ABSTRACT
The influence of hydrodynamic conditions in the coacervation medium of the carotenoid-rich buriti oil using gelatin-alginate as wall materials was investigated. The stirring speed had strong influence on the size of the microcapsules, and Reynolds number higher than 70000 resulted in particle size D[4,3] lower than 200 μm. The carotenoid encapsulation efficiency was around 80%, with freeze-dried particles presenting intense yellow color. Although the size of the microparticles was highly dependent on the hydrodynamic conditions during coacervate formation, complex coacervation of gelatin and sodium alginate showed to be a feasible process to producing buriti oil microcapsules.
Introduction
Buriti (Mauritia flexuosa L. f.) is a palm tree native from the Amazonian forest that produces fruits with an oily, edible pulp rich in carotenoids, especially β-carotene, and tocopherols.[Citation1] Microencapsulation by complex coacervation may protect these bioactive compounds as well as facilitating their introduction in dry formulations and preventing degradation due to exposure to hazardous ambient factors, including oxygen, light, and moisture.
Complex coacervation is a microencapsulation technique based on the associative interaction between oppositely charged polymers, usually a protein and a polysaccharide. In that process, the material to be encapsulated, most often a lipophilic compound, is initially emulsified in the biopolymer mixture. Subsequently, the conditions of the emulsion (pH, ionic strength, or temperature) are altered in order to promote interaction between the polymers, forming the coacervates. These are deposited on the surface of the emulsified droplets, forming a protective layer and giving rise to microcapsules.[Citation2–Citation5]
The most studied and well-understood coacervation system is the pair gelatin/gum Arabic.[Citation3] Nevertheless, although gum Arabic has been extensively used as encapsulation matrix for oils and flavors, its high cost and irregular availability have motivated research for alternative encapsulation materials.[Citation6] Gelatin is an abundant protein prepared by collagen hydrolysis, which is edible, biocompatible, and positively charged below its isoelectric point, permitting complexation with negatively charged polysaccharides at lower pH.[Citation7] Alginate is an anionic linear polysaccharide containing 1,4-linked D-mannuronic acid and L-guluronic acid residues, which is obtained from brown seaweed using dilute alkaline extraction and can be converted to sodium alginate by treatment with mineral acids. Sodium alginate is a natural, biocompatible, biodegradable, and hydrophilic polymer, which has been applied to micro-particulate formulations for drug delivery mainly because of its bio-adhesion, gelation, and pH-responsive properties.[Citation8] Only a few works have been reported investigating the performance of the pair gelatin-sodium alginate in complex coacervation.[Citation9–Citation12]
The characteristics of the microcapsules and, consequently, the encapsulation efficiency and release profile depend on the processing parameters adopted during coacervation, including type and concentration of wall polymers and core material, pH, presence of surfactants, and use of cross-linking agents. In addition, the agitation speed during coacervation exerts a great influence on the produced microcapsules. There are a great number of publications concerning the performance of different pairs of biopolymers in the complex coacervation of several lipophilic core materials, but only a limited number of works has been published reporting the effect of processing conditions on the microcapsule characteristics. Jegát and Taverdet[Citation13] and Lemetter et al.[Citation14] focused on the effect of stirring speed during coacervation on the size, morphology, and release profile of lipophilic materials encapsulated by complex coacervation in gelatin-gum Arabic, without varying the wall material concentration. To contribute to knowledge of the influence of hydrodynamic conditions during complex coacervation on properties of the resulting microcapsules, the present work was carried out aiming to evaluating the effect of stirring speed and wall polymer concentration on the size and morphology of buriti oil microcapsules produced by complex coacervation of gelatin and sodium alginate.
Materials and methods
Materials
Buriti oil (Amazon Oil, Brazil) was microencapsulated by complex coacervation using gelatin extracted from bovine skin, type B, with gel strength of 240 bloom (Gelita, Brazil) and sodium alginate GRINDSTED® FD 175 (Danisco, Brazil). The pH adjustment of coacervation (3.5 ± 0.1) was performed with 99.7% acetic acid (Dynamica, Brazil). The solutions were prepared using water purified by Milli-Q 3 system (Millipore, Milford, USA).
Methods
Complex coacervation: The microcapsule preparation was carried out according to the treatments shown in . The buriti oil was added to the gelatin solution and homogenized in ultraturrax disperser (IKA, model T25, Germany) at 15,000 rpm for 5 min. The resulting emulsion was heated to 50 ± 3°C, followed by addition of the sodium alginate solution at the same temperature, making up a final volume of 0.2 L. The pH was then adjusted to 3.5 ± 0.1 using acetic acid and a digital pH meter (Tecnal, model 3 MP, Brazil). This step was performed under continuous agitation, using a mechanical stirrer (Marconi, model MA 261, Brazil) with a 0.051 m-width turbine impeller settled in a 0.6 L-capacity vessel (diameter of 0.086 m), maintaining temperature at 50 ± 3°C throughout the process. The configuration of the coacervation vessel was based on the system used by Jegát and Taverdet[Citation13], and the influence of different stirring speeds (600, 950, 1300, 1650, and 2000 rpm) was evaluated. After pH adjustment, the vessel containing the coacervate suspension was immersed into an ice bath and kept under the same stirring speed for slow cooling up to 10 ± 2°C. After cooling, agitation was interrupted, and the vessel was covered with aluminum foil for protection against light, being stored for 24 h in a cold chamber (Consul, 280L, Brazil) at 5°C for complete precipitation of the particles. The excess water was then removed, and the coacervate particles were frozen at −34°C for 24 h (ultra-freezer, model FV 500, Liobrás, Brazil) and freeze dried (model L101, Liobrás, Brazil) for 72 h at temperature lower than −40°C (temperature at the compressor) and pressure of around 7 × 10–3 kPa. The dried samples were macerated in mortar, protected from light, and stored in desiccator for further analyses. The microencapsulation was followed through assessment of the capsule morphology at three steps of the production process: after coacervation, after freeze drying, and after rehydration at 25°C. The samples were placed on slides and analyzed using an optical microscope (Olympus, CX31) coupled to an 8.1 megapixel digital camera (Sony Cyber-Shot, DSC-W150). Hydrodynamic conditions during coacervation: The Reynolds number (Eq. 1) characterized the hydrodynamic conditions during coacervation:
Table 1. Formulation and processing conditions during microencapsulation of buriti oil in alginate-gelatin coacervates.
in which ρ is the density of coacervate suspension in kg⋅m−3, N is the rotational speed in rps, Da is the impeller diameter in m, and ηap is the apparent viscosity (in Pa⋅s) of the coacervate suspension at the shear rate (, in s−1) corresponding to N. The rotational speed was measured using a speedometer (model CC-VL520, Cateye, Japan) attached to the agitator shaft. The shear rate at the rotational speed N was estimated according to Eq. (2)[Citation15]:
in which DV is the vessel diameter in m. The rheological behavior of the coacervate suspensions was evaluated in shear rates varying from 1 to 300 s−1, at 50°C, using a rotational rheometer (model AR2000ex, TA Instruments, New Castle, USA) with concentric cylinders geometry. The Herschel-Bulkley model (Eq. 3) was fitted to the experimental flow curves, and the apparent viscosity was calculated by Eq. (4)[Citation15]:
in which σ0 is the yield stress (mPa), K is the consistency index (mPa⋅sn), and n is the flow behavior index (dimensionless).
Particle size: after freeze drying, the particle size distribution of the microcapsules was determined using a laser diffraction particle size distribution analyzer LA-950V2 (HORIBA, Japan) with anhydrous ethanol (PA) as the dispersing medium. The freeze-dried particles were previously dispersed in ethanol in a mechanical stirrer for 1 min and treated in an ultrasonic bath (model USC-1400A, Unique, Brazil) for 30 s. Particle size was expressed in terms of the volume mean diameter D[4,3], and the width of distribution was expressed by the span (Eq. 5):
in which D90, D50, and D10 are the particle diameters where 90%, 50%, and 10% of the population lie below these values, respectively.
Encapsulation efficiency: the microcapsule efficiency of encapsulating the carotenoids present in the buriti oil was determined as the ratio between the total carotenoid content within the microcapsules (EC) and the total amount of carotenoids present in the oil used in the formulation (TC), according to Eq. (6). Disruption of the microcapsules was achieved using a sodium citrate solution at 3%.
The oil was quantified by extraction with acetone, and the total carotenoid content was determined by spectrometry according to Rodriguez-Amaya and Kimura.[Citation16] The total carotenoids present in approximately 0.7 g of microcapsules were extracted with acetone using ultraturrax homogenizer (IKA, model T25, Germany). The homogenate was filtered under vacuum through a funnel with sintered glass plate (G2 porosity), and the extractions continued until the residue appeared devoid of color. The extract was added to a separator funnel containing approximately 10 mL of petroleum ether, followed by 400 mL of distilled water, and, after phase separation, the lower phase containing water and acetone was discarded. The remaining ethereal phase was washed five times for complete removal of the acetone. The resulting extract was transferred to a 25 mL volumetric flask, and, after adjusting the volume, the absorbance of the extract was read in a spectrophotometer (SP-22, Biospectro, Brazil) at 450 nm.
Color analysis was performed using a Konica Minolta colorimeter (CR-S, Konica Minolta, Japan). The color coordinates (CIE L*, a* and b*) were measured with D65 illuminant and 10º observation angle. Chroma (C*) and hue angle (h) were calculated according to Eq. (7) and (8).
Fourier-transform infrared spectroscopy (FTIR): the Perkin-Elmer FTIR (Spectrum Two IR, EUA) spectrophotometer connected to a full reflectance attenuator (FTIR-ATR) was used to obtain the FTIR data. The pure encapsulating materials and capsules were placed on the ZnSe crystal, and a pressure of 75 psi was applied. Scans in the range of 4000 to 650 cm−1 were acquired, with resolution of 4 cm−1. The data were analyzed by Spectrum One software.
Statistical analysis
The assays were repeated three times in sequence, and the analyses were performed in triplicate. The values of the analytical determinations were subjected to analysis of variance (ANOVA) and mean comparisons by Tukey’s test (p < 0.05) using the software Minitab 16.1.1.
Results and discussion
Complex coacervation
The produced microcapsules were multinucleated and irregular in shape, but given the suitable agitation speed, it was possible to obtain individual particles that turned into a free-flowing powder after freeze drying. After rehydration, the microcapsules could be dispersed and recovered their shape (). Devi and Kakati[Citation12] reported that non-agglomerated coacervates produced with gelatin and sodium alginate could only be obtained using certain amount of crosslinker. In the present work, the use of crosslinking agents was avoided, taking into account that most of the available crosslinkers are not allowed for use in foods, whereas others are somewhat expensive.[Citation17,Citation18] Saravanan and Rao[Citation10] observed great morphological differences between gelatin-alginate microcapsules produced by complex coacervation depending on the water solubility of core material: the particles were more spherical for the water-soluble drug metronidazole hydrochloride as core material and more irregular shaped when the core material was the drug indomethacin, which is insoluble in water. Zuanon et al.[Citation19] studied the complex coacervation between gelatin and gum Arabic to microencapsulate turmeric oleoresin and were able to produce spherical and multinucleated microcapsules at low wall material concentration (2.5%), whereas increased concentration of wall polymers (5 and 7.5%) and high proportion of core to wall materials (100%) resulted in heterogeneous and very irregular microcapsules.
Hydrodynamic conditions during coacervation
The hydrodynamic conditions during coacervation were varied by using five different stirring speeds (nominal values of 600, 950, 1300, 1650, and 2000 rpm) and two different concentrations of wall polymers (3:1 and 6:2 g/100 g of gelatin:alginate), although the proportions between the biopolymers and between the oil and wall material were kept constant, as indicated in .
The coacervation medium consists of a disperse system in which oil droplets, wall polymers, and coacervate particles are suspended in an aqueous phase. The increase in wall material concentration and the corresponding increase in oil content affected the rheological behavior of the system. The coacervate suspensions showed non-Newtonian behavior with yield stress, which was adequately described using the Herschel-Bulkley model (Equation 3). The flow behavior index, n, indicated shear thickening behavior (n > 1), and the yield stress and n increased with increasing concentration of the suspension. This behavior can be attributed to higher concentration of the dispersed phase and different size of the coacervate microparticles, as the nature of shear thickening depends on the properties of the dispersed phase and is governed by hydrodynamic interactions.[Citation20]
On the other hand, the increasing stirring speed affected the shear rate (Equation 2), and, due to the shear thickening behavior of the coacervation medium, this increase also affected the apparent viscosity.[Citation21] All these influences in the flow behavior could be taken into account through calculation of the Reynolds number (Equation 1), which increased with increasing stirring speed but decreased with increasing concentration of the coacervation medium due to the higher apparent viscosities.
Particle size
The particle size and particle size distribution are of paramount importance when the intention is the ingredient incorporation in food, since large particles may affect the textural attributes of the final product. The produced microparticles had size varying in the range of 132 to 490 μm (), which is in the range of particle sizes expected in complex coacervation.[Citation7]
Table 2. Particle size, encapsulation efficiency (%EE), brightness (L*), chroma (C*), and hue angle (h) of buriti oil microcapsules.
As illustrated in for the microcapsules produced with 3:1 g/100 g gelatin:alginate, the increase in the stirring speed led to decreasing particle size, confirmed by the decreasing values of the volume mean diameter (D[4,3]) shown in . According to Jegát and Taverdet[Citation13], the increase in the agitation speed generates an increase in the system turbulence that causes the disintegration of wall materials, forming smaller coacervates and thus smaller particles. This effect was observed in all tests, in which it is possible to observe a decrease in particle size with the increase of the stirring speed employed during coacervation.
Figure 2. Optical micrographics (bar = 200 μm) of microcapsules produced with 3:1 g/100 g gelatin:alginate, at different stirring speeds: (a) 600 rpm; (b) 950 rpm; (c) 1300 rpm; (d) 1650 rpm; (e) 2000 rpm.
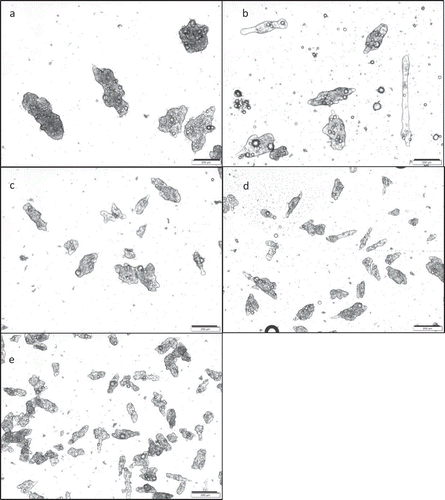
Regarding the influence of the wall material concentration, shows that there was no clear effect of this variable on particle size. Comunian et al.[Citation22] observed a decrease in particle size with increasing concentration of wall materials in complex coacervation of ascorbic acid by double emulsion in gelatin-gum Arabic. On the other hand, this dependence was not evident in the encapsulation of xylitol using the same method.[Citation23]
There was clear correlation between the Reynolds number and the particle size, independent of the concentration of wall materials in the suspension, with a decrease in the mean particle size with increasing Reynolds number (). All the coacervation assays were carried out at Reynolds number higher than 10000, which is the limit between laminar and turbulent flow in agitated vessels.[Citation24] Lemetter et al.[Citation14] observed that increasing Reynolds number led to lower particle size in complex coacervation using gelatin and gum Arabic and stated that this dimensionless number was the correct parameter to consider for scalability of the coacervation process. Our data suggest that above a certain value of Reynolds number (≈ 70000), there is a trend of stabilization in the mean particle size. This stabilization tendency was also observed by Castillo et al.[Citation25] when studying encapsulation of whole biocatalyst cells in gel beads, although their assays were carried out in laminar regimen (Re < 180).
Encapsulation efficiency
The values of encapsulation efficiency (%EE) of buriti oil by complex coacervation of gelatin:alginate were not affected by the processing conditions and were slightly above 80%. Microencapsulation by coacervation may result in high values of encapsulation efficiency, such as high as 96%.[Citation26] Qv et al.[Citation27] obtained values around 85% for the encapsulation efficiency of lutein. On the other hand, Santos et al.[Citation23] reported lower %EE for complex coacervation of a double emulsion of xylitol (varying from 31% to 72%) and attributed the low encapsulation efficiency to a probably insufficient polymer to core ratio. Zuanon et al.[Citation19] obtained %EE in the range of 49 to 73% for curcumin encapsulated in gelatin-gum Arabic coacervates and observed that increasing concentration of wall material resulted in higher encapsulation efficiency. Taking into account complex coacervation between gelatin and alginate, Saravanan and Rao[Citation10] observed that %EE depended highly on the type of core material, varying from 2.92% for a water-soluble drug (metronidazole hydrochloride) up to 96.3% for a water-insoluble drug (indomethacin).
Color analyses
According to , the color parameters of the microcapsules were influenced by the processing conditions during coacervation. Buriti oil has an orange-reddish color, and this is reflected by the values of the hue angle of the capsules (h ≈ 80), which are located in the region of intense yellowness. The chroma varying from 54 to 63 indicates that all the samples presented high color saturation. In addition, the values of C* increased and brightness (L*) decreased with increasing stirring speed, showing that smaller particles presented a more vivid yellow color, probably because of the lower thickness of the capsule wall.
Fourier-transform infrared spectroscopy
The infrared spectra of alginate, gelatin, and buriti oil are shown in , compared to the spectrum of microcapsules produced with 3:1 g/100 g gelatin:alginate at 600 rpm. The spectra of the different microcapsule formulations presented similar characteristics, with the peaks varying only in intensity, which can be attributed to the concentration of compounds used in their formulation.
In the alginate spectrum, it is possible to identify a band at wavelength value of 1609 cm−1, which is close to that found by Abruzzo et al.[Citation28] and indicates the C = O group. In the gelatin spectrum, it is possible to observe a band characteristic of the amide group, at the wavelength of 1545.0 cm−1, near that found by Yakimets et al.[Citation29] The characteristic peaks at 2928 and 2858 cm−1 of buriti oil were identified in all microcapsules, indicating their presence in the particles.
According to Saravanan and Rao[Citation10] and Santos et al.[Citation23], during complex coacervation, the carboxyl groups in the polysaccharide and the amino groups of the protein interact, forming the amide group that appears in the FTIR analysis at a wavelength around 1650 cm −1 and that can be observed in the spectrum of the microcapsules shown in . Devi and Kakati[Citation12] also observed the presence of amide groups in ascorbic acid loaded microparticles produced by complex coacervation of gelatin-alginate.
Conclusions
This work demonstrated the feasibility of producing buriti oil microcapsules through complex coacervation of gelatin-alginate with intense yellow color and high encapsulation efficiency of carotenoids. To produce microcapsules with lower particle sizes, it is essential that high Reynolds number is achieved during the coacervation step, since the hydrodynamic conditions in the coacervation medium exert great influence on the particle size of the coacervates.
References
- Albuquerque, M. L. S.; Guedes, I.; Alcantara Jr., J. R. P.; Moreira, S. G. C.; Neto, N. M. B.; Correa, D. S.; Zilio, S. C. Characterization of Buriti (Mauritia Flexuosa L.) Oil by Absorption and Emission Spectroscopies. Journal of the Brazilian Chemical Society 2005, 16, 1113–1117. 10.1590/S0103-50532005000700004
- Thies, C.;. A Survey of Microencapsulation Processes. In Microencapsulation: Methods and Industrial Applications; Benita, S.; Ed.; Marcel Dekker, Inc: New York, 1996; 1–19.
- Thies, C.;. Micoencapsulation of Flavors by Complex Coacervation. In Encapsulation and Controlled Release Technologies in Food Systems; Lakkis, J. M.; Ed.; Blackwell Publishing Ltd: Iowa, 2007; 149–170.
- Gouin, S.;. Microencapsulation: Industrial Appraisal of Existing Technologies and Trends. Food Science and Technology 2004, 15, 330–347.
- Schmitt, C.; Turgeon, S. L. Protein/Polysaccharide Complexes and Coacervates in Food Systems. Advances in Colloid and Interface Science 2011, 167, 63–70.
- Madene, A.; Jacquot, M.; Scher, J.; Desobry, S. Flavour Encapsulation and Controlled Release: A Review. International Journal of Food Science and Technology 2006, 41, 1–21.
- Xiao, Z.; Liu, W.; Zhu, G.; Zhou, R.; Niu, Y. A Review of the Preparation and Application of Flavor and Essential Oils Microcapsules Based on Complex Coacervation Technology. Journal of the Science of Food and Agriculture 2014, 94, 1482–1494.
- Pal, K.; Paulson, A. T.; Rousseau, D. Biopolymers in Controlled-Release Delivery Systems. In Handbook of Biopolymers and Biodegradable Plastics; Ebnesajjad, S.; Ed.; Elsevier: Chadds Ford, 2013; 239–362.
- Joseph, I.; Venkataram, S. Indomethacin Sustained Release from Alginate-Gelatin or Pectin-Gelatin Coacervates. International Journal of Pharmaceutics 1995, 126, 161–168.
- Saravanan, M.; Rao, K. P. Pectin–Gelatin and Alginate–Gelatin Complex Coacervation for Controlled Drug Delivery: Influence of Anionic Polysaccharides and Drugs Being Encapsulated on Physicochemical Properties of Microcapsules. Carbohydrate Polymers 2010, 80, 808–816. 10.1016/j.carbpol.2009.12.036
- Shinde, U.A; Nagarsenker, M.S. Characterization of Gelatin-Sodium Alginate Complex Coacervation System. Indian Journal of Pharmaceutical Sciences 2009, 71, 313–317. 10.4103/0250-474X.56033
- Devi, N.; Kakati, D. K. Smart Porous Microparticles Based on Gelatin/Sodium Alginate Polyelectrolyte Complex. Journal of Food Engineering 2013, 117, 193–204. 10.1016/j.jfoodeng.2013.02.018
- Jegát, C.; Taverdet, L. J. Stirring Speed Influence Study on the Microencapsulation Process and on the Drug Release from Microcapsules. Polymer Bulletin 2000, 44, 345–351.
- Lemetter, C. Y. G.; Meeuse, F. M.; Zuidam, N. J. Control of the Morphology and the Size of Complex Coacervate Microcapsules during Scale-Up. AIChE Journal 2009, 56, 1487–1496.
- Steffe, J. F.;. Rheological Methods in Food Process Engineering 2nd; Freeman Press: East Lansing, USA, 1996; 418 pp.
- Rodriguez-Amaya, D. B.; Kimura, M. General Procedure for Carotenoid Analyses. In HarvestPlus Handbook for Carotenoid Analysis; Rodriguez-Amaya, D. B., Kimura, M.; Eds.; International Food Policy Research Institute (IFPRI): Washington D.C., 2004; 8–20.
- Tavakolipour, H.; Bagheri, L.; Madadlou, A. Pomegranate Seed Oil-Loaded Particles of the Zein Cross-Linked with Citric Acid. Journal of Food Process Engineering 2015, 28, 49–56.
- Reddy, N.; Li, Y.; Yang, Y. Alkali-Catalyzed Low Temperature Wet Crosslinking of Plant Proteins Using Carboxylic Acids. Biotechnology Progress 2009, 25, 139–146.
- Zuanon, L. A. C.; Malacrida, C. R.; Telis, V. R. N. Production of Turmeric Oleoresin Microcapsules by Complex Coacervation with Gelatin–Gum Arabic. Journal of Food Process Engineering 2013, 36, 364–373.
- Sinaki, N. Y.; Martin, G.; Scanlon, M. G. Flow Behavior of Native Corn and Potato Starch Granules in Aqueous Suspensions. Food Biophysics 2016, 11, 345–353.
- Barnes, H. A. Shear‐Thickening (“Dilatancy”) in Suspensions of Nonaggregating Solid Particles Dispersed in Newtonian Liquids. Journal of Rheology 1989, 33, 329–366.
- Comunian, T. A.; Thomazini, M.; Alves, A. J. G.; De Matos Junior, F. E.; Balieiro, J. C. C.; Favaro-Trindade, C. S. Microencapsulation of Ascorbic Acid by Complex Coacervation: Protection and Controlled Release. Food Research International 2013, 52, 373–379.
- Santos, M. G.; Bozza, F. T.; Thomazini, M.; Favaro-Trindade, C. S. Microencapsulation of Xylitol by Double Emulsion Followed by Complex Coacervation. Food Chemistry 2015, 171, 32–39.
- Gabelle, J. C.; Morchain, J.; Anne-Archard, D.; Augier, F.; Liné, A. Experimental Determination of the Shear Rate in a Stirred Tank with a Non-Newtonian Fluid: Carbopol. AIChE Journal 2013, 59, 2251–2266.
- Castillo, E.; Ramírez, D.; Casas, L.; López-Munguía, A. A Two-Phase Method to Produce Gel Beads: Application in Design of a Whole Cell Β-Galactosidase Catalyst. Applied Biochemistry and Biotechnology 1992, 34, 477–486.
- Alvim, I. D.; Grosso, C. R. F. Microparticles Obtained by Complex Coacervation: Influence of the Type of Reticulation and the Drying Process on the Release of the Core Material. Ciência E Tecnologia De Alimentos 2010, 30, 1069–1076.
- Qv, X.-Y.; Zeng, Z.-P.; Jiang, J.-G. Preparation of Lutein Microencapsulation by Complex Coacervation Method and Its Physicochemical Properties and Stability. Food Hydrocolloids 2011, 25, 1596–1603.
- Abruzzo, A.; Bigucci, F.; Cerchiara, T.; Saladini, B.; Gallucci, M. C.; Cruciani, F.; Vitali, B.; Luppi, B. Chitosan/Alginate Complexes for Vaginal Delivery of Chlorhexidine Digluconate. Carbohydrate Polymers 2013, 91, 651–658.
- Yakimets, I.; Wellner, N.; Smith, A. C.; Wilson, R. H.; Farhat, I.; Mitchell, J. Mechanical Properties with Respect to Water Content of Gelatin Films in Glassy State. Polymer 2005, 46, 12577–12585.