ABSTRACT
This study investigated the effects of enzymatic deglycosylation following ultrasound pretreatment on structure and immunoreactivity of soybean 7S globulin. Soybean 7S globulin was pretreated by ultrasound (40 kHz, 300 W) and enzymatically deglycosylated by peptide-N-glycosidase F (PNGase F). Changes in structure of processed soybean 7S globulin were characterized by sodium dodecyl sulphate-polyacrylamide gel electrophoresis, reversed-phase high-performance liquid chromatography, ultraviolet absorption spectrum, circular dichroism spectrum, and surface hydrophobicity analysis. Enzyme-linked immunoabsorbent assay was used to evaluate IgE-binding ability. The results showed that the glycan moieties of soybean 7S globulin were effectively removed by PNGase F, which significantly modified protein structures including the secondary and tertiary structures of 7S globulin. Individual enzymatic deglycosylation could reduce IgE-binding capacity of 7S globulin, whereas enzymatic deglycosylation following ultrasound pretreatment enhanced its IgE-binding capacity. In conclusion, soybean 7S globulin treated by single enzymatic deglycosylation can reduce potential allergenicity and may be employed in hypoallergenic food preparation.
Introduction
Soybean is widely used in the food industry for its nutritional value and desirable functional properties.[Citation1] However, soybean is among the so-called “big 8”, which together account for over 90% of all documented food allergies in the United States.[Citation2] Based on sedimentation property assessment of soybean proteins, they are classified into four groups, namely, 2S (9.4%), 7S (43%), 11S (43.6%), and 15S (4.6%) proteins.[Citation3,Citation4]
Soybean 7S globulin is not only a glycoprotein but also one of the most important allergens. β-conglycinin (140–170 kDa) is major component of 7S globulin, which consists of three subunits, namely, α (~67 kDa), α′ (~71 kDa), and β (~50 kDa) subunits. α subunit is also defined as Gly m Bd 60K.[Citation5] Moreover, β-conglycinin is a glycoprotein containing 4–5% of carbohydrate moieties, which are N-linked to polypeptide chains.[Citation6] Gly m Bd 30K (34 kDa), which consists of 258 amino acid residues, is another glycoprotein contained in disulphide linkages of 7S globulin.[Citation7] A study reported that Gly m Bd 28K (26 kDa) is a glycoprotein-bearing xylosyl residue and can bind with IgE from soybean-sensitive patients.[Citation8] Currently, 38 kinds of soybean allergens are known, and three of them, including Gly m Bd 60K, Gly m Bd 30K, and Gly m Bd 28K exist in 7S globulin; these three molecules are major allergens found in soybeans.[Citation9]
Several approaches are used to reduce or eliminate allergenicity of food proteins, and examples of these approaches include heat treatment, enzymatic hydrolysis, deglycosylation, and fermentation.[Citation10] Among these processing methods, deglycosylation is novel processing method for glycoprotein allergen. In reported food allergies, especially for plant proteins, specific antibodies to carbohydrate determinants were frequently found.[Citation11] Several N-linked glycan moieties were previously described as partial epitopes of glycoprotein allergens.[Citation12] IgE-binding epitopes are distributed not only in polypeptide fractions but also in N-glycan portion of glycoproteins. Garrido-Arandia et al.[Citation13] stated that N-glycan- and protein fractions in main kiwi allergen, namely, Act d 2, are recognized by specific IgE of kiwi-allergic patients. Benedé[Citation14] demonstrated that deglycosylated ovomucoid with lower IgE-binding forms in 80% of studied patients causes slight reduction in percentage of activated basophils. Zhang and Mine[Citation15] postulated that carbohydrate moieties of ovomucoid inhibit IgG- and IgE-binding properties. For soybean allergen, 7S globulin possesses high-mannose type structures. β(1,2)-Xylose residues remain in soybean storage glycoproteins, which are one cause of allergenic epitopes.[Citation8] Allergenicity of Gly m Bd 28K diminishes after deglycosylation.[Citation16] Nevertheless, the systematic studies have not been previously reported regarding to the structural changes of soybean 7S globulin during enzymatic deglycosylation, and the role of their glycan moieties on their allergenicity is practically unknown.
High-intensity ultrasound (20–100 kHz) is also frequently used in food processing to alter food structure and physicochemical properties via cavitation, heating, dynamic agitation, shear stresses, and turbulence.[Citation17] Arzeni[Citation18] demonstrated that sulfhydryl content was unchanged, whereas surface hydrophobicity increased significantly after ultrasound treatment of whey, soybean, and egg proteins. Ultrasonication of soybean trypsin inhibitor significantly decreased both β-turn and random coil contents and increased β-sheet structure.[Citation19] Ultrasound can induce conformational changes in food proteins and thereby influence their allergic reactivity. Li[Citation20] examined that the allergic potency of shrimp proteins were reduced under conditions of 30 kHz and 800 W for 1.5 h at 50°C. However, although increasing scientific researches focus on the changes in structure and properties of food proteins during ultrasonic treatment, there are few reports about the effect of ultrasound on soybean proteins in terms of allergenicity.
Therefore, the present paper aimed to explore feasibility of enzymatic deglycosylation coupled with ultrasound. Ultrasonic frequency of 40 kHz and power output of 300 W were selected based on our own laboratory data.[Citation21] We evaluated the effects of deglycosylation following ultrasound on the structure and potential allergenicity of soybean 7S globulin to formulate an effective method for removing glycan moieties and reducing potential allergenicity of soybean 7S globulin.
Materials and methods
Materials
Soybean cultivar ‘Dong nong 42’ was kindly provided by Key Laboratory of Soybean Biology, Northeast Agricultural Univ., China. Peptide-N-glycosidase F (PNGase F) was purchased from New England Biolabs. Schiff reagent was supplied by Beijing Leagene Biotechnology Co., Ltd. Bovine serum albumin (BSA), 8-Anilino-1-naphthalenesulfonic acid (ANS), trifluoroacetic acid (TFA), o-phenylenediamine dihydrochloride (OPD), biotin-labelled goat anti-human IgE and streptavidin-conjugated horseradish peroxidase were from Sigma–Aldrich (St. Louis, MO., USA). All chemicals used in this research were of analytical grade. Sera from seven soybean-allergic patients were collected from the First Affiliated Hospital of Guangxi Medical University (Nanning, China), and the ethical requirements were fulfilled.
Extraction of 7S globulin
Soybean 7S globulin was isolated according to the method of Nagano.[Citation22] Proteins were dialyzed and stored in −20°C. Protein determination was performed by Bio-Rad Protein Assay[Citation23] using BSA as standard.
Enzymatic deglycosylation of soybean 7S globulin after ultrasound pretreatment
Two milligrams per millilitre of soybean 7S globulin in 50 mM Tris-HCl buffer (pH 8.0) was ultrasonicated with ultrasound processor (NingBo Scientz Biotechnology Co. Ltd., NingBo, China) (40 kHz, 300 W) for 0, 80, and 100 min, successively. Typical reaction conditions of deglycosylation were established per the manufacturer’s procedure. A 10 µL total reaction volume was prepared by mixing 20 µg glycoprotein, 1 µL 10× glycoprotein denaturing buffer, and distilled H2O (when necessary). Glycoproteins were denatured via preheating at 100°C for 10 min. A total reaction volume of 20 µL was concocted by adding 2 μL 10× G7 Reaction Buffer, 2 µL 10% NP-40, 1–5 µL PNGase F and distilled H2O. Control group without PNGase F was also included in the study. Mixed system was incubated at 37°C for 4.5 h. After deglycosylation, PNGase F was inactivated by heating at 100°C for 8 min.
Sodium dodecyl sulphate-polyacrylamide gel electrophoresis (SDS-PAGE)
SDS-PAGE was performed according to Laemmli[Citation24] with some modifications. Samples were resuspended in reducing sample buffer and heated for 5 min in boiling water bath. Aliquots of 15 μL prepared samples were loaded into gels and run with a minigel apparatus (Bio-Rad Laboratories, Hertfordshire, UK) with 12% separating gel and 4% stacking gel. The gels were stained with Coomassie Brilliant Blue R-250 and periodic acid Schiff (PAS) reagent, respectively.[Citation25] Coomassie Brilliant Blue R-250 was used to stain polypeptide chains, whereas PAS reagent was involved in attaching carbohydrate moieties to 7S globulin.
Reversed-phase high-performance liquid chromatography (RP-HPLC)
Native and processed 7S samples were placed (80 μL, 0.5 mg/mL) onto LC-20AT HPLC column (Inertsil WP300 C8, 5 μm particle size, 250 × 4.6 mm, Shimadzu). Binary solvent system consisted of solvent A, which contained 0.1% (v/v) TFA in Milli-Q water (Milli-Q-Plus, Millipore), and solvent B, which contained 0.1% (v/v) TFA in acetonitrile at flow rate of 1.0 mL/min. Prior to sample injection, column was equilibrated with 20% acetonitrile for 20 min. For solvent B, multistep linear gradient elution was continuous from 20% at 0 min to 45% at 95 min, maintained at 45% for 110 min, and then returned to initial conditions for 0%. ultraviolet (UV) detection was performed at 210 nm.
Circular dichroism (CD)
CD studies of native and processed 7S globulins were performed on JASCO J810 spectropolarimeter (JASCO Corporation, Tokyo, Japan) using 0.1 cm path length quartz cell at 25°C. CD spectrum was collected at protein concentration of 0.2 mg/mL. Data points were recorded with scan range of 190–250 nm, scan speed of 50 nm/min, spectral bandwidth of 1.0 nm, sensitivity of 20 mdeg, and response time of 0.25 s. Results for each sample were averaged, and mean residue ellipticity (θ) was expressed as deg·cm2/dmol. Secondary structure contents were calculated using JASCO secondary structure software.
UV absorption spectrum
UV absorption spectrum was recorded using a UV-2450 spectrophotometer (Shimadzu Corporation, Japan) and scanned from 250 to 350 nm. Protein concentration was 0.1 mg/mL.
ANS fluorescence intensity
Surface hydrophobicities of native and processed 7S globulin were evaluated using ANS as fluorescence probes according to Kato et al.[Citation26] An aliquot of 50 μL 8.0 mM ANS solution was added to 5.0 mL of each of protein sample solution (protein concentration was 0.2 mg/mL). Fluorescence intensity was measured with fluorescence spectrometer (F-4500, HITACHI, Japan) with 2.5 nm slit width and 1 cm path length. Excitation wavelength was 390 nm, and emission wavelength was 400–650 nm at scanning speed of 1200 nm/min.
Field emission scanning electron microscopy (FESEM)
FESEM (JSM-6701F, JEOL DATUM Ltd., Tokyo, Japan) was used for microstructure analysis of soybean 7S globulins.[Citation27] Samples were diluted to 0.2 mg/mL. After dilution, 1 mL sample was dropped and coated uniformly on aluminium foil paper and dried overnight at 60°C. Samples were preserved in culture dish filled with desiccant silica gel. It needs to be sprayed before examination under FESEM.
IgE-binding evaluation
By competitive inhibition of enzyme-linked immunoabsorbent assay (ELISA), we evaluated IgE-binding of native and deglycosylated soybean 7S globulins. A 96-well ELISA microplate was coated with native 7S globulin (20 μg/mL) in coating buffer (pH 9.6, 0.015 M Na2CO3, 0.035 M NaHCO3) and then incubated overnight at 4°C. Plates were washed with a phosphate-buffered saline solution containing 0.05% Tween-20 (PBST), and residual-free binding sites were blocked with 3% gelatin in PBS for 1 h at 37°C. A new microplate was also blocked with 3% gelatin in PBS for 1 h at 37°C. After washing thrice with PBST, 55 µL of serum pool of soybean-allergic patients (diluted 1:10) was incubated with 55 μL of native or processed sample for 1 h at 37°C. Then, 100 μL of mixture was removed from coated plate and incubated for 2 h at 37°C. Wells were washed again, and 100 µL of biotin-labelled goat anti-human IgE (diluted 1:5000) was also added, and mixture was incubated for 1 h at 37°C. After washing, 100 μL streptavidin-conjugated horseradish peroxidase was added before incubation for 1 h at 37°C. Reaction was initiated with 100 μL substrate solution of OPD for 15 min at 37°C, stopped by adding 50 µL 2 M H2SO4 and OD at 490 nm was measured by ELISA reader (Bio-Rad Laboratories, Hercules, CA, USA). Percentage of inhibition was expressed as 100–100(B1 − B0)/(B2 − B0), where B0 is average absorbance of blank control wells without primary antibodies; B1 is average absorbance of wells with processed proteins; B2 is average absorbance of positive control wells without competitive proteins (maximum absorbance). Supplementary table lists specific IgE levels of seven soybean patients’ sera, and IgE levels were quantified by the CAP-fluorescent enzyme immunoassay system (Phadia, Uppsala, Sweden). There were two patients had eczema, and four had urticaria.
Statistical analysis
All experiments were conducted in triplicate. Data were represented as mean and standard deviation, which were analysed with statistical significance using ANOVA (SPSS for Windows, version 13.0, Chicago, IL, USA) and Fisher’s least significant difference (p < 0.05) test.
Results and discussion
SDS-PAGE analysis
presents typical SDS-PAGE profile for soybean 7S globulin treated with ultrasound following enzymatic deglycosylation. , stained by Coomassie Brilliant blue, shows a diffuse group of 7S globulin protein bands with molecular weight ranging from 17 to 95 kDa and obviously indicates β-conglycinin and Gly m Bd 30K (P34) bands. Bands with similar molecular mass were exhibited, but slight reduction in molecular weight of polypeptide of β-conglycinin and higher electrophoretic mobility were observed in lane of 7S globulin treated with enzymatic deglycosylation compared with the native 7S globulin. It can be deduced that the oligosaccharides linked to polypeptide chains of 7S globulin was removed by PNGase F. Similar result was reported by Amigo-Benavent et al[Citation28], who observed slightly lower molecular weight and higher electrophoretic mobility of deglycosylated β-conglycinin. However, no significant changes in protein bands were observed compared with lanes 2–4 of 7S globulin treated with enzymatic deglycosylation following ultrasound pretreatment. Results suggested that ultrasound has no significant effect on primary structure of soybean 7S globulins.
Figure 1. SDS-PAGE profile for soybean 7S globulin treated with ultrasound following the deglycosylation. Lane 1: Native; Lane 2: Deglycosylation; Lane 3: Ultrasound 80 min assisted deglycosylation; Lane 4: Ultrasound 100 min assisted deglycosylation. (A) Coomassie Brilliant blue staining and (B) Schiff reagent staining.
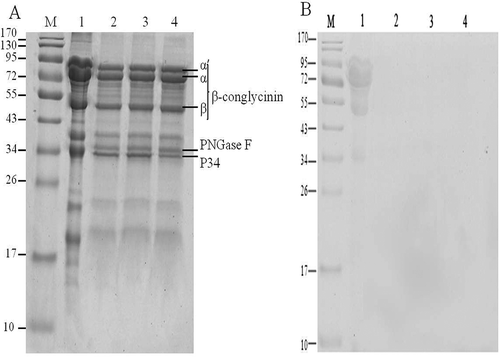
Schiff reagent combined with periodic acid is often used for glycoprotein detection in gel electrophoresis. Periodic acid is strong oxidizing agent and oxidizes 1,2-propylene glycol in sugars to form dialdehydes, which combine with Schiff reagent molecules to form insoluble magenta compound, resulting in clearly stained glycoprotein bands in electrophoresis lane. As shown in , protein bands were clearly visualized in lane of native soybean 7S globulin stained by Schiff reagent, whereas no bands were detected in lane of deglycosylated 7S fractions (lanes 2, 3, and 4). It further confirmed that the glycan moieties of soybean 7S globulin were effectively removed by PNGase F after enzymatic deglycosylation.
RP-HPLC analysis
presents RP-HPLC profiles of native and processed soybean 7S globulins. Compared with the native forms, the new peak of PNGase F at 87 min was observed in elution profiles of deglycosylated forms. The retention times of processed forms have been changed, which showed that enzymatic deglycosylation prolonged elution period of 7S globulin, whereas elution peaks of 7S globulin treated by enzymatic deglycosylation following ultrasound were similar to that of the single enzymatic glycosylation, implying that ultrasound pretreatment did not have a significant impact on 7S globulin primary structure. It is well known that the compounds were separated based on their hydrophobic characteristics in RP-HPLC. Results showed that hydrophobicity of soybean 7S globulin increased after enzymatic deglycosylation. Hydrophobic changes may be due to the variation in protein molecule and structure because of the removal of the carbohydrate moieties under the action of deglycosylation enzyme.
Far-UV CD spectra
shows the effects of enzymatic deglycosylation following ultrasound pretreatment on secondary structures of 7S globulins. As shown in ), strong positive peak near 190 nm and negative peak at approximately 210 nm were observed in native soybean 7S globulins showing that β-sheet is probably the major secondary structure of native soybean 7S globulins.[Citation29] As presented in ), significant changes were observed in percentage of secondary structure of processed 7S proteins compared with native 7S globulin. As indicated in the same figure, α-helix content and random coils decreased, whereas β-sheet content and β-turn increased significantly after 7S globulin deglycosylation. However, no difference was observed in secondary structure of 7S globulins in other deglycosylated groups. Results revealed that secondary structures of 7S globulin were altered when carbohydrate chains were cleaved by PNGase F treatment, but ultrasonic pretreatment did not significantly affect secondary structure of deglycosylated 7S globulins. Our results were consistent with the changes reported by Shivanjali, who discovered that α-helix content of deglycosylated carboxypeptidase is lower than that of the native form.[Citation30] Hwang[Citation31] also noted that secondary structures of deglycosylated ovotransferrin were fairly different from native forms, showing that α-helix and random coil transformed into β-sheets, and this finding completely coincides with our results.
UV absorption spectrum and surface hydrophobicity analysis
As shown by UV absorption spectra, at approximately 280 nm, characteristic absorption peak appeared (), and this result can be attributed to UV absorption of tryptophan and tyrosine residues, also to some extent on phenylalanine, histidine, and cysteine.[Citation32] The processed 7S globulins exhibited significant change in UV absorption compared with native forms. Enzymatic deglycosylation could decrease absorption intensity. UV absorption intensity of native 7S globulin was highest at 270 nm, followed by those of singly deglycosylated and enzymatically deglycosylated after ultrasound pretreatment 7S globulins. Difference in absorption intensity was associated with presence of chromophoric groups which indicates protein conformation change. Singly deglycosylated 7S globulin exhibited similar maximum absorption peak position with that of native form, but the UV absorption peak position in sample treated with ultrasound pretreatment followed by enzymatic deglycosylation was slightly changed, and the blue shift of 3 nm was observed. The phenomenon of spectral blue shift could be due to the unfolding of 7S globulin molecules during the ultrasound pretreatment. Data on CD spectrum clearly supported UV analysis results.
Figure 4. UV spectrum analysis (A) and surface hydrophobicity (B) of soybean 7S globulin treated with ultrasound following the deglycosylation.
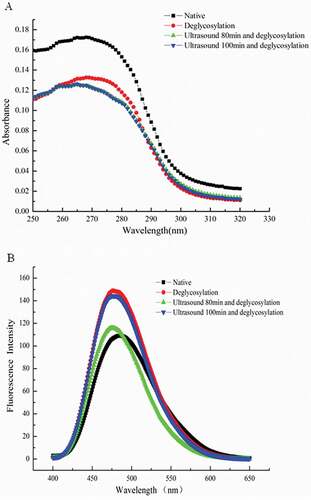
ANS is widely used as fluorescent probe to measure surface hydrophobicity of proteins. Hydrophobic interactions play important roles in stabilizing tertiary protein structures. displays surface hydrophobicity of native and processed 7S globulins. Compared with native form, significant blue shift in fluorescence spectra and increase in fluorescence intensity were observed after deglycosylation of 7S globulin, implying increased surface hydrophobicity.[Citation33] This result was consistent with analysis results of RP-HPLC.
Our results agreed with those of Gopal[Citation34], who discovered higher excitation intensities of deglycosylated porcine pancreatic α-amylase (PPA) and its isoforms (PPA-I and PPA-II) than those of native proteins. However, slight red shift in emission spectra was observed, and this result indicated changes in tertiary structure of samples.
Protein microstructure analysis
The effect of enzymatic deglycosylation following ultrasound pretreatment on surface characteristics of soybean 7S globulin was shown in (20,000×). Disordered structures with irregular fragments were manifested on surface morphology of native 7S globulin, whereas numerous small particles, namely, oligosaccharides attached to glycoproteins, were observed on glycoprotein surface after enzymatic deglycosylation (upper right corner of small images in ). Through comparison of , , and , significant difference in effect of deglycosylation on soybean 7S globulin was observed. For 7S protein of deglycosylation following ultrasound 80, 100 min, there is lower deglycosylation. The phenomenon may be explained that ultrasonic can destroy the dimensional structure of protein, which may influence the sites of soybean 7S globulin during deglycosylation.
IgE-binding analysis
The potential allergenicity of food proteins has been reduced by food processing technologies to modify allergens from food products. Soybean 7S globulin is a glycoprotein. Therefore, enzymatic deglycosylation should be a method to modify IgE reactivity of the 7S globulin. Results of potential allergenicity of native or processed 7S globulins were estimated through competitive inhibition of ELISA and are shown in . It shows that singly deglycosylated 7S globulin exhibited a decrease in IgE-binding compared with the native form. Deglycosylation possibly caused disappearance of allergenic epitopes located in oligosaccharides. Previous research reported that constitute approximately 4–5% of soybean 7S globulins.[Citation35] Given that oligosaccharide content of 7S globulin is relatively low, no significant reduction in IgE-binding was observed. Regarding to the potential allergenicity of soybean protein modified by ultrasonication. Yang[Citation21] et al. in our early studies found that protein from soybean sprouts treated with ultrasonication at 300 W yielded the highest decrease in IgE-binding potency. Thus, the experiment that enzymatic deglycosylation following ultrasound pretreatment (300 W) was conducted in this study. However, it showed that deglycosylation following ultrasound pretreatment enhanced the IgE-binding of soybean 7S globulin, which was attributed to protein structural changes induced by extreme ultrasound. Single ultrasound treatment could damage disulphide bonds and cause loosening and unfolding of polypeptides chain in glycoproteins under certain conditions, which echoed the changes in UV absorption and surface hydrophobicity measurements. The loose soybean 7S globulins might expose some allergenic epitopes on surface of molecules even after enzymatic deglycosylation. Such difference could be explained by IgE-binding epitope characterization of soybean 7S globulin and its deglycosylated form in our future work. Moreover, further investigations, such as animal or clinical studies, are still required to confirm whether single deglycosylation-treated 7S globulins can significantly reduce allergic symptoms related to consumption of their regular counterparts.
Conclusion
In conclusion, this study provided important insights into relationship between protein structure and IgE-binding of deglycosylated soybean 7S globulins. Enzymatic deglycosylation significantly damaged structural integrity of proteins, including their secondary and tertiary structure, and deglycosylation following ultrasound treatment could destroy disulphide bonds and cause loosening and unfolding of polypeptides chain in 7S globulins. In vitro IgE-binding ability showed that single deglycosylation decreased IgE-binding of soybean 7S globulin, and deglycosylation following ultrasound treatment significantly increased IgE-binding of 7S globulins. Our results indicated that single deglycosylation can be considered as effective strategy for reducing potential allergenicity of soybean 7S globulins.
Supplemental Material
Download ()Supplemental data
Supplemental data for this article can be access on the publisher’s website.
Additional information
Funding
References
- Wang, X.-S.; Tang, C.-H.; Li, B.-S.; Yang, X.-Q.; Li, L.; Ma, C.-Y. Effects of High-Pressure Treatment on Some Physicochemical and Functional Properties of Soy Protein Isolates. Food Hydrocolloids 2008, 22(4), 560–567. DOI: 10.1016/j.foodhyd.2007.01.027.bk_AQCmts3b
- Meinlschmidt, P.; Ueberham, E.; Lehmann, J.; Schweiggert-Weisz, U.; Eisner, P. Immunoreactivity, Sensory and Physicochemical Properties of Fermented Soy Protein Isolate. Food chemistry 2016, 205, 229–238. DOI: 10.1016/j.foodchem.2016.03.016.
- L’hocine, L.; Boye, J. I. Allergenicity of Soybean: New Developments in Identification of Allergenic Proteins, Cross-Reactivities and Hypoallergenization Technologies. Critical reviews in food science and nutrition 2007, 47(2), 127–143. DOI: 10.1080/10408390600626487.
- Nishinari, K.; Fang, Y.; Guo, S.; Phillips, G. O. Soy Proteins: A Review on Composition, Aggregation and Emulsification. Food Hydrocolloids 2014, 39, 301–318. DOI: 10.1016/j.foodhyd.2014.01.013.
- Verma, A. K.; Kumar, S.; Das, M.; Dwivedi, P. D. A Comprehensive Review of Legume Allergy. Clin Rev Allergy Immunol 2013, 45(1), 30–46. DOI: 10.1007/s12016-012-8310-6.
- Maruyama, N.; Katsube, T.; Wada, Y.; Oh, M. H.; Barba De La Rosa, A. P.; Okuda, E.; Utsumi, S. The Roles of the N-Linked Glycans and Extension Regions of Soybean β‐conglycinin in Folding, Assembly and Structural Features. European journal of biochemistry 1998, 258(2), 854–862. DOI: 10.1046/j.1432-1327.1998.2580854.x.
- Wilson, S.; Martinez‐Villaluenga, C.; De Mejia, E. G. Purification, Thermal Stability, and Antigenicity of the Immunodominant Soybean Allergen P34 in Soy Cultivars, Ingredients, and Products. Journal of food science 2008, 73(6), T106–T114. DOI: 10.1111/jfds.2008.73.issue-6.
- Kimura, Y.; Ohno, A.; Takagi, S. Structural Analysis of N-Glycans of Storage Glycoproteins in Soybean (Glycine max L) Seed. Bioscience, Biotechnology, and Biochemistry 1997, 61(11), 1866–1871. DOI: 10.1271/bbb.61.1866.
- Wu, Y. M.; Guan, R. X.; Liu, Z. X.; Li, R. Z.; Chang, R. Z.; Qiu, L. J. Synthesis and Degradation of the Major Allergens in Developing and Germinating Soybean Seed. Journal of integrative plant biology 2012, 54(1), 4–14. DOI: 10.1111/j.1744-7909.2011.01092.x.
- Verhoeckx, K. C.; Vissers, Y. M.; Baumert, J. L.; Faludi, R.; Feys, M.; Flanagan, S.; Herouet-Guicheney, C.; Holzhauser, T.; Shimojo, R.; Van Der Bolt, N.; et al. Food Processing and Allergenicity. Food Chem Toxicol 2015, 80, 223–240. DOI: 10.1016/j.fct.2015.03.005.
- Altmann, F. The Role of Protein Glycosylation in Allergy. International archives of allergy and immunology 2006, 142(2), 99–115. DOI: 10.1159/000096114.
- Van Ree, R. Carbohydrate Epitopes and Their Relevance for the Diagnosis and Treatment of Allergic Diseases. International archives of allergy and immunology 2002, 129(3), 189–197. DOI: 10.1159/000066770.
- Garrido‐Arandia, M.; Murua‐García, A.; Palacin, A.; Tordesillas, L.; Gómez‐Casado, C.; Blanca‐Lopez, N.; Ramos, T.; Canto, G.; Blanco, C.; Cuesta‐Herranz, J. The Role of N-Glycosylation in Kiwi Allergy. Food science & nutrition 2014, 2(3), 260–271. DOI: 10.1002/fsn3.99.
- Benedé, S.; López-Fandiño, R.; Reche, M.; Molina, E.; López-Expósito, I. Influence of the Carbohydrate Moieties on the Immunoreactivity and Digestibility of the Egg Allergen Ovomucoid. PLoS ONE 2013, 8(11), e80810. DOI: 10.1371/journal.pone.0080810.
- Zhang, J. W.; Mine, Y. Characterization of IgE and IgG Epitopes on Ovomucoid Using Egg-White-Allergic Patients’ Sera. Biochemical and biophysical research communications 1998, 253(1), 124–127. DOI: 10.1006/bbrc.1998.9761.
- Tsuji, H.; Bando, N.; Hiemori, M.; Yamanishi, R.; Kimoto, M.; Nishikawa, K.; Ogawa, T. Purification and Characterization of Soybean Allergen Gly M Bd 28K. Bioscience, Biotechnology, and Biochemistry 1997, 61(6), 942–947. DOI: 10.1271/bbb.61.942.
- Shriver, S. K.; Yang, W. W. Thermal and Nonthermal Methods for Food Allergen Control. Food Engineering Reviews 2011, 3(1), 26–43. DOI: 10.1007/s12393-011-9033-9.
- Arzeni, C.; Martinez, K.; Zema, P.; Arias, A.; Pérez, O. E.; Pilosof, A. M. R. Comparative Study of High Intensity Ultrasound Effects on Food Proteins Functionality. Journal of Food Engineering 2012, 108(3), 463–472. DOI: 10.1016/j.jfoodeng.2011.08.018.
- Huang, H.; Kwok, K. C.; Liang, H. H. Inhibitory Activity and Conformation Changes of Soybean Trypsin Inhibitors Induced by Ultrasound. Ultrasonics Sonochemistry 2008, 15(5), 724–730. DOI: 10.1016/j.ultsonch.2007.10.007.
- Zhenxing, L.; Caolimin, L.; Jamil, K. Reduction of Allergenic Properties of Shrimp (Penaeus vannamei) Allergens by High Intensity Ultrasound. European Food Research and Technology 2006, 223(5), 639–644. DOI: 10.1007/s00217-005-0246-0.
- Yang, H.; Gao, J.; Yang, A.; Chen, H. The Ultrasound-Treated Soybean Seeds Improve Edibility and Nutritional Quality of Soybean Sprouts. Food Research International 2015, 77, 704–710. DOI: 10.1016/j.foodres.2015.01.011.
- Nagano, T.; Hirotsuka, M.; Mori, H.; Kohyama, K.; Nishinari, K. Dynamic Viscoelastic Study on the Gelation of 7 S Globulin from Soybeans. Journal of Agricultural and food chemistry 1992, 40(6), 941–944. DOI: 10.1021/jf00018a004.
- Bradford, M. M. A Rapid and Sensitive Method for the Quantitation of Microgram Quantities of Protein Utilizing the Principle of Protein-Dye Binding. Analytical Biochemistry 1976, 72(1–2), 248–254. DOI: 10.1016/0003-2697(76)90527-3.
- Laemmli, U. K. Cleavage of Structural Proteins during the Assembly of the Head of Bacteriophage T4. Nature 1970, 227(5259), 680–685. DOI: 10.1038/227680a0.
- Kapitany, R. A.; Zebrowski, E. A High Resolution PAS Stain for Polyacrylamide Gel Electrophoresis. Analytical biochemistry 1973, 56(2), 361–369. DOI: 10.1016/0003-2697(73)90202-9.
- Kato, A.; Nakai, S. Hydrophobicity Determined by a Fluorescence Probe Method and Its Correlation with Surface Properties of Proteins. Biochimica et Biophysica Acta (BBA) - Protein Structure 1980, 624(1), 13–20. DOI: 10.1016/0005-2795(80)90220-2.
- Chen, Y.; Ono, T. Protein Particle and Soluble Protein Structure in Prepared Soymilk. Food Hydrocolloids 2014, 39, 120–126. DOI: 10.1016/j.foodhyd.2014.01.005.
- Amigo-Benavent, M.; Athanasopoulos, V. I.; Ferranti, P.; Villamiel, M.; Del Castillo, M. D. Carbohydrate Moieties on the in Vitro Immunoreactivity of Soy Beta-Conglycinin. Food Research International 2009, 42(7), 819–825. DOI: 10.1016/j.foodres.2009.03.003.
- Tang, C.-H.; Sun, X. Structure–Physicochemical Function Relationships of 7S Globulins (Vicilins) from Red Bean (Phaseolus angularis) with Different Polypeptide Constituents. Food Hydrocolloids 2011, 25(3), 536–544. DOI: 10.1016/j.foodhyd.2010.08.009.
- Joshi, S.; Katiyar, S.; Lennarz, W. J. Misfolding of Glycoproteins Is a Prerequisite for Peptide: N-Glycanase Mediated Deglycosylation. FEBS Lett 2005, 579(3), 823–826. DOI: 10.1016/j.febslet.2004.12.060.
- Hwang, H. S.; Kim, B. S.; Park, H.; Park, H. Y.; Choi, H. D.; Kim, H. H. Type and Branched Pattern of N-Glycans and Their Structural Effect on the Chicken Egg Allergen Ovotransferrin: A Comparison with Ovomucoid. Glycoconj 2014, 31(1), 41–50. DOI: 10.1007/s10719-013-9498-2.
- Jiang, J.; Chen, J.; Xiong, Y. L. Structural and Emulsifying Properties of Soy Protein Isolate Subjected to Acid and Alkaline pH-shifting Processes. J Agric Food Chem 2009, 57(16), 7576–7583. DOI: 10.1021/jf901585n.
- Stanciuc, N.; Banu, I.; Turturica, M.; Aprodu, I. pH and Heat Induced Structural Changes of Chicken Ovalbumin in Relation with Antigenic Properties. Int J Biol Macromol 2016, 93(Pt A), 572–581. DOI: 10.1016/j.ijbiomac.2016.09.025.
- Gopal, B. A.; Singh, S. A.; Muralikrishna, G. Porcine Pancreatic Alpha Amylase and Its isoforms – Effect of Deglycosylation by peptide-N-glycosidase F. F. International Journal of Biological Macromolecules 2008, 43(2), 100–105. DOI: 10.1016/j.ijbiomac.2008.03.008.
- Yamauchi, F.; Kawase, M.; Kanbe, M.; Shibazaki, K. Separation of the β-Aspartamido-Carbohydrate Fractions from Soybean 7S Protein: Protein-Carbohydrate Linkage. Agricultural and Biological Chemistry 2014, 39(4), 873–878.