ABSTRACT
Hertia cheirifolia is an endemic medicinal species of the Tunisian and Algerian flora. The species was found to be rich in monoterpene hydrocarbons and sesquiterpene lactones. Significant variation of essential oil composition was observed between the plant organs. Flower buds were characterized by the highest content of the drimane type (34.08%). Leaf essential oil showed the uppermost percentage of α-pinene (35.63%). Flowers and flower buds exhibited the highest amount of germacrene D (13.18 and 12.80%, respectively). The composition of leaf essential oil varied significantly depending on the harvesting time. The lowest amount of α-pinene (19.49%) and the uppermost contents of drimenin (36.16%) and the drimane type (29.14%) were observed in January (the beginning of the fruiting stage), while leaves collected in March (the full fruiting stage of plants) were marked by the most important accumulation of germacrene D (21.18%). All essential oils were found to possess antioxidant, acetylcholinesterase (AChE), and α-amylase inhibitory activities. The level of activity varied according to the oil composition. Leaf essential oils collected at the full flowering stage exhibited the important radical scavenging capacity (22.3 mg Eq trolox/g EO) and the uppermost inhibitory activities against AChE (2.91 mg Eq donepezil/g EO) and α-amylase (14.4 mg Eq acarbose/g EO). Flower buds essential oils were the most efficient inhibitors of AChE (1.86 mg Eq donepezil/g EO), while essential oils of leaves and fruits possess the highest activity against α-amylase (8.32 and 8.84 mg Eq acarbose/g EO, respectively).
Introduction
Plant secondary metabolites, naturally occurring chemical compounds, are believed to have various therapeutic values. Saponins, phenolic acids, flavonoids, terpenoids, and alkaloids are known to have antioxidant and inhibitory properties against enzymes involved in several diseases.[Citation1,Citation2] Plant phenological and development stages, plant parts, and ecological and genetic factors are shown to have a significant effect on the quality and quantity of these natural compounds.[Citation3,Citation4,Citation5,Citation6] Hence, the choice of the adequate genotype and organ and the optimal harvesting time is necessary for the production of plant active products with high medicinal value.
The Asteraceae is the largest family of flowering plants, comprising more than 1500 genera and 20000 species worldwide.[Citation7] Numerous Asteraceae species are known to have substantial pharmacological proprieties according to their important phytochemical compounds such as polyphenols (C-glycosyl flavonoids, caffeoylquinic acids, etc.), diterpenes, and sesquiterpenes lactones (eudesmane-type, germacrane-type, drimane-type, etc.).[Citation8,Citation9] Species belonging to the Asteraceae family, such as Artemisia, Achillea, Chrysanthemum, and Centaurea species, are also rich in essential oils with monoterpenes and sesquiterpenes and their oxygenated derivatives being the main terpenic compounds.[Citation9,Citation10,Citation11,Citation12] However, the qualitative and quantitative variations of essential oil composition were largely reported within and among species.[Citation10,Citation11] In Tunisia, the family includes 254 species, of which 62 have medicinal properties.[Citation13].Hertia cheirifolia (L.) Kuntze, an endemic Asteraceae species specific to Tunisian and Algerian flora, is an evergreen sub-shrub growing in large clumps with ascending and very leafy stems. Leaves are grey-green, alternate, fleshy, and oblong. Flowers are yellow. The flowering period extends from November to December. Fruits are achenes with white pappus that facilitate their dispersion.[Citation14] In Tunisia, the species grows mainly on clay postures and stony slopes. It is confined to well-drained soils with a preference for calcareous ones.
Several eremophilenolides compounds, such as 10β-hydroxyeremophilenolide, 8β-methoxy-10β-hydroxyeremophilenolide, and 8β, 10β-dihydroeremophilenolide, were isolated from the aerial parts of Hertia cheirifolia.[Citation15,Citation16] The fractionation of the chloroform extract also resulted in the isolation of one sesquiterpenoid (Bakkenolide) and two steroids (Stigmasterol and stigmasterol 3beta-glucoside).[Citation17] The few existing reports performed on the essential oil composition of Hertia cheirifolia showed that in Algeria the most abundant components were drimenin and 1, 2-Di (2-pyridinyl)-1,2-ethanediol.[Citation18] Majouli et al.[Citation19] reported that essential oils obtained from fresh vegetative aerial parts (leaves + stems), roots, and flowers of Tunisian Hertia cheirifolia were mainly composed of α-pinene and germacrene D.[Citation19] A significant variation of the essential oil composition was observed among the investigated plant parts.
The species was found to possess interesting biological properties. Leaf extracts exhibited potent antioxidant activity and interesting protective capacity against DNA damage, lipid peroxidation, protein oxidation, and inflammation.[Citation20,Citation21] Essential oils exhibited considerable antioxidant and α-glucosidase inhibitory activities.[Citation18,Citation19] The level of biological activity of the plant extract depends mainly on its chemical composition, which is influenced by several factors such as the plant genotype, the organ type, the phenological stage, and environmental conditions.
Accordingly, the aims of our study are i) to analyse the chemical composition of essential oils from leaves, flower buds, flowers, and fruits of Hertia cheirifolia from the same collection site, ii) to investigate the seasonal variability of the leaf essential oil composition during the phenological stages of the species (vegetative, flowering, and fruiting), and iii) to assess the antioxidant capacity, acetylcholinesterase (AChE), and α-amylase inhibitory activities of the essential oils. This study is essential for determining the adequate crop materials and the optimal harvesting times for a good-quality production of natural active compounds.
Materials and methods
Plant material
Aerial parts of Hertia cheirifolia were collected from 10 random plants growing wild in Sousse (35°50’N 10°35’E, lower semiarid bioclimate with a rainfall ranging between 300 and 400 mm/year). Plant material was harvested in sequence from September 2013 (vegetative stage) to March 2014 (full ripening), corresponding to distinct phenological stages of the species (). The differentiation of floral meristems and the development of flower buds and fruits were the main morphological characteristics used to define the main phenological stages of the species. The vegetative period was marked by the newly formed branches and leaves. The transition of vegetative meristems to floral ones and the initiation of flower buds until the full blooming correspond to the flowering stage. The fruiting stage was characterized by the appearance of the pappus and the beginning of achenes dispersal (). According to the stage of development, branches containing leaves associated with flower buds, flowers, or fruits were collected from the same 10 plants in one day during the first week of each month. Voucher specimens (HC-Sousse-2013-2014) were deposited at the herbarium of Tunisian National Institute of Applied Sciences and Technology (INSAT). The main climatic conditions (air temperature, precipitation, and relative humidity) throughout the seven months of collection are summarized in .
Table 1. The main climatic conditions throughout the seven months of collection.
Essential oil distillation and identification of compounds
Leaves, flower buds, flowers, and fruits were air dried at room temperature for two weeks. The dried samples (100 g) were separately ground in liquid nitrogen and subjected to hydrodistillation for 3 h using a Clevenger-type apparatus. The essential oil extractions were performed on three lots of each sample. The obtained essential oils were dried over anhydrous sodium sulphate and stored at 4°C until analysis.
The essential oil composition was determined by GC-MS (Gas chromatography-mass spectrometry) analysis. An Agilent gas chromatograph (7890 A) equipped with an HP-5MS column (30 m ×0.25 mm, 0.25-µm film thickness) and interfaced with an Agilent mass selective detector (MSD 5975C) was used. Helium was used as the carrier gas at a flow rate of 0.8 ml/min. Oven temperature program was from 40 to 250°C at 4°C/min. Transfer line temperature was 230°C; the MS quadrupole temperature was 150°C; the mass scan range was from 50 to 550 amu at 70 eV; and the scan velocity was 2.91 scans/s. Next, 1 µl of essential oil, diluted with n-hexane, was injected manually. The identification of volatile constituents was performed by comparing their linear retention indices (RIs) calculated according to a series of n-alkanes (C9–C24) with those of authentic standards available in our laboratory or from the literature, and also by comparison of their mass spectra with those of the reference compounds obtained from NIST08 and NIST mass spectral libraries.
Evaluation of antioxidant activity
The antioxidant activity of the obtained essential oil was tested using the free radical scavenging activity, the ferric reducing antioxidant power assay (FRAP), the inhibition of β-carotene bleaching test, and the metal chelating activity.
Free radical scavenging
The evaluation of the free radical scavenging activity against DPPH (2,2-diphenyl-pycrilhydrazil hydrate) was determined according to Doshi et al.[Citation22] with some modifications. Diluted essential oil (100 µl, 10 mg/ml) was mixed with 900 µl of DPPH methanolic solution (60 µM), vigorously shaken and allowed to stand at room temperature in the dark. The absorbance of the mixture was measured at 517 nm after 30 min. The radical-scavenging activities, expressed as percentage inhibition of DPPH, were calculated according to the formula: % Inhibition = 100 × [(Ac - As)/Ac], where Ac and As represent the absorbance of the control and the sample, respectively. The results were expressed as equivalent Trolox per gram of essential oil (OE) according to the linear regression equation y = 0.6642× - 0.3427; R2 = 0.998.
Ferric reducing antioxidant power assay
The ferric reducing antioxidant power was adapted from Messaoud et al.[Citation23] The FRAP reagent (900 µl) was mixed with 90 µl distilled water and 30 µl of diluted essential oils (5 mg/ml) and incubated at 37°C for 30 min. The ferric reducing power was calculated from a standard curve plotted using the Fe SO4.7H2O linear regression equation y = 1.759× + 0.0016; R2 = 0.992. The results were expressed as µmol of Fe2+/g EO.
β-Carotene bleaching test
The capacity of the essential oils to inhibit β-carotene bleaching was determined according to Messaoud et al.[Citation23] The bleaching of β-carotene was determined using the formula: % Inhibition = 100× [(Ast-Ct)/(C0-Ct)], where Ast and Ct are the absorbance measured after incubation for 120 min for the sample and the control, respectively, and C0 is the absorbance of the control measured at zero time. Results, determined by the linear regression of Butylated hydroxytoluene (y = 1.380× + 2.063; R2 = 0.988), were expressed as equivalent BHT per gram of essential oil (BHT/g EO).
Metal chelating activity
The ferrous ion chelating activities of essential oil were measured according to Yan et al.[Citation24] In brief, 300 µl of diluted samples (5 mg/ml) was added to 300 µl of FeSO4 solution (0.125 mM) and left for incubation at room temperature for 5 min. The reaction was initiated by adding 300 µl of ferrozine (0.3125 mM). The mixture was vigorously shaken and allowed to stand for 10 min. The capacity of the essential oil to chelate ion was determined using the following formula: chelating effect (%) = 100× (Ac-As/Ac), where Ac is the absorbance value of the control (consisting of methanol, iron, and ferrozine) and As is the absorbance of the tested oil. The chelating activity was calculated from a standard curve plotted using the EDTA (Ethylenediaminetetraacetic acid) linear regression equation y = 2.719× + 1.638; R2 = 0.997, and the results were expressed as equivalent EDTA per gram of essential oil.
AChE inhibition assay
The AChE activity was carried out using the colorimetric method of Eldeen et al.[Citation25] with some modifications. Briefly, 330 µl of 50 mM tris buffer (pH 8), 20 µl of diluted oil (10mg/ml) and 50 µl of AChE enzyme solution (0.28 U/ml) were incubated during 15 min at 37°C. Later, 100 µl of 0.15 mM acetylthiocholine iodide (ATCI) and 500 µl of 0.3 mM DTNB (5,5'-dithiobis-2-nitrobenzoic acid) were added and allowed to stand for 30 min at 37°C. The absorbance of the mixture was measured at 405 nm. AChE inhibitory activity (I %) was calculated as follows: I (%) = 100 × [(Ac-As)/Ac], where Ac and As are the absorbance values of the control and the tested sample, respectively. Results were expressed as equivalent Donepezil (a drug used for the treatment of mild to moderate dementia of Alzheimer’s type) according to the linear regression equation y = 8.825× + 0.0691; R2 = 0.9976.
α-Amylase inhibition assay
The α-amylase inhibitory assay of the essential oils was evaluated according to Kim et al.[Citation26] with some modifications. In brief, 100 µl of essential oil (diluted in 10% methanol in 0.02 M sodium phosphate buffer at a concentration of 10 mg/ml) was mixed with 100 µl of α-amylase solution (1U) and 200 µl of 0.02 M sodium phosphate buffer (pH 6.9). After pre-incubation at 37°C for 10 min, 180 µl of starch solution in 0.02 M sodium buffer was added. The resulting mixture was incubated at 37°C for 20 min and then stopped with 180 µl 3, 5-dinitrosalicylic acid colour reagent. After incubation in a heating bath (90°C) for 15 min and cooled down to room temperature, the reaction mixture was diluted with 600 µl of deionized water, and absorbance was measured at 540 nm. The absorbance of the blank (10% methanol instead of oil and amylase solution) and control (10% methanol instead of oil) samples was also determined. The inhibition of α-amylase was calculated using the following equation:
α-amylase inhibition (%) = 100× [(Ac-As)/Ac, where Ac is the absorbance of the solution without essential oil (10% methanol instead of oil) and As is the absorbance of the test sample. The essential oil activity was calculated from a standard curve plotted using the acarbose linear regression equation y = 3.765× - 2.978; R2 = 0.996, and the results were expressed as equivalent acarbose per gram of essential oil.
Statistical analysis
All determinations were conducted in triplicates. Variations of essential oil composition and biological activities among organs (flower buds, flowers, fruits, and the average values of leaves over all the 7 months) or between leaves collected at different months were determined by the analysis of variance (ANOVA) procedure (p < 0.05), followed by Duncan’s multiple range test. Calculations were performed using the SAS program version 9. The same analysis was carried out to assess the variation of the antioxidant, α-amylase, and anti-AChE activities. Correlations between the essential oil compounds or chemical classes and climatic parameters were determined with the PROC CORR (procedure correlation) procedure using SAS version 9. Correlations between the essential oil composition and their biological activities were also determined.
Results and discussion
Variation of essential oil composition among organs
The essential oil composition of Hertia cheirifolia leaves, flower buds, flowers, and fruits is summarized in . Twenty-three volatile components, belonging to different chemical classes (oxygenated and non-oxygenated monoterpenes and sesquiterpenes, and sesquiterpene lactones), were identified. All the investigated essential oils were found to be rich in sesquiterpene lactones (41.10–66.61%), followed by monoterpene hydrocarbons (13.61–38.61%) and sesquiterpene hydrocarbons (5.51–17.92%). The major compounds were α-pinene (12.67–35.63%), germacrene D (5.25–13.18%), drimane-type sesquiterpene lactone (13.81–34.08%), and drimenin (27.29–32.55%).
Table 2. Essential oil composition (%) of leaves, flower buds, flowers, and fruits of Hertia cheirifolia.
As shown in , the determination of the unknown compound as a drimane-type sesquiterpene lactone was mainly based on the comparison of its mass spectrum with that of drimenin (a well-known drimane-type sesquiterpene lactone derivative). In fact, the molecular ion peak (M+) of m/z 234, corresponding to C15H22O2 as the molecular formula structure, and the base peak of m/z 109 are the main characteristics of drimane-type sesquiterpene lactone components.[Citation27,Citation28]
The total essential oil composition and the occurrence of the drimane-type sesquiterpene lactone and drimenin, observed in our study, are not in line with the few previous studies reported on the species. In fact, Hertia cheirifolia growing in Algeria was characterized by its richness in drimenin (67.5%) and 1,2-Di (2-pyridinyl)-1,2-ethanediol (11.2%).[Citation18] However, α-pinene (22.1–70.4%) and germacrene D (5.9–9.5%) were determined as the main essential oil compounds of the species harvested from the centre of Tunisia (upper arid bioclimate).[Citation19]
Several factors such as organ type, growth stages, and ecological and genetic factors were found to have significant effects on the yield and composition of plant essential oils.[Citation29,Citation30] Thus, the disagreement of our results with those previously reported on H. cheirifolia could be explained by at least one of these factors. Accordingly, further studies based on the combined genetic, ecological, and chemical traits performed on H. cheirifolia populations collected from their whole distribution area may provide additional information about the qualitative and quantitative variations of the species’ essential oil profiles.
In our study, ANOVA revealed significant differences (p < 0.05) between the essential oil composition of leaves, flower buds, flowers, and fruits (). Flower buds were characterized by the lowest amount of α-pinene (12.67%) and the highest content of the drimane-type sesquiterpene lactone (34.08%). Essential oil extracted from leaves exhibited the uppermost percentage of α-pinene (35.63%) and the lowest amount of the drimane type (13.81%) and drimenin (27.9%). Flower buds and flowers exhibited the highest amounts of germacrene D (12.80 and 13.18%, respectively) and drimenin (32.53 and 32.55%, respectively). The content of minor compounds such as sabinene, myrcene, β-elemene, and β-caryophyllene also varied significantly (p < 0.05) between the analysed plant organs.
Majouli et al.[Citation19] also reported significant differences between essential oils extracted from the vegetative parts (leaves + stems), roots, and flowers of H. cheirifolia. The variation of essential oil composition among the plant organs could be attributed to the specific function of terpenic compounds in plants, including the deterrence of herbivores and the attraction of pollinators. In addition, regulatory controls operating at the organ, cellular, and enzyme levels also play an important role in controlling the pathway biosynthesis of compounds.[Citation31,Citation32]
Variation of leaf essential oil composition according to phenological stages
The chemical composition of Hertia cheirifolia essential oils extracted from leaves, with regard to the phenological stage of the plants, was also assessed (). A total of 23 compounds were identified. Significant variations of the essential oil composition according to the collection period were detected (). The vegetative stage was dominated by the highest percentage of monoterpene hydrocarbons (57.61–58.22%) and the lowest content of sesquiterpene lactones (22.41–22.42%). At the full flowering stage (December), the amount of sesquiterpene lactones increased to 45.38%. The highest percentage of these compounds (65.30%) was observed at the beginning of fruiting (January). Leaves collected at the full fruiting stage (March) were marked by the most important accumulation of sesquiterpene hydrocarbons (27.05%). The content variation of these essential oil fractions was mainly due to the change of major component percentages throughout the seven months of analysis (). In fact, the content of α-pinene, representing over 50% of the essential oil at the vegetative period, decreased markedly during the flowering and ripening periods, while the percentages of drimenin, germacrene D, and the drimane-type sesquiterpene lactone increased as the season progressed (). Furthermore, a significant variation (p < 0.05) of the essential oil composition was also observed within each phenological stage (). The lowest amount of α-pinene (19.49%) and the uppermost contents of drimenin (36.16%) and the drimane type (29.14%) were observed at the beginning of fruiting (January), while the full fruiting stage (March) was marked by the most important accumulation of germacrene D (21.18%).
Table 3. Variation of leaf essential oil composition (%) at different phenological stages of Hertia cheirifolia.
Figure 4. Variation of the percentages of α-pinene, germacrene D, drimane type, and drimenin during the three phenological stages. For each compound, bars with different letters are significantly different (p < 0.05).
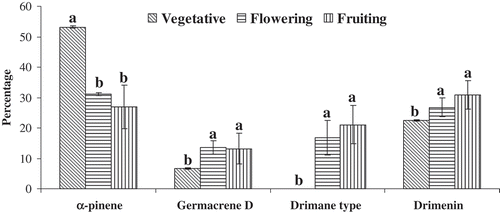
The variation of Hertia cheirifolia leaf essential oils during the seven months of analysis could be explained by the change of climatic conditions such as temperature, rainfall, humidity, day length, and sunlight.[Citation32,Citation33,Citation34] In our study, the air temperature, the precipitation, and the relative humidity levels were changed throughout the seven months. These climatic parameters were found to have a significant influence on the essential oil composition of Hertia cheirifolia. Accordingly, the percentages of monoterpene hydrocarbons, principally constituted of α-pinene, were positively correlated to the average of air temperature. However, the occurrence of sesquiterpene lactones, mainly the drimane-type compound, was negatively correlated to the air temperature. The content of drimenin was negatively linked to humidity ().
Table 4. Correlation coefficients (R) between major components and climatic parameters.
Climate changes during seasons (temperature, humidity, UV-B radiation, atmospheric CO2, etc.) have a significant impact on the synthesis of terpenes. Climatic effects could be traduced directly on the activity of several enzymes, such as isopentenyl diphosphate isomerise and prenyltransferases, that catalyze the synthesis of many terpenes, or indirectly by the downregulation or impairment of primary metabolism, and consequently the insufficient supply of photosynthetic metabolites involved in the terpene’s biosynthesis pathway.[Citation35,Citation36,Citation37,Citation38] Thus, the change of plant biochemical pathways and physiological processes with respect to harvesting time could reduce or elicit the synthesis of different terpenic compounds with important consequences for plant protection against environmental constraints.[Citation39,Citation40,Citation41]
Antioxidant, AChE, and α-amylase inhibitory activities
All the investigated essential oils were found to possess antioxidant activity as determined by the four complementary assays ( and ). However, the level of activity varied significantly between plant organs (). Essential oils extracted from leaves exhibited the highest radical scavenging activity (15.6 mg Eq trolox/g EO), while the lowest capacity (1.35 mg Eq trolox/g EO) was observed for the flower essential oils. The highest ferric reducing power (166.7 µmol Fe2+/g EO) was also observed with essential oils from leaves. The inhibition of β-carotene bleaching ranged between 10.2 (fruits) and 38.6 mg Eq BHT/g EO (flowers). The highest ferrous ion chelating activity (8.42 mg Eq EDTA/g EO) characterized the flower essential oils, while the lowest activity was recorded for essential oil of fruits (4.95 mg Eq EDTA/g EO). Hertia cheirifolia essential oils were found able to inhibit the activity of AChE and α-amylase enzymes (). Flower buds essential oils were the most efficient inhibitors of AChE (1.86 mg Eq donepezil/g EO), while essential oils extracted from leaves and fruits possessed the highest activity against α-amylase (8.32 and 8.84 mg Eq acarbose/g EO, respectively).
Table 5. Antioxidant, acetylcholinesterase, and α-amylase inhibitory activities of leaves, flower buds, flowers, and fruits.
Table 6. Antioxidant, acetylcholinesterase, and α-amylase inhibitory activities of leaf essential oil at different phenological stages.
Antioxidant, AChE, and α-amylase inhibitory activities of the essential oils extracted from leaves varied significantly along their harvesting time (). The vegetative (October) and the beginning of flowering (November) stages were marked by the highest capacity to chelate ferrous ions (8.4 and 8.3 mg Eq EDTA/g EO, respectively). Leaves collected in September (vegetative period) exhibited the important activity to inhibit the bleaching of β-carotene (51.6 mg Eq BHT/g EO). The uppermost reducing power activity (225 µmol Fe2+/g EO) was observed in November (beginning of flowering). Essential oils of leaves collected at the full flowering stage of the species (December) exhibited the important radical scavenging capacity (22.3 mg Eq trolox/g EO) and the uppermost inhibitory activities against AChE (2.91 mg Eq donepezil/g EO) and α-amylase (14.4 mg Eq acarbose/g EO).
Recently, Majouli et al.[Citation19] reported that the essential oils from the fresh flowers, vegetative part, and roots of Hertia cheirifolia possessed substantial antioxidant activities and inhibitory property against α-glucosidase. The level of each activity varied among plant organs. However, to the best of our knowledge, no published report is yet available on the inhibitory capacity of H. cheirifolia essential oils against cholinesterase and α-amylase. The inhibition of α-amylase, the enzyme involved in the digestion of carbohydrates, is considered as a strategy for the treatment of type 2 diabetes and obesity diseases.[Citation42,Citation43] Furthermore, natural AChE inhibitors from plant sources offer an attractive strategy in pharmacological application for Alzheimer’s.[Citation44,Citation45,Citation46]
The biological activities of Hertia cheirifolia essential oils, determined in our study, could be explained in part by the presence of sesquiterpenes lactones (the drimane-type sesquiterpene and drimenin) in high concentration. These compounds, mainly drimane derivatives, are known for their promising antioxidant and inhibitory activities of several enzymes involved in health disease.[Citation2,Citation47] They also possessed numerous biological activities, such as anticancer, antibacterial, antifungal, and antiviral.[Citation48,Citation49,Citation50] Monoterpenes, such as α-pinene among the major compounds of Hertia cheirifolia essential oils, were found to exhibit potent inhibitory activities against AChE and α-amylase enzymes.[Citation51,Citation52,Citation53]
In our study, the biological activities of essential oils varied significantly between organs and also during the plant development. These variations are mainly explained by the essential oil composition differences for either major or minor compounds .[Citation54,Citation55,Citation56] In fact, the correlation analysis performed between the major essential oil compounds and the analysed biological activities () showed that the contents of monoterpene hydrocarbons and α-pinene were positively correlated to the inhibition of β-carotene bleaching, contrary to the amounts of sesquiterpene lactones, drimane-type compounds, and drimenin. Furthermore, the content of drimenin was negatively correlated to the scavenging activity. Sesquiterpene lactones, mainly the drimane-type compound, were negatively correlated to the ferrous chelating activity and positively related to the anti-AChE capacity. However, the biological activities of essential oils are not directly related to the amount of the main compound, but also depended on the interactions between the major and minor ones, which produce synergetic and/or antagonistic responses. [Citation56,Citation57,Citation58] Thus, the absence of a significant correlation between the percentages of certain compounds and the level of activity does not neglect their beneficial potentialities because their biological activities have been widely reported.
Table 7. Correlation coefficients (R) between oil compounds and antioxidant, acetylcholinesterase, and α-amylase inhibitory activities.
Conclusion
This study highlighted the effect of harvesting season and plant part on the essential oil composition and biological activities of Hertia cheirifolia. The essential oils extracted from the leaves, flower buds, flowers, and fruits were characterized by their richness in monoterpene hydrocarbons and sesquiterpene lactones. Significant variations of the essential oil composition were observed both between plant organs, and between the phenological stages of the species (vegetative, flowering, and fruiting). The essential oil differences observed throughout the months of collection (from September to March) were mainly related to ecological parameters affecting the plant growth and secondary metabolites biosynthesis.
Hertia cheirifolia essential oils possessed a considerable antioxidant activity and effective inhibitory effects against enzymes related to diabetes (α-amylase) and Alzheimer’s (AChE). These properties could highlight the exploitation of the species in diverse pharmaceutical fields. However, the level of activity varied according to the plant organs and harvesting time. Leaves and flowers exhibited the highest biological activities. The level of biological activities of leaf essential oils varied significantly according to the phenological stage of plants. In fact, the important antioxidant and α-amylase and AChE inhibitory activities were determined in the vegetative and flowering periods. These results should support the choice of the adequate organ and the harvesting time of leaves for the preferential exploitation of Hertia cheirifolia according to the desired biological activities.
Complementary investigations based on the isolation and identification of individual bioactive compounds and the in vivo assessment of their antioxidant and enzymes inhibitory activities are recommended to promote the biological activities of this medicinal crop for various natural therapies.
Acknowledgments
The authors thank the Tunisian Ministry of Scientific Research and Technology and the National Institute of Applied Science and Technology for their financial support.
References
- Piero, N.M.; Kimuni, N.S.; Ngeranwa, J.J.N.; Orinda, G.O.; Njagi, J.M.; Maina, D.; Agyirifo, S.D.; Gathumbi, K.; King’e, W.S.; Njagi, E.N.M. Antidiabetic and Safety of Lantana rhodesiensis in Alloxan Induced Diabetic Rats. Journal of Developing Drugs 2015, 4, 129.
- Khan, S.; Khan, H.; Ali, F.; Ali, N.; Khan, F.U.; Khan, S.U. Antioxidant, Cholinesterase Inhibition Activities and Essential Oil Analysis of Nelumbo nucifera Seeds. Natural Product Research 2016, 30(11), 1335–1338.
- Moghaddam, M.; Pirbalouti, A.G.; Mehdizadeh, L.; Pirmoradi, M.R. Changes in Composition and Essential Oil Yield of Ocimum ciliatum at Different Phenological Stages. European Food Resesearch and Technology 2015, 240, 199–204.
- Scogings, P.F.; Hattas, D.; Skarpe, C.; Hjältén, J.; Dziba, L.; Zobolo, A.; Rooke, T. Seasonal Variations in Nutrients and Secondary Metabolites in Semi-arid Savannas Depend on Year and Species. Journal of Arid Environments 2015, 114, 54–61.
- Mastino, P.M.; Marchetti, M.; Costa, J.; Usai, M. Comparison of Essential Oils from Cistus Species Growing in Sardinia. Natural Product Research 2016, 1–9.
- Geraci, A.; Di Stefano, V.; Di Martino, E.; Schillaci, D.; Schicchi, R. Essential Oil Components of Orange Peels and Antimicrobial Activity. Natural Product Research 2016, 1–7.
- Bremer, K. Subfamily Barnadesioideae. In: Astaraceae. Cladistics and Classification. K. Bremer Ed.; Timber Press; Portland, 1994; 149–160.
- Formisano, C.; Sanna, C.; Ballero, M.; Chianese, G.; Sirignano, C.; Rigano, D.; Millán, E.; Muñoz E.; Taglialatela-Scafati, O. Anti-inflammatory Sesquiterpene Lactones from Onopordum illyricum L. (Asteraceae), an Italian Medicinal Plant. Fitoterapia 2017, 116, 61–65.
- Younsi, F.; Trimech, R.; Boulila, A.; Ezzine, O.; Dhahri, S.; Boussaid, M.; Messaoud C. Essential Oil and Phenolic Compounds of Artemisia herba-alba (Asso.): Composition, Antioxidant, Antiacetylcholinesterase and Antibacterial Activities. International Journal of Food Properties 2016, 19, 1425–1438.
- Almadiy, A.A.; Nenaah, G.E.; Al Assiuty, B.A.; Moussa, E.A.; Mira, N.M. Chemical composition and antibacterial activity of essential oils and major fractions of four Achillea species and their nanoemulsions against foodborne bacteria. LWT-Food Science and Technology 2016, 69, 529–537.
- Karamenderes, C.; Demirci, B.; Baser, K.H.C. Composition of Essential Oils of Ten Centaurea L. taxa from Turkey. Journal of essential oil research 2008, 20(4), 342–349.
- Zhang, C.; Qin, M.J.; Shu, P.; Hong, J.L.; Lü, L.; He, D.X. Chemical Variations of the Essential Oils in Flower Heads of Chrysanthemum indicum L. from China. Chemistry & Biodiversity 2010, 7(12), 2951–2962.
- Le Floc’h, E. Contribution à une étude ethnobotanique de la flore tunisienne. Imprimerie Officielle de la République Tunisienne, Tunisia.1983.
- Pottier-Alapetite, G. Flore de la Tunisie: Angiospermes Dicotylédones, Gamopétales. In: I.O.R. Tunisienne, Ed.; Tunis: Scientifiques Tunisiennes, Ministère de l’Enseignement Supe´rieur et de la Recherche Scientifique, Ministère de l’Agriculture, 1981.
- Massiot, G.; Nuzillard, J.M.; Olivier, L.; Aclinou, P.; Benkouider, A.; Khelifa, A. Eremophilenolides from Hertia cheirifolia. Phytochemistry 1990, 29, 2207–2210.
- Aclinou, P.; Georges, M. Absolute Configuration of Eremophilenolides from Hertia cheirifolia. Phytochemistry 1993, 4, 859–860.
- Ammar, S.; Edziri, H.; Mahjoub, M.A.; Chatter, R.; Bouraoui, A.; Mighri, Z. Spasmolytic and Anti-inflammatory Effects of Constituents from Hertia cheirifolia. Phytomedicine 2009, 16(12), 1156–1161.
- Zellagui, A.; Gherraf, N.; Lahouel M. Drimenin-rich Essential Oils from Hertia cheirifolia Growing in Algeria. Journal of New Technology and Materials 2012, 2, 38–40.
- Majouli, K.; Hlila, M. B.; Hamdi, A.; Flamini, G.; Jannet, H.B.; Kenani, A. Antioxidant Activity and α-Glucosidase Inhibition by Essential Oils from Hertia cheirifolia (L.). Industrial Crops and Products 2016, 82, 23–28.
- Kada, S.; Bouriche, H.; Senator, A.; Demirtaş, I.; Özen, T.; Çeken Toptanci, B.; Kizil, G.; Kizil, M. Protective Activity of Hertia cheirifolia Extracts Against DNA Damage, Lipid Peroxidation and Protein Oxidation. Pharmaceutical Biology 2017, 55(1), 330–337.
- Bouriche, H.; Kada, S.; Assaf, A. M.; Senator, A.; Gül, F.; Dimertas, I. Phytochemical Screening and Anti-inflammatory Properties of Algerian Hertia cheirifolia Methanol Extract. Pharmaceutical Biology 2016, 54(11), 2584–2590.
- Doshi, P.; Adsule, P.; Banerjee, K.; Oulkar, D. Phenolic Compounds, Antioxidant Activity and Insulinotropic Effect of Extracts Prepared from Grape (Vitis vinifera L.) by Products. Journal of Food Science and Technology 2015, 52, 181–190.
- Messaoud, C.; Laabidi, A.; Boussaid, M. Myrtus communis L. Infusions: The Effect of Infusion Time on Phytochemical Composition, Antioxidant, and Antimicrobial Activities. Journal of Food Science 2012, 77, 941–947.
- Yan, L.Y.; Teng, L.T.; Jhi, T.J. Antioxidant Properties of Guava Fruits: Comparison with Some Local Fruits. Sunway Academic Journal 2006, 3, 9–20.
- Eldeen, I.M.S.; Elgorashi, E.E.; Van Staden, J. Antibacterial, Anti-inflammatory, Anticholinesterase and Mutagenic Effects of Extracts Obtained from Some Trees Used in South African Traditional Medicine. Journal of Ethnopharmacology 2005, 102, 457–464.
- Kim, J-S.; Hyun, T.K.; Kim, M-J. The Inhibitory Effects of Ethanol Extracts from Sorghum, Foxtail Millet and Proso Millet on α-Glucosidase and α-Amylase Activities. Food Chemistry 2011, 124, 1647–1651.
- Henquet, M.G.; Prota, N.; van der Hooft, J.J.; Varbanova, M.; Hulzink, R.J.; de Vos, M.; Prins M.; de Both, M.T.J.; Franssen, M.C.R.; Bouwmeester, H.; Jongsma, M.A. Identification of a Drimenol Synthase and Drimenol Oxidase from Persicaria hydropiper, Involved in the Biosynthesis of Insect Deterrent Drimanes. The Plant Journal 2017, 90, 1052–1063.
- Prota, N.; Mumm, R.; Bouwmeester, H.J.; Jongsma, M.A. Comparison of the Chemical Composition of Three Species of Smartweed (genus Persicaria) with a Focus on Drimane Sesquiterpenoids. Phytochemistry 2014, 108, 129–136.
- Grulova, D.; De Martino, L.; Mancini, E.; Salamon, I.; De Feo, V. Seasonal Variability of the Main Components in Essential Oil of Mentha× piperita L. Journal of Agricultural and Food Chemistry 2015, 95(3), 621–627.
- Siebert, D.A.; Tenfen, A.; Yamanaka, C.N.; de Cordova, C.M.M.; Scharf, D R.; Simionatto, E.L.; Alberton, M.D. Evaluation of Seasonal Chemical Composition, Antibacterial, Antioxidant and Anticholinesterase Activity of Essential Oil from Eugenia brasiliensis Lam. Natural Product Research 2015, 29(3), 289–292.
- Verma, R.S.; Padalia, R.C.; Arya, V.; Chauhan, A. Aroma Profiles of the Curry Leaf Murraya koenigii (L.) Spreng. Chemotypes: Variability in North India During the Year. Industrial Crops and Products 2012, 36, 343–349.
- Kakaraparthi, P.S.; Srinivas, K.V.N.S.; Kumar, J.K.; Kumar, A.N.; Rajput, D.K.; Anubala, S. Changes in the Essential Oil Content and Composition of palmarosa (Cymbopogon martini) Harvested at Different Stages and Short Intervals in Two Different Seasons. Industrial Crops and Products 2015, 69, 348–354.
- Ramak, P.; Osaloo, S.K.; Sharifi, M.; Ebrahimzadeh, H.; Behmanesh, M. Biosynthesis, Regulation and Properties of Plant Monoterpenoids. Journal of Medicinal Plants Research 2014, 8(29), 983–991.
- Patel, R.P.; Singh, R.; Rao, B.R.; Singh, R.R.; Srivastava, A.; Lal, R.K. Differential Response of Genotype× Environment on Phenology, Essential Oil Yield and Quality of Natural Aroma Chemicals of Five Ocimum Species. Industrial Crops and Products 2016, 87, 210–217.
- Magel, E.; Mayrhofer S.; Müller A.; Zimmer I.; Hampp R.; Schnitzler J.P. Determination of the role of products of photosynthesis in substrate supply of isoprenoid biosynthesis in poplar leaves. Atmospheric Environment 2006, 40, 138–151.
- Arneth, A.; Schurgers, G.; Hickler, T.; Miller, P.A. Effects of Species Composition, Land Surface Cover, CO2 Concentration and Climate on Isoprene Emissions from European Forests. Journal of Plant Biology 2008, 10, 150–162.
- Schnitzler, J.P.; Louis, S.; Behnke, K.; Loivamäki, M. Poplar Volatiles-biosynthesis, Regulation and (eco) Physiology of Isoprene and Stress‐induced Isoprenoids. Journal of Plant Biology 2010, 12, 302–316.
- Estell, R.E.; Fredrickson, E.L.; James, D.K. Effect of Light Intensity and Wavelength on Concentration of Plant Secondary Metabolites in the Leaves of Flourensia cernua. Biochemical Systematics and Ecology 2016, 65, 108–114.
- Vickers, C.E.; Gershenzon, J.; Lerdau, M.T.; Loreto, F. A Unified Mechanism of Action for Volatile Isoprenoids in Plant Abiotic Stress. Nature Chemical Biology 2009, 5, 283–291.
- Sharafzadeh, S.; Zare, M. Influence of Growth Regulators on Growth and Secondary Metabolites of Some Medicinal Plants from Lamiaceae Family. Advances in Environmental Biology 2011, 5, 2296–2302.
- Akbarian, A.; Rahimmalek, M.; Sabzalian, M.R. Variation in Essential Oil Yield and Composition of Dorema aucheri Boiss, an Endemic Medicinal Plant Collected from Wild Populations in Natural Habitats. Chemistry and Biodiversity 2016, DOI: 10. 1002/cbdv. 201600160.
- Shobana, S.; Sreerama, Y.N.; Malleshi, N. G. Composition and Enzyme Inhibitory Properties of Finger Millet (Eleusine coracana L.) Seed Coat Phenolics: Mode of Inhibition of α-Glucosidase and Pancreatic Amylase. Food Chemistry 2009, 115(4), 1268–1273.
- Zengin, G.; Sarikurkcu, C.; Aktumsek, A.; Ceylan, R. Antioxidant Potential and Inhibition of Key Enzymes Linked to Alzheimer’s Diseases and Diabetes Mellitus by Monoterpene-rich Essential Oil from Sideritis galatica Bornm. Endemic to Turkey. Records Journal of Natural Products 2016, 10, 195–206.
- Bagci, E.; Aydin, E.; Mihasan, M.; Maniu, C.; Hritcu, L. Anxiolytic and Antidepressant-like Effects of Ferulago angulata Essential Oil in the Scopolamine Rat Model of Alzheimer’s disease. Flavour and Fragrance Journal 2016, 31, 70–80.
- Chen, H.W.; He, X.H.; Yuan, R.; Wei, B.J.; Chen, Z.; Dong, J.X.; Wang, J. Sesquiterpenes and a Monoterpenoid with Acetylcholinesterase (AchE) Inhibitory Activity from Valeriana officinalis var. latiofolia in Vitro and in Vivo. Fitoterapia 2016, 110, 142–149.
- Sarikurkcu, C.; Uren, M.C.; Kocak, M.S.; Cengiz, M.; Tepe, B. Chemical Composition, Antioxidant, and Enzyme Inhibitory Activities of the Essential Oils of Three Phlomis Species as Well as Their Fatty Acid Compositions. Food Science and Biotechnology 2016, 25(3), 687–693.
- Amoah, S.K.; Dalla Vecchia, M.T.; Pedrini, B.; Carnhelutti, G.L.; Gonçalves, A.E.; dos Santos, D.A.; Biavatti, M.W.; de Souza, M. Inhibitory Effect of Sesquiterpene Lactones and the Sesquiterpene Alcohol Aromadendrane-4β, 10α-diol on Memory Impairment in a Mouse Model of Alzheimer. European Journal of Pharmacology 2015, 769, 195–202.
- Derita, M.; Montenegro, I.; Garibotto, F.; Enriz, R.D.; Fritis, M.C.; Zacchino, S.A. Structural Requirements for the Antifungal Activities of Natural Drimane Sesquiterpenes and Analogues, Supported by Conformational and Electronic Studies. Molecules 2013, 18, 2029–2051.
- Paza, C.; Carcamo, G.; Silva, M.; Becerra, J.; Urrutia, H.; Sossa, K. Drimendiol, a Drimane Sesquiterpene with quorum Sensing Inhibition Activity. Natural Product Communications 2013, 8(2), 147–148.
- Montenegro, I.; Tomasoni, G.; Bosio, C.; Quiñones, N.; Madrid, A.; Carrasco, H.; Olea, A.; Martinez, R.; Cuellar, M, Villena, J. Study on the Cytotoxic Activity of Drimane Sesquiterpenes and Nordrimane Compounds Against Cancer Cell Lines. Molecules 2014, 19(11), 18993–19006.
- Miyazawa, M.; Yamafuji, C. Inhibition of Acetylcholinesterase Activity by Bicyclic Monoterpenoids. Journal of Agricultural and Food Chemistry 2005, 53, 1765–1768.
- Kivrak, İ.; Duru, M. E.; Öztürk, M.; Mercan, N.; Harmandar, M.; Topçu, G. Antioxidant, Anticholinesterase and Antimicrobial Constituents from the Essential Oil and ethanol extract of Salvia potentillifolia. Food Chemistry 2009, 116(2), 470–479.
- Ustun, O.; Senol, F.S.; Kurkcuoglu, M.; Orhan, I.E.; Kartal, M.; Baser, K.H.C. Investigation on Chemical Composition, Anticholinesterase and Antioxidant Activities of Extracts and Essential Oils of Turkish Pinus Species and Pycnogenol. Industrial Crops and Products 2012, 38, 115–123.
- Hussain, A.I.; Anwar, F.; Sherazi, S.T.H.; Przybylski, R. Chemical Composition, Antioxidant and Antimicrobial Activities of Basil (Ocimum basilicum) Essential Oils Depends on Seasonal Variations. Food Chemistry 2008, 108(3), 986–995.
- Zhang, D.Y.; Yao, X.H.; Duan, M.H.; Wei, F.Y.; Wu, G.H.; Li, L. Variation of essential Oil Content and Antioxidant Activity of Lonicera Species in Different Sites of China. Industrial Crops and Products 2015, 77, 772–779.
- Tohidi, B.; Rahimmalek, M.; Arzani, A. Essential oil composition, total phenolic, flavonoid contents, and antioxidant activity of Thymus species collected from different regions of Iran. Food Chemistry 2017, 220, 153–161.
- Aazza, S.; Lyoussi, B.; Miguel, M.G. Antioxidant and antiacetylcholinesterase activities of some commercial essential oils and their major compounds. Molecules 2011, 16, 7672–7690.
- Tak, J.H.; Jovel, E.; Isman, M.B. Comparative and Synergistic Activity of Rosmarinus officinalis L. Essential Oil Constituents Against the Larvae and an Ovarian Cell Line of the Cabbage Looper, Trichoplusia ni (Lepidoptera: Noctuidae). Pest Management Science 2016, 72(3), 474–480.