn.; Shahidi, F.; Ed.; Blackie Academic & Professional: London, 1998; 61–83.
- Descalzo, A. M.; Insani, E. M.; Biolatto, A.; Sancho, A. M.; Garcìa, P. T.; Pensel, N. A. Influence of Pasture or Grain-based Diets Supplemented with Vitamin E on Antioxidant/Oxidative Balance of Argentine Beef. Meat Science 2005, 70, 35−44.
- Calkins, C.R.; Hodgen, J.M. A Fresh Look at Meat Flavor. Meat Science 2007, 77, 63–80.
- Macleod, G. The Flavor of Beef. In Flavor of Meat, Meat Products and Seafoods, 2nd edn.; Shahidi, F.; Ed.; Blackie Academic & Professional: London, 1998; 27–60.
- Elmore, J. S.; Cooper, S. L.; Enser, M.; Mottram, D. S.; Sinclair, L. A.; Wilkinson, R. G. Dietary Manipulation of Fatty Acid Composition in Lamb Meat and Its Effect on the Volatile Aroma Compounds of Grilled Lambs. Meat Science 2005, 69, 233−242.
- Vasta, V.; Luciano, G.; Dimauro, C.; Röhrle, F.; Priolo, A.; Monahan, F.J.; Moloney, A.P. The Volatile Profile of Longissimus Dorsi Muscle of Heifers Fed Pasture, Pasture Silage or Cereal Concentrate: Implication for Dietary Discrimination. Meat Science 2011, 87, 282–289.
- Kerler, J.; Winkel, Ch. The Basic Chemistry and Process Conditions Underpinning Reaction Flavour Production. In Food Flavour Technology; Taylor, A.J.; Ed.; Sheffield Academic Press: Sheffield, UK, 2002; 27–59.
- Olivares, A.; Navarro, J.L.; Flores, M. Distribution of Volatile Compounds in Lean and Subcutaneous Fat Tissues during Processing of Dry Fermented Sausages. Food Research International 2009, 42, 1303–1308.
- deMan, J.M. Principles of Food Chemistry, 3rd edn.; A Chapman & Hall Food Science Book, Aspen Publishers, Inc: Gaithersburg, Maryland, 1999. ( Chapter 2).
- Spurvey, S.; Pan, B.S.; Shahidi, F. Flavour of Shellfish. In Flavor of meat, meat products and seafoods, 2nd edn.; Shahidi, F.; Ed.; Blackie Academic & Professional: London, 1998; 159–196.
- Meinert, L.; Schäfer, A.; Bjergegaard, Ch.; Aaslyng, M.D.; Bredie, W.L.P. Comparison of Glucose, Glucose 6-phosphate, Ribose, and Mannose as Flavor Precursors in Pork; the Effect of Monosaccharide Addition on Flavor Generation. Meat Science 2009, 81, 419–425.