ABSTRACT
Bioactive fraction of brown algae Sargassum wightii (SWE) was obtained using silica column chromatography and preparative Thin Layer Chromatography (TLC). FT-IR and LC–mass spectrometry ESI analysis revealed presence of various phlorotannins in the SWE. The IC50 value of SWE was found to be 59.91, 51.04, and 55.21 μg/ml for scavenging of 2,2-diphenyl-1-picrylhydrazyl, 2,2-azino-bis-(3-ethylbenzothiazoline-6-sulfonic acid), and Ferric Reducing Antioxidant Power assay, respectively. SWE inhibited angiotensin-I-converting enzyme (ACE) in mixed-type manner with IC50 value of 56.96 µg/ml and Ki value of 45 µg/ml. The dual function such as antioxidant and ACE inhibition of SWE warrants further study to understand the antihypertensive potential in vivo.
Introduction
Hypertension (raised blood pressure) is the leading cause of mortality among noncommunicable diseases with estimation of 9.4 million deaths annually worldwide.[Citation1] Hypertension is remediable risk factor, if untreated can lead to stroke, myocardial infarction, cardiac failure, dementia, renal failure, and blindness. Angiotensin-I-converting enzyme (ACE) plays an important role in the regulation of blood pressure and is widely related to the treatment of hypertension. ACE is a bivalent dipeptidyl carboxyl metallopeptidase predominantly located in the vascular endothelial cells of the lung. ACE plays an integral part in renin–angiotensin system. Renin produced from the juxtaglomerular apparatus of the kidney catalytically cleaves angiotensinogen to produce the inactive decapeptide angiotensin I. ACE converts the angiotensin I into octapeptide vasoconstrictor called angiotensin II. In addition, ACE catalyzes the bradykinin (a hypotensive peptide) to inactive components. Angiotensin II is also involved in the release of a sodium-retaining steroid called aldosterone from the adrenal cortex, which in turn results in increased blood pressure.
Imbalance between reactive oxygen species (ROS) production to antioxidant status results in oxidative stress. Debate prevails regarding whether oxidative stress is a cause or a result of hypertension; however, it is often found that hypertension is associated with increased vascular oxidative stress.[Citation2] Nitric oxide (NO) released by endothelial cells plays a major role in vascular relaxation. NO is rapidly degraded by the ROS. Experimental model of hypertension in animals and human hypertension is shown to be associated with decreased NO availability, impaired antioxidants, and increased oxidative stress.[Citation3–Citation5] Redox-dependent signaling cascades activation and Nicotinamide adenine dinucleotide phosphate (NADPH) oxidase-driven generation of ROS are involved in the role of angiotensin-II-induced hypertension.[Citation6,Citation7] Also, angiotensin II stimulates the production of ROS and hydrogen peroxide in the polymorphonuclear leucocytes, which inactivates the NO as well as other vascular relaxing factor such as prostacyclin.[Citation8]
All these facts congregate the importance of synergistic strategy such as inhibition of ACE as well as curbing oxidative stress by the same lead molecule as important therapeutic approach to treat hypertension. ACE inhibitors, such as captopril, enalapril, alcacepril, and lisinopril, are currently used in the treatment of hypertension in humans. However, these synthetic drugs are linked to various side effects such as cough, taste aversion, skin rashes, or formation of edema.[Citation9] On the other hand, to overcome oxidative stress in hypertension, few studies have shown that (1) diets rich in fruits and vegetables and (2) combination supplement with vitamin C, vitamin E, beta-carotene, and zinc have resulted in significant reduction of blood pressure.[Citation10,Citation11] However, long-term trials have failed to consistently support the antihypertensive effects of antioxidants.[Citation12] Hence, it is clear that natural compounds with both ACE inhibition efficiency as well as antioxidative property are necessary for prevention and treatment of hypertension.
Marine macroalgae or seaweeds are rich in diverse bioactive compounds and are gaining importance in the identification of novel compounds in the prevention or treatments of various diseases. Brown algae Sargassum belongs to the family Sargassaceae and order Fucales is widely distributed in tropical and temperate oceans. Sargassum has been consumed as food and used as medicine in different countries. Sargassum has been found to be rich in bioactive compounds such as phycocolloids, phlorotanins, meroterpenoids, fucoidans, alginic acid, sterols, and glycolipids. Various pharmacological properties which include anticancer, anti-inflammatory, antimicrobial, anticoagulant, antioxidant, hypoglycemic, hypolipidemic, hepatoprotective, and neuroprotective are suggested for Sargassum.[Citation13] Sargassum wightii (SWE) is one of the most important species and distributed in abundance on the southern coasts of Tamil Nadu, India. SWE was less explored for its medicinal properties; only few studies have explained the biological activity of the crude extract prepared from S. wightii.[Citation14–Citation16] In the present study, S. wightii was fractionated using various solvents in Soxhlet extractor. Further, the solvent fraction was subjected to silica gel chromatography to partially purify and identify the solvent fraction rich in bioactive compounds with both ACE inhibition and antioxidant activity. The active fraction was further characterized using spectroscopic analysis to identify various functional groups involved in bioactivity.
Material and methods
Chemicals
ACE from rabbit lung, hippuryl-l-histidyl-l-leucine (HHL), 2,2-diphenyl-1-picrylhydrazyl (DPPH), 2,2-azino-bis-(3-ethylbenzothiazoline-6-sulfonic acid) (ABTS), and 2,4,6-tris-(2-pyridyl-S-triazine) were purchased from Sigma-Aldrich Chemicals Co., USA. Pre-coated thin-layer chromatography plates (silica gel 60 F254, 0.2 mm) were purchased from MERCK, Germany. All other chemicals used were of highest purity available, procured locally.
Brown algae
The brown algae S. wightii was collected along the coastal area of Rameshwaram Island, Tamil Nadu, India. The samples were washed three times with fresh water to remove the attached salts, sand, and epiphytes. Finally, the algae were rinsed carefully with fresh distilled water, shade dried at room temperature, and powdered. The powdered algae were maintained in vacuum-packed bags at deep freezer (−20°C) prior to extraction.
Preparation of the extracts from S. wightii
The schematics showing the extraction of S. wightii are shown in . The active constituents from the S. wightii powder (500 g) were extracted sequentially using solvents (5000 ml; 1:10 w/v) of different polarities, namely, hexane, ethyl acetate, and methanol by Soxhlet extraction at 60–80°C for 6 h. The ethyl acetate fraction (49.73 g) was further chromatographed over a silica gel (60–120 mesh) column with hexane:ethyl acetate with different ratios (9.5–7.5:0.5–2.5), yielding five subfractions. The subfraction F4 (150 mg) with significant ACE I inhibitory activity (data not shown) was further subjected to preparative thin-layer chromatography (silica gel 60 F254, 0.2 mm) using hexane:ethyl acetate (9:1) solvents as mobile phase. The band with retention factor value 0.42 was scraped off carefully from the plate. The scratched sample was dissolved in ethyl acetate (40 ml) and centrifuged at 12,000 rpm for 15 min to remove silica. The extract was concentrated by the vacuum rotary evaporator at 40°C under reduced pressure (240 mbar). The concentrated partially purified S. wightii ethyl acetate extract (SWE; 30 mg) was solubilized in 10% dimethyl sulfoxide (DMSO) and used for further analysis.
FT-IR analysis of active compounds of ethyl acetate fraction from S. wightii
The infrared spectral analysis was performed by Shimadzu FT-IR 8300 instrument, Japan. Potassium bromide pellet was prepared by mixing 5 mg of SWE from S. wightii with 100 mg of anhydrate potassium bromide. The spectrum was recorded in the range at 4000–400 cm−1.
HPLC–ESI–MS analysis of active compounds from ethyl acetate fraction
The identification of active compounds in SWE was performed by mass spectrometry (MS) analyses on an Agilent 6520 Q-TOP-MS/MS system coupled with an Agilent 1200 HPLC (Agilent technology, USA). The mobile phase consisting of a 0.1% formic acid aqueous solution (A) and methanol (B) was delivered at a flow rate of 0.3 ml/min under an isocratic program: 40% A and 60% B for 2 min. The sample injection volume was 1 µl. The capillary temperature was set to 310°C and nebulizer pressure to 35 psi. The drying gas flow rate was 10 l/min. Detection was carried out within a mass range of m/z 10–1000.
In vitro antioxidant activity of SWE
Various antioxidant activities, such as scavenging activity of DPPH and ABTS as well as Ferric Reducing Antioxidant Power (FRAP) assay, were carried out as described earlier.[Citation17–Citation19] For different radical scavenging activities, 20–100 µg/ml concentration of SWE was used. All the absorbance was recorded using Shimadzu UV-1800 (Japan) UV–vis spectrophotometer. Ascorbic acid was used as a positive control for the entire antioxidant assay.
ACE enzyme activity and inhibitor studies
The enzyme activity of ACE was determined by the methods of Cushman and Cheung[Citation20] with slight modifications as described in Ko et al.[Citation21] Briefly, assay mixture consisting of 100 µl of the ACE enzyme (25 mU/ml) and 200 µl of 8.0 mM HHL was incubated at 37°C for 30 min. After incubation, the reaction was stopped by the addition of 600 µl of 1 M HCl. The hippuric acid was isolated by addition of 500 µl of ethyl acetate. About 200 µl of upper layer was transferred to a clean test tube and evaporated in the dry-oven at 80°C for 1 h. The dried residue was dissolved in 1 ml of distilled water, and its absorbance was measured at 228 nm.
For inhibitor studies, concentrated stock solution of SWE was further diluted with distilled water to obtain required working concentration solution. The final concentration of DMSO in assay mixture is maintained at <0.1% which did not affect the ACE enzyme activity. ACE enzyme was incubated with different concentration of SWE at 4°C for 30 min. After incubation, the ACE enzyme activity was determined as given above. A standard drug captopril was used as positive control.
Kinetic determination
The inhibition kinetics of ACE by SWE was determined by Lineweaver and Burk double reciprocal plot and Dixon plot.[Citation22,Citation23] ACE enzyme inhibition was determined over a range of HHL concentrations (2–8 mM) in the absence and in the presence of SWE (60–100 µg/ml). All the assays were carried out in two sets of triplicates and data were presented as mean ± SD.
Results
Characterization of SWE
Successive solvent extraction was performed using different solvents in the order of increasing polarity. Ethyl acetate extract was further subjected to silica gel column fractionation and TLC separation to obtain active extract called SWE. The phenol content present in SWE was found to be 49.10 ± 2.52 µg/mg equivalent to gallic acid. The FT-IR spectrum of SWE was shown in . Broad absorbance peak observed at 3441 cm−1 corresponds to O–H stretching might be due to the presence of polyols in the SWE. Appearance of peak at 2924 and 2855 cm−1 is characteristic band for C–H stretching vibration of aromatics and alkanes, respectively. The presence of alkene stretch was assigned by the band at 1692.82 cm−1. The strong peak at 1596.15 cm−1 corresponds to aromatic C=C bond. The peaks identified near at 1398.96 and 1222.59 cm−1 correspond to C–O bending vibrations. The medium peak at 1161.44, 1110.85, and 1036.70 cm−1 corresponds to alkoxy and phenol C–O stretch. The peak at 837.38 and 725.17 cm−1 corresponds to CH bend of substituted aromatic. Altogether, the FT-IR spectrum is in good agreement with the presence of phenolic compounds in the SWE. Further, SWE resolved into 15 peaks in LC analysis (). The corresponding m/z obtained through positive mode ESI–MS spectra for each peak resolved in LC was given in .
Table 1. Retention time of various peak in LC and corresponding [M + H+] m/z obtained through ESI–MS analysis of SWE.
Antioxidant activities of SWE
SWE exhibited significant concentration-dependent scavenging activity of various radicals such as DPPH and ABTS (, ). At 100 µg/ml concentration, SWE showed about 76% of DPPH scavenging activity. The IC50 values for DPPH scavenging were found to be 59.91 and 3.87 µg/ml for SWE and ascorbic acid, respectively. In ABTS assay, the IC50 value for SWE was found to be 51.04 µg/ml. SWE at 100 µg/ml concentration showed about 81% ABTS scavenging activity. In addition, SWE significantly reduced Fe3+ ions to Fe2+ ions which were measured using FRAP assay (). In FRAP assay, the IC50 value of SWE was found to be 55.21 μg/ml. In the presence of 100 µg/ml concentration of SWE, the FRAP was found to be about 77%.
Figure 5. Effect of SWE on ACE activity. Percentage inhibition of SWE and standard drug captopril relative to control at various concentrations are shown (A). The control (100%) activity corresponds to the activity devoid of any added SWE. LB plot (B) and Dixon plot (C) for the inhibition of SWE on ACE activity are shown. Results are expressed as mean ± SD (n = 6).
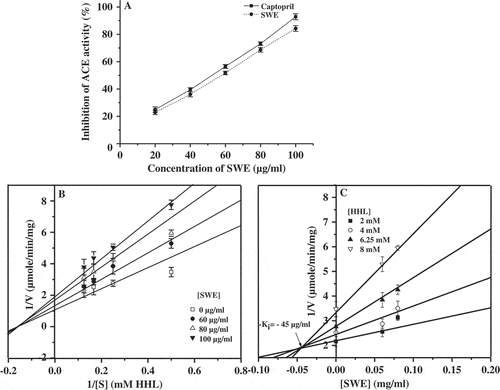
Inhibition of ACE by SWE
The effect of SWE on ACE activity is shown in . From the graph, it is clear that SWE showed dose-dependent inhibition of ACE activity. The inhibition of SWE is equivalent to that of standard drug captopril. The IC50 value was found to be 56.96 and 51.79 µg/ml for SWE and captopril, respectively. The Lineweaver–Burk (LB) plot also known as double reciprocal plot is obtained by plotting inverse velocity as a function of the inverse of the substrate concentration. In general, the LB plot is used to determine the type of inhibition by the inhibitor. The LB plot in the presence of SWE indicated that the Km value increased and Vmax decreased for ACE activity. The Michaelis constant Km value was found to be −0.16 mM. This result indicates that SWE inhibits the ACE activity in a mixed-type manner (). Dixon plot has been used in conjunction with LB plot to determine the inhibitor constant (Ki). Dixon plot is obtained by plotting inverse velocity against the inhibitor concentration, at two or more values of the substrate concentration. The corresponding Ki value of SWE against ACE is 45 µg/ml as revealed by Dixon plot ().
Discussion
Hypertension leads to various cardiovascular diseases; hence, researchers are keen in identifying efficient bioactive compounds that act against hypertension and lower the level of cardiovascular disease. ACE enzyme inhibitors and antioxidants are considered important therapeutic strategy to overcome hypertension. Due to increased risk of side effects of synthetic compounds, alternative and complementary medicine which consists of either pure bioactive substances or extracts that contain various bioactive components from natural sources are gaining importance to overcome hypertension. Various brown algae were studied for their antihypertensive property; however, S. wightii predominantly present in southern coasts of Tamil Nadu, India, are less explored for its antihypertensive property. In the present study, it has been shown that ethyl acetate extract of S. wightii has potent antioxidant and ACE inhibition property which can be further applied as anti-hypertensive therapeutics.
The ethyl acetate fraction of S. wightii was further purified by silica gel chromatography and preparative TLC to obtain SWE which contained bioactive principles. Previous study has shown that ethyl acetate extraction of brown algae results in phlorotannin rich fraction. In accordance to these observations, in the present study, the quantitative analysis of SWE showed the presence of phenolic compounds. Further, the FT-IR spectrum of SWE is similar to that of standard phloroglucinol.[Citation24] In a previous study, FT-IR analysis of phloroglucinol resulted in major peaks in the range of 3070–521 cm−1. Similarly, in SWE, the peak at 3441 cm−1 (3070 cm−1 in phloroglucinol) due to OH group, peak at 2924 cm−1 (2942 cm−1 in phloroglucinol) due to C–H stretching vibration of aromatics, peak at 1596.15 cm−1 (1514 cm−1 in phloroglucinol) due to aromatic C=C stretch, peaks at 1161.44, 1110.85, and 1036.70 cm−1 (1161 and 1011 cm−1 in phloroglucinol) due to phenol C–O stretch confirms the presence of phloroglucinol units in the SWE.[Citation24] It is well known that brown algae are a rich source of phlorotannin molecules. Phlorotannins are polyphenolic compounds produced due to polymerization of phloroglucinol units. Phlorotannins are distinguished based on bond type between the phloroglucinol units (diphenyl ethers or biphenyls), presence of number of OH group, and number of phloroglucinol units polymerized. SWE when subjected to LC separated into 15 peaks, in which 12 peaks showed the presence of compound with m/z [protonated molecular ions (M + H+)] greater than 900. From the literature, it is presumed that the peak 7 with m/z 992 might be octaphlorethol A[Citation25], peak 9 with m/z 994 might be fucophloroethols with eight units of phloroglucinol,[Citation26] and peak 15 with m/z 972 might be 2,7 phloroglucinol-6,6ʹ-bieckol.[Citation27] All the other compounds with the obtained m/z have not been identified yet. Altogether, through the results obtained from FT-IR and LC–ESI–MS analysis of SWE, it is safe to assume that the SWE is enriched in phlorotannins with complex structure and high molecular weight.
The SWE showed potent DPPH and ABTS radical scavenging activity. The phlorotannins present in the SWE might have donated the hydrogen atom for stabilization of the radicals. In addition, the SWE showed potent Fe3+-reducing power. The potent FRAP activity shown by SWE can be correlated with the phenolic nature of the phlorotannins. It has also been shown that number and location of OH group also will play a vital role in reducing Fe2+. The efficient antioxidant and metal scavenging activity of SWE can be positively applied against hypertension treatment. Most importantly, it has been found that SWE possess significant ACE inhibition property which is comparable to that of standard drug captopril. Among the various Korean sea weed studied, the ethanol fraction of Ecklonia stolonifera, Ecklonia cava, Pelvetia siliquosa, Undaria pinnatifida, and Gentianella tenella had shown ACE inhibition.[Citation28] In another study, the enzymatic digest of various brown sea weed such as E. cava, Ishige okamurae, Sargassum fulvellum, Sargassum horneri, Sargassum coreanum, Sargassum thunbergii, and Scytosiphon lomentaria had shown ACE inhibition.[Citation29] Also, recently Fucus spiralis extract was shown to possess ACE inhibition.[Citation30] However, phlorotannin enriched E. cava was shown to be potent ACE inhibitor in comparison to other brown algae. The SWE fraction obtained using S. wightii in the present study has shown similar potential of ACE inhibition in comparison to E. cava. Among the various phlorotannin molecules, the compounds such as eckol, phlorofucofuroeckol A, dieckol, phloroglucinol, triphlorethol-A, octaphlorethol A, and 6,6ʹ bieckol were reported for its ACE inhibition.[Citation21,Citation28,Citation31,Citation32] However, low molecular weight phlorotannin showed only less ACE inhibition. Among these phlorotannins, phlorofucofuroeckol A (pentamer), dieckol (hexamer), 6,6ʹ bieckol (hexamer), and octaphlorethol A (octamer) had shown significantly higher ACE inhibition.[Citation21,Citation28,Citation31,Citation32] SWE which is rich in high molecular weight phlorotannins would be advantageous in the inhibition of ACE. The mechanism or structure activity relationship for phlorotannin inhibition of ACE is not well established till date. It has been postulated that protein precipitation property of tannins might play an important role in ACE inhibition; however, precipitation of proteins was not observed in the ACE assay with the concentration of SWE used in the present study. Similarly, tannic acid which also belongs to tannins along with phlorotannins showed potent ACE inhibition in the concentration much lower than required to precipitate protein. The docking of tannic acid did not fit into the active site of ACE due to its large polymer size.[Citation33] However, binding of phlorotannins on ACE either through peptide or amide linkages might result in reduced efficiency of ACE enzyme activity. Also, it has been proposed that a closed ring dibenzo-1,4-dioxin moiety may be crucial to the ACE inhibition by phlorotannins.[Citation34] In addition, tannins isolated from Chinese herbs were shown to inhibit ACE through sequestration of Zn2+ which is present in active site of the enzyme.[Citation35] In contrast to these observations, a recent study has shown the binding of octaphlorethol A and 6, 6′ bieckol in the active site of the ACE using in silico tool. However, such binding did not affect the Zn2+ present in the active site.[Citation21,Citation32] Hence, an elaborate study is required to understand the mechanism of inhibition of ACE by phlorotannins. The enzyme kinetics studies have shown that SWE inhibited ACE in a mixed-type of inhibition from which it can be inferred that bioactive components of SWE might bind to ACE irrespective of substrate binding to the enzyme. Similarly, dieckol was found to inhibit ACE in noncompetitive manner.[Citation21] The results from kinetic study reveal that it is unlikely for bioactive components present in SWE to interact with the active site of the enzyme. Either interaction of bioactive components on the ACE enzyme other than active site or sequestration of Zn2+ might have resulted in the inhibition of ACE.
Conclusion
Whole solvent extracts or pure phlorotannin isolated from various brown algae were widely studied for its different therapeutic application. The results from the present study clearly demonstrate that SWE fraction obtained by extraction of S. wightii using ethyl acetate and partially purified using silica column chromatography and preparative TLC had shown both antioxidant and ACE inhibition property. Such ACE inhibition by SWE is efficient similar to that of well-known drug captopril. By spectroscopic, quantitative determination of phenolics and LC-MS ESI analysis, the presence of various phlorotannin compounds in SWE is speculated for its biological property. Further, purification of each components present in SWE is required to completely elucidate the bioactive principle involved in antioxidant and ACE inhibition of SWE. In addition, in vivo study using rat model is required to understand the efficacy of SWE for treatment against hypertension. The biological activities shown by brown algae S. wightii in vitro in the present study further reveal the potential for producing functional food products using the brown algae for the health benefit of the humans.
Acknowledgments
The authors also extent their acknowledgement to Dr. M. Palanisamy, Scientist D, Botanical Survey of India, Tamil Nadu Agriculture University, Coimbatore, India for the identification and authentication of brown algae as Sargassum wightii Greville. All authors declare no conflict of interest.
Additional information
Funding
References
- Lim, S.S.; Vos, T.; Flaxman, A.D.; Danaei, G.; Shibuya, K.; Adair-Rohani, H.; et al. A Comparative Risk Assessment of Burden of Disease and Injury Attributable to 67 Risk Factors and Risk Factor Clusters in 21 Regions, 1990-2010: A Systematic Analysis for the Global Burden of Disease Study 2010. Lancet 2012, 380, 2224–2260. DOI: 10.1016/S0140-6736(12)61766-8.
- Grossman, E.;. Does Increased Oxidative Stress Cause Hypertension? Diabetes Care 2008, 31, S185–S189. DOI: 10.2337/dc08-s246.
- Nakazono, K.; Watanabe, N.; Matsuno, K.; Sasaki, J.; Sato, T.; Inoue, M. Does Superoxide Underlie the Pathogenesis of Hypertension? Proceedings of the National Academy of Sciences of the United States of America 1991, 88, 10045–10048. DOI: 10.1073/pnas.88.22.10045.
- Paravicini, T. M.; Touyz, R. M. Redox Signaling in Hypertension. Cardiovascular research 2006, 71, 247–258. DOI: 10.1016/j.cardiores.2006.05.001.
- Gonzalez, J.; Valls, N.; Brito, R.; Rodrigo, R. Essential Hypertension and Oxidative Stress: New Insights. World journal of cardiology 2014, 6, 353–366. DOI: 10.4330/wjc.v6.i6.353.
- Rajagopalan, S.; Kurz, S.; Munzel, T.; Tarpey, M.; Freeman, B. A.; Griendling, K. K.; Harrison, D. G. Angiotensin II-mediated Hypertension in the Rat Increases Vascular Superoxide Production via Membrane NADH/NADPH Oxidase Activation. Contribution to Alterations of Vasomotor Tone. Journal of clinical investigation 1996, 97, 1916–1923. DOI: 10.1172/JCI118623.
- Laursen, J. B.; Rajagopalan, S.; Galis, Z.; Tarpey, M.; Freeman, B. A.; Harrison, D. G. Role of Superoxide in Angiotensin II-induced but Not Catecholamine-Induced Hypertension. Circulation 1997, 95, 588–593. DOI: 10.1161/01.CIR.95.3.588.
- Kumar, K. V.; Das, U. N. Are Free Radicals Involved in the Pathobiology of Human Essential Hypertension? Free radical research communications 1993, 19, 59–66. DOI: 10.3109/10715769309056499.
- Parish, R. C.; Miller, L. J. Adverse Effects of Angiotensin Converting Enzyme (ACE) Inhibitors. An Update. Drug Safety 1992, 7, 14–31. DOI: 10.2165/00002018-199207010-00004.
- Ardalan, M. R.; Rafieian-Kopaei, M. Antioxidant Supplementation in Hypertension. Journal of renal injury prevention 2014, 3, 39–40.
- Tamadon, M. R.; Baradaran, A.; Rafieian-Kopaei, M. Antioxidant and Kidney Protection; Differential Impacts of Single and Whole Natural Antioxidants. Journal of renal injury prevention 2014, 3, 41–42.
- Baradaran, A.; Nasri, H.; Rafieian-Kopaei, M. Oxidative Stress and Hypertension: Possibility of Hypertension Therapy with Antioxidants. Journal of research in medical sciences 2014, 19, 358–367.
- Liu, L.; Heinrich, M.; Myers, S.; Dworjanyn, S. A. Towards A Better Understanding of Medicinal Uses of the Brown Seaweed Sargassum in Traditional Chinese Medicine: A Phytochemical and Pharmacological Review. Journal of ethnopharmacology 2012, 142, 591–619. DOI: 10.1016/j.jep.2012.05.046.
- Syad, A. N.; Shunmugiah, K. P.; Kasi, P. D. Antioxidant and Anti-Cholinesterase Activity of Sargassum Wightii. Pharmaceutical biology 2013, 51, 1401–1410. DOI: 10.3109/13880209.2013.793721.
- Sujatha, D.; Singh, K.; Vohra, M.; Kumar, K. V.; Sunitha, S. Antilithiatic Activity of Phlorotannin Rich Extract of Sarghassum Wightii on Calcium Oxalate Urolithiais - InVitro and in Vivo Evaluation. International brazilian journal of uUrology 2015, 41, 511–520. DOI: 10.1590/S1677-5538.IBJU.2014.0357.
- Maneesh, A.; Chakraborty, K.; Makkar, F. Pharmacological Activities of Brown Seaweed Sargassum Wightii (Family Sargassaceae) Using Different in Vitro Models. International journal of food properties 2017, 20, 931–945. DOI: 10.1080/10942912.2016.1189434.
- Blois, S.;. A Note on Free Radical Formation in Biologically Occurring Quinones. Biochimica et biophysica acta 1955, 18, 165. DOI: 10.1016/0006-3002(55)90038-X.
- Benzie, I. F.; Strain, J. J. The Ferric Reducing Ability of Plasma (FRAP) as a Measure of “Antioxidant Power”: The FRAP Assay. Analytical biochemistry 1996, 239, 70–76. DOI: 10.1006/abio.1996.0292.
- Re, R.; Pellegrini, N.; Proteggente, A.; Pannala, A.; Yang, M.; Rice-Evans, C. Antioxidant Activity Applying an Improved ABTS Radical Cation Decolorization Assay. Free radical biology and medicine 1999, 26, 1231–1237. DOI: 10.1016/S0891-5849(98)00315-3.
- Cushman, D. W.; Cheung, H. S. Spectrophotometric Assay and Properties of the Angiotensin-Converting Enzyme of Rabbit Lung. Biochemical pharmacology 1971, 20, 1637–1648. DOI: 10.1016/0006-2952(71)90292-9.
- Ko, S.-C.; Jung, W.-K.; Kang, S.-M.; Lee, S.-H.; Kang, M. C.; Heo, S.-J.; Kang, K.-H.; Kim, Y.-T.; Park, S.-J.; Jeong, Y. Angiotensin I-Converting Enzyme (ACE) Inhibition and Nitric Oxide (No)-Mediated Antihypertensive Effect of Octaphlorethol A Isolated from Ishige Sinicola: In Vitro Molecular Mechanism and in Vivo SHR Model. Journal of functional foods 2015, 18, 289–299. DOI: 10.1016/j.jff.2015.07.003.
- Lineweaver, H.; Burk, D. The Determination of Enzyme Dissociation Constants. Journal of the american chemical society 1934, 56(3), 658–666. DOI: 10.1021/ja01318a036.
- Dixon, M.;. The Determination of Enzyme Inhibitor Constants. Biochemical journal 1953, 55, 170–171. DOI: 10.1042/bj0550170.
- Leyton, A.; Pezoa-Conte, R.; Barriga, A.; Buschmann, A.; Mäki-Arvela, P.; Mikkola, J.-P.; Lienqueo, M. Identification and Efficient Extraction Method of Phlorotannins from the Brown Seaweed Macrocystis Pyrifera Using an Orthogonal Experimental Design. Algal research 2016, 16, 201–208. DOI: 10.1016/j.algal.2016.03.019.
- Lee, S. H.; Kang, S. M.; Ko, S. C.; Kang, M. C.; Jeon, Y. J.; Octaphlorethol, A. A Novel Phenolic Compound Isolated from Ishige Foliacea, Protects against Streptozotocin-Induced Pancreatic Beta Cell Damage by Reducing Oxidative Stress and Apoptosis. Food and chemical toxicology 2013, 59, 643–649. DOI: 10.1016/j.fct.2013.07.011.
- Ferreres, F.; Lopes, G.; Gil-Izquierdo, A.; Andrade, P. B.; Sousa, C.; Mouga, T.; Valentão, P. Phlorotannin Extracts from Fucales Characterized by HPLC-DAD-ESI-MSn: Approaches to Hyaluronidase Inhibitory Capacity and Antioxidant Properties. Marine drugs 2012, 10, 2766–2781. DOI: 10.3390/md10122766.
- Kang, S.-M.; Heo, S.-J.; Kim, K.-N.; Lee, S.-H.; Jeon, Y.-J. Isolation and Identification of New Compound, 2, 7 ″-Phloroglucinol-6, 6′-Bieckol from Brown Algae, Ecklonia Cava and Its Antioxidant Effect. Journal of functional foods 2012, 4, 158–166. DOI: 10.1016/j.jff.2011.10.001.
- Jung, H. A.; Hyun, S. K.; Kim, H. R.; Choi, J. S. Angiotensin‐Converting Enzyme I Inhibitory Activity of Phlorotannins from Ecklonia Stolonifera. Fisheries science 2006, 72, 1292–1299. DOI: 10.1111/fis.2006.72.issue-6.
- Athukorala, Y.; Jeon, Y.-J. Screening for Angiotensin 1-Converting Enzyme Inhibitory Activity of Ecklonia Cava. Preventive nutrition and food science 2005, 10, 134–139. DOI: 10.3746/jfn.2005.10.2.134.
- Paiva, L.; Lima, E.; Neto, A. I.; Baptista, J. Angiotensin I-Converting Enzyme (ACE) Inhibitory Activity of Fucus Spiralis Macroalgae and Influence of the Extracts Storage temperature-A Short Report. Journal of pharmaceutical and biomedical analysis 2016, 131, 503–507. DOI: 10.1016/j.jpba.2016.08.029.
- Wijesinghe, W.; Ko, S.-C.; Jeon, Y.-J. Effect of Phlorotannins Isolated from Ecklonia Cava on Angiotensin I-Converting Enzyme (ACE) Inhibitory Activity. Nutrition research and practice 2011, 5, 93–100. DOI: 10.4162/nrp.2011.5.2.93.
- Ko, S.-C.; Kang, M. C.; Kang, N.; Kim, H.-S.; Lee, S.-H.; Ahn, G.; Jung, W.-K.; Jeon, Y.-J. Effect of Angiotensin I-Converting Enzyme (ACE) Inhibition and Nitric Oxide (NO) Production of 6, 6′-Bieckol, a Marine Algal Polyphenol and Its Anti-Hypertensive Effect in Spontaneously Hypertensive Rats. Process biochemistry 2017, 58, 326–332. DOI: 10.1016/j.procbio.2017.04.014.
- Al Shukor, N.; Van Camp, J.; Gonzales, G. B.; Staljanssens, D.; Struijs, K.; Zotti, M. J.; Raes, K.; Smagghe, G. Angiotensin-Converting Enzyme Inhibitory Effects by Plant Phenolic Compounds: A Study of Structure Activity Relationships. Journal of agricultural and food chemistry 2013, 61, 11832–11839. DOI: 10.1021/jf404641v.
- Wijesekara, I.; Kim, S.-K. Angiotensin-I-converting Enzyme (ACE) Inhibitors from Marine Resources: Prospects in the Pharmaceutical Industry. Marine Drugs 2010, 8, 1080–1093. DOI: 10.3390/md8041080.
- Liu, J.-C.; Hsu, F.-L.; Tsai, J.-C.; Chan, P.; Liu, J. Y.-H.; Thomas, G. N.; Tomlinson, B.; Lo, M.-Y.; Lin, J.-Y. Antihypertensive Effects of Tannins Isolated from Traditional Chinese Herbs as Non-Specific Inhibitors of Angiontensin Converting Enzyme. Life Sciences 2003, 73, 1543–1555. DOI: 10.1016/S0024-3205(03)00481-8.