ABSTRACT
Structured lipids (SL) were synthesized via enzymatic (EI) and chemical interesterification of high oleic sunflower oil (SO) and fully hydrogenated high oleic SO with Lipozyme TL IM (Thermomyces lanuginose) for 3 h at 70°C, 300 rpm. Reaction showed changes in the triacylglycerols (TAGs) composition, solid fat content (SFC), thermal behavior, regiospecific distribution, microstructure, and polymorphism. Results revealed that the EI caused considerable rearrangement of the TAG species with lower levels of tri-saturated and tri-unsaturated TAG and higher levels of monoun- and diunsaturated TAG. The interesterified blends showed reduced SFC between 20 and 35°C, lowering the melting point. After 3-h incubation, EI produced acyl migration to some extent. The SL showed the required characteristics for application as bakery fats and as additives for lipid crystallization in the food industry.
Introduction
The physical characteristics of oils and fats are determined by their chemical compositions such as the types of fatty acid (FA) and their distribution along the glycerol backbone. Most fats and oils have only limited application in their original forms. High oleic sunflower oil (HOSO) shows high thermo-oxidative stability, due the high levels of oleic acid (over 88%). These types of oils could be great value in foods (e.g., fried products, bakery products and margarines, and frozen desserts).[Citation1]
Different strategies can be used to modify the original composition of oils such as total hydrogenation, interesterification, and fractionation. Interesterification is one of the most important methods used to change the TAG composition, aimed at producing low-trans or trans-free fats with appropriate crystallization behavior and desirable texture.[Citation2]
Structured lipids (SL) are developed to obtain restructured oils or fats with higher nutritional value and metabolic activity by adding different sized FA chains to the glycerol molecule, as well as changing their position on the molecule, according to the purpose of the application.[Citation3] SL can be obtained by chemical and enzymatic synthesis. Several studies have shown that interesterification by enzyme catalysis is more efficient with respect to selectivity and reaction control as compared to chemical catalysis.[Citation4,Citation5] This selectivity is dependent on the type of enzyme used, and also immobilization and the reaction conditions. The enzymatic reactions are carried out under mild conditions, reducing the energy required and making it easier to recover the products and to produce lipids of higher nutritional quality.[Citation6] Fats and oils of vegetable origin are generally rich in unsaturated FAs (UFAs) in the central position (sn-2) of the triacylglycerols (TAGs), while the saturated FAs (SFAs) principally occupy the positions sn-1 and sn-3. SFAs in the sn-2 position of dietary TAG may elevate the LDL concentrations more than the same FA in the sn-1 or sn-3 positions, because the metabolic fat is different to that of the sn-1 and sn-3 positions.[Citation7] The synthesis via enzymatic catalysis using immobilized sn-1,3-specific lipase presents the advantage of controlling the process, selectivity, and regiospecificity.[Citation8,Citation9] Pacheco et al. evaluated the occurrence of the acyl migration phenomenon during the specific lipase-catalyzed interesterification of liquid and fully hydrogenated soybean oil.[Citation10] They observed acyl migration at the sn-2 position even when using sn-1,3-specific lipase, and this effect was related to the type of lipase and the concentration, temperature, and addition of solvent. However, this type of biocatalyst is still preferred as compared to nonspecific or chemical catalysts. Several researchers have studied enzymatic synthesis using an sn-1,3-specific lipase as the biocatalyst in different applications, such as human milk fat substitutes, cocoa butter equivalents, and low-trans or trans-free plastic fats.[Citation11–Citation16]
Lipozyme TL IM is an sn-1,3-specific lipase from Thermomyces lanuginosus immobilized on granulated silica via ionic adsorption. This immobilization of the lipase on a support may alter its performance in many interesting ways, such as selective hydrolysis or oxidations, kinetic resolutions of racemic mixtures, or kinetically controlled synthesis (pH and temperature). Lipozyme TL IM is indicated for use in the food industry (e.g., enzymatic synthesis of medium and long-chain TAGs, human milk fat substitutes, cocoa butter equivalents, low-trans or trans-free plastic fats, monoacylglycerols, and diacylglycerols).[Citation17-Citation19] Lee et al. optimized the enzymatic interesterification (EI) reaction of palm kernel oil and palm oil blends with Lipozyme TL IM for the medium- and long-chain TAGs using a faced central composite design.[Citation20] To date, most studies have only focused on the melting properties of different ratios of lipid blends for various applications in the food industries, such as confectionary, margarine, and shortening, which are beneficial to the health. Ifeduba et al. studied the EI of HOSO and tripalmitin or tristearin with Lipozyme TL IM to produce low-saturated, zero-trans plastic fats.[Citation21]
Ribeiro et al.[Citation22] studied the EI of HOSO and fully hydrogenated soybean oil for 9 h, and samples were collected for analysis at 3, 6, and 9 h of process, evaluating the thermal behavior (differential scanning calorimeter [DSC]) and acyl migration. The reaction with 3 h showed the advantage of selectivity for production of new TAG, lower acyl migration when compared with the other reaction times, evidenced by 13C NMR and lower levels of partial acylglycerols. The aim of this study was to develop sustainable processes, with low-energy consumption and short reaction times, low acyl migration, and specific final products obtained in the EI catalyzed by a sn-1,3-specific lipase, of binary blend of SO and fully hydrogenated high oleic sunflower oil (FHSO) in different weight ratios (60:40, 50:50, 40:60, 30:70) using 7% (w/w) Lipozyme TL IM at 70°C for 3 h. Chemical interesterification (CI) was carried out as the control. These SL were projected to have broad applications in foods, such as in bakery/confectionery fats and a lipid crystallization additive.
Material and methods
Material
The ready-to-eat deodorized HOSO was provided by the company Dow AgroSciences—distributed by the company Quantiq Ltda (São Paulo, Brazil). The FHSO, ready for industrial use, was provided by the company Sina S.A. (Pirapozinho, São Paulo, Brazil). The FA compositions are given in . The immobilized enzyme Lipozyme TL IM (Thermomyces lanuginosus lipase, sn-1,3-specific, specific activity of 329 IUN/g, IUN = interesterification unit) was donated by the company Novozymes do Brasil Ltda (Araucária, Brazil). The chemical catalyzer, 99% powdered anhydrous sodium methoxide, was obtained from Sigma-Aldrich (St. Louis, MO, United States).
Table 1. Fatty acid compositions (%) of the raw materials, high oleic sunflower (SO), fully hydrogenated high oleic sunflower oil (FHSO), and the binary blends (SO:FHSO).
Preparation of the blends (BE) and conditioning of the enzyme
During the process, 100 g samples of SO and FHSO were prepared and melted in each of the following proportions to form the blends: 60:40, 50:50, 40:60, and 30:70 (w/w). The blends were maintained at 80°C for 30 min using a magnetic stirrer to destroy their crystalline memory and then stored in glass vials under refrigeration (−5°C). On a laboratory scale, the sn-1,3-specific lipase (Lipozyme TL IM) was conditioned to remove the moisture. The Lipozyme TL IM had a moisture content of 5% (w/w), and the manufacturer (Novozymes) recommended reducing this content before carrying out the experiment in order to decrease the free fatty acids (FFA) and the mono (MAG) and diacylglycerols (DAG) formed by hydrolysis of the fat. To this, 100 g of the binary blend was added in a 250-mL rounded bottom flask and heated in a mineral oil bath at 70–75°C. When the temperature of the mixture reached 70°C, 7% (w/w) of the enzyme was added and the blend was agitated at 300 rpm using magnetic stirring under nitrogen atmosphere for 30 min. The stirring was turned off, the enzyme was decanted, and the top oil phase was removed with a pipette. The conditioned enzyme was used immediately in the EI.
EI
On the process, 100 g of each blend produced in the proportions of 60:40, 50:50, 40:60, and 30:70 (w/w) was added to a round-bottomed flask containing 7% of conditioned enzyme and heated in a mineral oil bath at 70–75°C with magnetic shaking at 300 rpm in an atmosphere of nitrogen. The total reaction time was 3 h and samples were collected for analysis and placed in screw-topped test tubes, maintaining the shaking. Pure nitrogen was passed through the mixture, the tube closed and submitted to 10 min heating in a water bath at 95°C to inactivate the enzyme. The FFA content was determined as oleic acid (% in weight) according to the American Oil Chemists’ Society Official Method No. Ca 5a-40 (2009).[Citation23] To remove the FFA, the sample was filtered into a twin-walled separating funnel in a heated water bath to avoid solidification of the mixture. Five drops of a phenolphthalein solution were then added, followed by 1 mL of 0.2 M NaOH. The interesterified mixture was washed with hot water (50–60°C) until disappearance of the pink color and then filtered through filter paper containing anhydrous sodium sulfate to remove the moisture and biocatalyzer particles. The products were dried under vacuum at 110°C for 30 min and then stored under refrigeration (−5°C) in glass vials.
CI
CI was carried out with the same proportions of SO and FHSO used in EI. On a laboratory scale, 100 g of each blend was interesterified under magnetic shaking in the presence of 0.4% (w/w) sodium methoxide at a temperature of 100°C. The reaction time was 20 min under vacuum, according to the optimization carried out by Grimaldi, Gonçalves, and Ando.[Citation24] The reaction was then interrupted by adding 5 mL of the 5% citric acid solution (5 g/100 mL) and the interesterified samples washed with distilled water (80°C). To remove the moisture, anhydrous sodium sulfate was added to the filter paper through which the samples were filtered under vacuum, followed by drying at 110°C for 30 min. The samples were stored under refrigeration (−5°C) in glass vials.
FA composition
The fatty acid methyl esters were prepared according to the method of Hartman & Lago.[Citation25] The FA content was determined according to the American Oil Chemists’ Society Official Method No. Ce 1f-96 (2009).[Citation23] The chromatographic operating conditions were Agilent 6850 Series capillary gas chromatograph, equipped with a DB-23 Agilent capillary column (50% cyanopropyl-methylpolysiloxane), dimensions: 60 m, 0.25 mm i.d., and 0.25 µm film. The analytical conditions were oven temperature: 110–215°C (5°C/min), 215°C—24 min; detector temperature: 280°C; injector temperature: 250°C; carrier gas: helium; split ratio 1:50; injection volume: 1.0 µL. The qualitative composition was identified by comparing the peak retention time with the peaks of the respective FA standards. The analysis was carried out in duplicate and the average values reported with their standard deviation.
Analysis of TAG profiles
The TAG composition was determined according to the American Oil Chemists’ Society Official Method No. Ce 5-86 (2009) using gas chromatography (CGC Agilent 6850 Series GC System).[Citation23] A DB-17HT capillary column (Agilent Catalog 122–1811—50% phenylmethylpolysiloxane), with a length of 15 m, internal diameter of 0.25 mm, and film thickness of 0.15 µm, was used. The analytical conditions were split injection, 1:100 ratio; column temperature: 250°C, programmed to increase to 350°C at a rate of 5°C/min; stripping gas: helium at 1.0 mL/min; injector temperature: 360°C; detector temperature: 375°C; volume injected: 1.0 µL; sample concentration: 10 mg/mL of tetrahydrofuran. The TAG groups were identified by comparison of the retention times, according to Antoniosi Filho, Mendes and Lanças.[Citation26] The analysis was carried out in duplicate and the average values reported with their standard deviations.
Acylglycerides composition
The acylglycerides composition (TAG, DAG, and others) was determined by high-performance liquid chromatography in a series 250 Perkin Elmer chromatograph (Waltham, MA, USA) equipped with a Sicon Analytic refractive index detector (Hitachi High Technologies America, Schaumburg, IL, USA) and two columns (500 Å, 7.8 × 300 mm and 100 Å, 300 × 7.8 mm) (Jordi Gel DVB, Apple Valley, MN, USA). The analytical conditions were samples diluted in a ratio of 1:100 (v/v) with tetrahydrofuran; mobile phase: tetrahydrofuran (HPLC grade); flow: 1 mL/min, injection volume: 20.0 µL.[Citation27] The analyses were carried out in triplicate and the average values reported with their standard deviations.
Regiospecific distribution
Approximately 150 mg of each sample was liquefied by heating with hot air and immediately dissolved in oxygen-free deuterated chloroform (500 µL, CDCl3) using tetramethylsilane (1%, TMS) as the chemical shift reference. The blends were maintained in an ultrasound bath at 60°C until a homogenous solution was obtained and then transferred to 5 mm NMR tubes. Quantitative 13C NMR spectra were acquired at 30°C without twisting under the control of an ICON-NMR (Bruker Biospin, Rheinstetten, Germany) with a Bruker Avance-III (500 MHz) spectrometer operating at 125.69 MHz for 13C, equipped with a 5-mm BBO probe. The following acquisition parameters were used: 13C excitation pulse 90° for 10 μs, sweep-width of 238 ppm, repetition time of 25 s (acquisition time 10 s + relaxation delay 15 s), number of scans 128, and receiver gain adjusted automatically. The experimental time was 55 min. The UFA (170.88 ppm) and SFAs (SFA) (170.91 ppm) esterified at the sn-2 position on the TAG could be differentiated on the 13C NMR spectrum, and also the UFA (171.29 ppm) and SFA (171.32 ppm) esterified at the sn-1,3 positions. The assignment of the UFA and SFA was effected based on the inductive effect of the double bond on the carbonyl group using the method described by Vlahov et al.[Citation28] The analyses were carried out in duplicate and the average values reported with their standard deviations.
SFC
The solid fat content (SFC) was determined by nuclear magnetic resonance spectrometry using a Bruker Minispec PC 120 (Silberstreifen, Rheinstetten, Germany) together with Tcon 2000 high precision dry baths (0–70°C) (Duratech, Carmel, IN, USA). The SFC was determined according to the American Oil Chemists’ Society Official Method No. Cd 16b-93 (2009), this being a direct method with readings of the samples in series at the temperatures of 10, 20, 25, 30, 35, 40, 45, 50, 55, 60, and 65°C, with tempering for non-stabilized fats.[Citation23] In addition, it was necessary to modify the standard tempering prescribed by AOCS Method Cd 16b-93 in order to ensure stabilization of the crystallization of the blends: the samples were kept at 0°C for 2 h and at each reading temperature for 1 h. The analysis was carried out in duplicate and the average values reported with their standard deviations.
Thermal behavior
The crystallization profiles of the samples were determined in a model Q2000 DSC (TA Instruments) according to the American Oil Chemists’ Society Official Method No. Cj 1-94 (2009), weighing 8–12 mg of sample into aluminum pans and sealing them.[Citation23] The instrument was calibrated with indium (MP = 156.5°C, ΔHf = 28.45 J/g).) The samples were heated to 80°C at 10°C/min, maintained at this temperature for 10 min, and then cooled to −60°C at 10°C/min. The analysis was carried out in duplicate.
Light polarized microscopy
In order to study the morphology and the crystalline dimensions, the samples were melted at 70°C in a microwave oven. With the aid of a capillary tube, a drop of sample was placed on a glass slide preheated to the controlled temperature (70°C) and covered with a coverslip. The slides were prepared in duplicate and maintained for 24 h in an incubator at 25°C. The morphology of the crystals was evaluated using a polarized light microscope (Olympus BX 51) attached to a digital video camera (Media Cybernetics). The slides were then placed on the support of a heating plate, maintained at the same crystallization temperature. The images were captured by the Image Pro-Plus applicative (Media Cybernetics), using polarized light and an amplification of ×40. Three visual fields were focused on each slide, and the mean diameter of the crystals was selected as the evaluation parameter for the quantitative analysis of the images and calculated using the Image Pro-Plus program, version 7.0 (Media Cybernetics, Bethesda, USA).
X-ray diffraction
The samples were completely melted and crystallized at 25°C in an incubator for 24 h to stabilize fat crystallization, and the X-ray diffraction was determined according to the American Oil Chemists’ Society Official Method No. AOCS Cj 2-95 (2009).[Citation23] The measurements were made using a Philips PW 1710 diffractometer with the Bragg-Brentano (Ɵ:2Ɵ) geometry, and CuKα radiation (l = 1.5418 Å, voltage of 40 KV and current of 30 mA). The measurements were taken in 0.03° steps in 2Ɵ with an acquisition time of 2 s and scan from 14.5° to 27.5° (2Ɵ scale). The polymorphic forms were identified from the short spacings (SSs), which are characteristic of the crystals.
Statistical analysis
The data obtained for the acylglycerides composition and regionspecific distribution were submitted to an analysis of variance (ANOVA) using the STATISTICA version 8 software (StatSoft Inc., Tulsa, OK, USA). Tukey’s test was used for the means comparison with a 5% significance level (p ≤ 0.05).
Results and discussion
FA composition
shows the composition in the major FA of the raw materials and the blends (BE) with different ratios. The major FA in SO was oleic acid (C18:1) at 87.4%, followed by 5.8% of linoleic acid (C18:2) and 3.7% of palmitic acid (C16:0).[Citation1] In the FHSO, the principal FA was stearic acid (C18:0) at 96.3%, followed by 3.7% of palmitic acid (C16:0). The blends only showed four types of FA (C16:0, C18:0, C18:1, and C18:2). The different blends (BE) varied from 3.6% to 3.8% of palmitic acid (C16:0), followed by 40.4–68.2% of stearic acid (C18:0), from 26.2% to 52.4% of oleic acid (C18:1) and from 1.8% to 5.8% of linoleic acid (C18:2).
TAG composition
The TAG species in the SO, FHSO, blends, and interesterified products are presented in . The predominant TAG species in the SO were OOO (80.4%), followed by POO/OPO (11.8%), OOL/OLO (5.8%), OLL (2.1%), and PPO/POP (0.5%). The major TAG species in FHSO were SSS (88.1%) and PSS/SPS (11.9%). The main TAG species in the physical blends were OOO (35.9–61.8%) and SSS (24.2–48.7%). Both interesterification produced significant alterations in the TAG compositions of the blends. The CI is generally a random process that produces positional randomization of the acyl groups in the TAG and EI methods using a 1,3-specific lipase which have advantage as selectivity and regiospecificity of FA.[Citation22] There was a sharp decrease in OOO and SSS; the new TAG species such as SSO/SOS, SOO/OSO, and PSO/POS were detected. The main TAG species formed were SSO/SOS (20.0–38.0%) and SOO/OSO (14.4–30.0%) by CI. The results of EI were SSO/SOS (30.0–36.4%) and SOO/OSO (14.0–30.5%). A small amount of PSO/POS (4.5–5.3%) was also observed in the interesterified products, as a new species produced by both reactions. Chae et al. also observed this type of behavior for the EI of HOSO and fully hydrogenated soybean oil with Lipozyme TL IM.[Citation29] Significant decreases in the three major TAG (OOO, SSS, and PSS) were identified. Meanwhile, marked increases in the newly formed TAG species POS and SOS, as well as in SOO, were also noted.
Table 2. TAG composition (%) of high oleic sunflower (SO) and fully hydrogenated high oleic sunflower oil (FHSO), blend (BE), and after chemical (CI) and enzymatic interesterification (EI).
Acylglycerides composition
In interesterification processes, partial glycerides such as diacylglycerols (DAG) and MAG, which are related to certain reaction parameters (reaction time, reaction temperature, enzyme load, substrate ratio, and water content), are formed.[Citation30,Citation31] The values found for TAG, DAG, and MAG in the blends and in the interesterified products formed during CI and EI are shown in . Higher values were observed for DAG (8.6–9.9%) due to the enzymatic reaction as compared to the chemical reaction (7.8–9.5%). Ahmadi et al. studied the enzymatic and CI of HOSO and fully hydrogenated canola oil and also found higher DAG levels for EI as compared to CI.[Citation4]
Table 3. Acylglycerides composition (%), triacylglycerol (TAG), diacylglycerol (DAG), and monoacylglycerol (MAG) of the blends (BE) and the after chemical (CI) and enzymatic interesterification (EI).
Regiospecific distribution
The stereospecific distribution of FA in fats and oils has an effect on their nutritional and technological qualities. 13C NMR spectroscopy proved to be an effective analysis for determining the TAG structures. The methodology provided good results with minimum sample preparation and in significantly shorter experimental times than other methods. The composition and distribution of FAs between sn-1,3 and sn-2 positions of the TAG can easily be carried out and calculated by using the quantitative high-resolution 13C NMR methodology based on the carbonyl carbons of the TAG chains.[Citation32] shows the percentages of UFA and SFA present at the sn-2 and sn-1,3 positions of the glycerol before and after EI and CI. There was a decrease in UFA at position sn-2 in the physical blends (BE), due to the proportion FHSO. CI is a random reaction, showing a smaller percentage of UFA at the sn-2 position of the TAG than EI. The CI-sample had between 23.2% and 49.2% of UFA at the sn-2 position, while the EI-sample had 27.0–53.2% for all the blend ratios. The EI showed that the migration in the sn-2 position compared to the physical blends (BE); it was less than the CI. The SFA content increased at the sn-1,3 position with the proportion of FHSO for all the BE. The range of SFA in the sn-2 position was higher in CI than in EI, being, respectively, 53.6–77.7% and 51.0–74.8%. Acyl migration is a thermodynamic process and occurs spontaneously during EI using a specific lipase, resulting in a loss of selectivity.[Citation30] Several authors have studied the use of Lipozyme TL IM in IE, analyzing the acyl migration. The results showed that the specificity of the lipase can be influenced by acyl migration; long reaction times cause an increase in acyl migration.[Citation33,Citation34]
Table 4. Distribution of the fatty acids (%) at the sn-2, sn-1,3 positions on the TAG in the blend (BE), after chemical (CI) and enzymatic interesterification (EI).
SFC
SFC curve is an important parameter for analyzing many characteristics of shortenings and margarines such as appearance, spreadability, oil exudation, and others properties.[Citation35] Interesterification resulted in a decrease in SFC in all the blends with all the weight ratios. This effect is associated with a reduction in trisaturated TAG (SSS, PSS/SPS) and a simultaneous increase in the percentages of disaturated–monounsaturated (SSO/SOS, PSO/POS) and monosaturated–diunsaturated TAG (SOO/OSO, POO/OPO). The shows the SFC curves obtained for the blends, and for the interesterified fats obtained by CI and EI. There was a greater decrease in the SFC curves for all the interesterified blends after the range 20–35°C. At temperatures above this range, the SFC curves of the CI and EI were very similar, decreasing linearly until melting completely at between 60 and 65°C. For all weight ratios, the physical blends showed SFC values of 38.0–68.6% at 25°C, while the SFC values of the CI fats were 29.8–78.9% and of the EI fats 35.7–81.6% at 25°C. At 40°C, the SFC values of the blends were reduced to 29.7–61.5%, those of the SL from CI to 8.8–49.7% and those from EI to 11.3–53.7%. All the SL had 10% higher SFC at 20 and 22°C, a result that gave them the same resistance to exudation.[Citation36] All the SL showed more than 25% SFC () at room temperature (25°C). These SL were projected to have broad applications in foods, as a fatty base should be diluted with small proportions of liquid oil in order to adjust its physical properties for shortenings and as a crystallization additive for lipids.
Figure 1. Solid fat content (%) of high oleic sunflower (SO) and fully hydrogenated high oleic sunflower oil (FHSO) blend (BE) (▲), after chemical (CI) (x) and enzymatic interesterification (EI) (■).
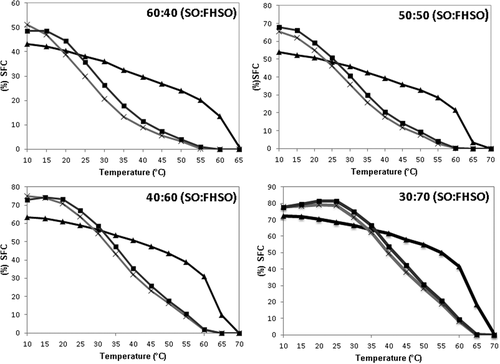
Crystallization behavior
The thermal behavior of the fats can be studied using DSC. The DSC thermal profiles can be used to monitor the EI reaction and to explain the change in the physical properties of the interesterified products.[Citation37] The shapes of the curves were modified by the reaction, reflecting alterations in the TAG compositions. The shows the crystallization profiles of the BE, EI, and CI of the SO:FHSO ratios. The physical blends (BE) showed a pronounced peak, which characterizes the trisaturated fraction (SSS) of the FHSO with a high crystallization point. The crystallization temperatures of the blends increased with increase in the FHSO content; therefore, the crystallization process was accelerated. The crystallization onset temperatures ranged from 44.5 to 49.1°C. The second peak showed a smaller intensity of the triunsaturated fraction (OOO) of the SO, with a low crystallization point from −14.5 to −16.6°C. Similar results were observed in a study of EI from HOSO and tripalmitin or tristearin.[Citation21] After interesterification (EI and CI) were produced other types of intermediary TAG, changing the crystallization profiles. In the interesterified products from the blends at 60:40 and 50:50 (SO:FHSO), three peaks were obtained and two peaks to 40:60 and 30:70 for the crystallization behavior, with similar crystallization temperatures for EI and CI. These second peaks had a range of crystallization onset temperatures from 16.5 to 22.9°C. There was a higher intensity of the peak of intermediate TAGs, such as monounsaturated-disaturated (SSO/SOS) TAG and diunsaturated-monosaturated (SOO/OSO) TAG. This type of behavior was also observed by Ribeiro et al. in the interesterification of blends from soybean oil and fully hydrogenated soybean oil.[Citation38]
Microstructure
Light polarized microscopy is the technique to visualize most used the microstructural network of fats in order to explain the differences in texture and crystals of these materials, and the morphological changes that occurred during the growth of these crystals. This technique also showed changes in the polymorphic forms, resulting from interesterification of the fats.[Citation39] shows the SO:FHSO blends before and after interesterification crystalline structures being obtained by crystallization and cooling at 25°C/24 h, with a magnification of ×40. The formation of spherulites was observed in the physical blends (BE), being larger for the 40:60 and 30:70 blends (OS:FHSO) due to the higher proportion of tristearin (SSS). The presence of large spherulites or spherulite clusters gives the fat an undesirable grainy texture and is mainly due to the tristearin content of the blends.[Citation40] It was observed that the crystal diameter decreased with increasing SO content in the blends. Enzymatic and CI altered the crystal morphology of all the blends. After interesterification, less dense spherulites crystals were formed, closely packed structures being observed in the 40:60 and 30:70 blends (SO:FHSO) as compared to the physical blends. However, after interesterification, the 50:50 and 60:40 blends (SO:FHSO) showed the formation of highly dense spherulites crystals with a loosely packed structure. The interesterification of blends of canola oil and fully hydrogenated cottonseed oil caused a decrease in crystal size.[Citation41] The differences in the crystal structures can be significantly influenced by interactions between the molecules in the crystalline state and the amount of liquid oil around the crystals, factors that would promote differences at many different structural levels, from the formation of crystals and aggregates up to the formation of floccules.[Citation42] The EI and CI processes decreased the mean diameter of the crystals, but simultaneously, an increase in FHSO concentration of the blends resulted in an increase in the mean diameter of the crystals for both the EI and CI processes. The range of mean diameter crystal obtained with EI and CI was between 18.5 and 22.7 µm. The mean diameter of the fats crystals should be less than 30 µm to prevent a feeling of grittiness in the mouth.[Citation43]
Polymorphism
Polymorphic forms of fats are solid phases of the same chemical composition which on melting yield identical liquid phases. The α form is metastable and will thus transform into more stable forms. Two crystalline forms are called “monotropic” when one is more stable than the other, and recrystallization will only take place in the direction of the more stable form. The three polymorphic (α, β′, and β) forms can form directly from the melt. The irreversibility of the α to β′ to β transformation is also evident.[Citation44] X-ray diffraction is commonly used to differentiate between lipid polymorphs. The SSs represent the distance between adjacent planes in a crystalline arrangement.[Citation45] shows the X-ray diffraction pattern with the SS and polymorphic forms of the 50:50 and 30:70 (SO:FHSO) physical blends (BE) and fats (EI and CI) interesterified at 25°C for 24 h. The crystals that exhibited polymorphic form β′ have an orthorhombic structure and are characterized in the diffraction X-ray analysis by SSs near 3.89 and 4.20 Å, while the polymorphic form β, with a triclinic structure, usually presents an interplanar spacing feature at 4.6 Å.[Citation46] The physical blends exhibited a mixture of β (strong or medium intensity) and β′ (medium intensity) forms, whereas after interesterification in the 30:70 blend (CI and EI), the intensity of the SS representing the β form appeared to be diminished, and the β′ crystal form was more dominant than the physical blend. For the 50:50 blend, after interesterification (CI and EI), a mixture of the β (strong intensity) and β′ (medium and weak intensity) forms continued to be present. According to the results, the β′ polymorph is associated with an increase of FHSO in the blend. Diffractograms of the blends before (BE) and after interesterification (EI and CI) at 25°C for 24 h are shown in . The β′ crystals are small and their morphology is appropriate for their plasticity characteristics, stabilizing the air in fats for bakery and confectionary products. The β form comprises large crystals that give hardness so that they may be used as a crystallization additive for lipids. Previous studies reported similar results.[Citation17,Citation47]
Table 5. Polymorphic forms of the blends before (BE) and the after chemical (CI) and enzymatic interesterification (EI), obtained at 25°C for 24 h.
Conclusion
In conclusion, the enzymatic process showed advantages over CI with respect to the low reaction temperature and selectivity in the formation of new TAG. The enzymatic process with 3 h of reaction time showed less acyl migration in the sn-2 position of UFA, as evidenced by 13C NMR. The SL obtained displayed suitable characteristics for application as a fatty base for shortenings and as a crystallization additive for lipids.
References
- Alberio, C.; Izquierdo, N. G.; Galella, T.; Zuil, S.; Reid, R.; Andrés Zambelli, A.; Aguirrezábal, L. A. N. A New Sunflower High Oleic Mutation Confers Stable Oil Grain Fatty Acid Composition across Environments. European Journal of Agronomy 2016, 73, 25–33. DOI: 10.1016/j.eja.2015.10.003.
- Osborn, H. T.; Akoh, C. C. Structured Lipids-Novel Fats with Medical, Nutraceutical and Food Applications. Comprehensive Reviews in Food Science and Food Safety 2002, 3, 93−103.
- Iwasaki, Y.; Yamane, T. Enzymatic Synthesis of Structured Lipids. Journal of Molecular Catalysis B: Enzymatic 2000, 10, 129–140. DOI: 10.1016/S1381-1177(00)00120-X.
- Ahmadi, L.; Wright, A. J.; Marangoni, A. G. Chemical and Enzymatic Interesterification of Tristearin/Triolein-Rich Blends: Chemical Composition, Solid Fat Content and Thermal Properties. European Journal of Lipid Science and Technology 2008, 110, 1014–1024. DOI: 10.1002/ejlt.v110:11.
- Farfán, M.; Álvarez, A.; Garate, A.; Bouchon, P. Comparison of Chemical and Enzymatic Interesterification of Fully Hydrogenated Soybean Oil and Walnut Oil to Produce a Fat Base with Adequate Nutritional and Physical Characteristics. Food Technology and Biotechnology 2015, 53, 361–366. DOI: 10.17113/ftb.53.03.15.3854.
- Akoh, C. C.;. Making New Structured Fats by Chemical Reaction and Enzymatic Modification. Lipid Technology 1997, 5, 61–66.
- Berry, S. E. E.;. Triacylglycerol Structure and Interesterification of Palmitic and Stearic Acid-Rich Fats: An Overview and Implications for Cardiovascular Disease. Nutrition Research Reviews 2009, 22, 3–17. DOI: 10.1017/S0954422409369267.
- Garima, P.; Sabir, J. S. M.; Baeshen, N. A.; Akoh, C. C. Enzymatic Synthesis of Extra Virgin Olive Oil Based Infant Formula Fat Analogues Containing ARA and DHA: One-Stage and Two-Stage Syntheses. Journal of Agricultural and Food Chemistry 2013, 61, 10590−10598.
- Svensson, J.; Adlercreutz, P. Effect of Acyl Migration in Lipozyme TL IM-catalyzed Interesterification Using a Triacylglycerol Model System. European Journal of Lipid Science and Technology 2011, 113, 1258–1265. DOI: 10.1002/ejlt.201100097.
- Pacheco, C.; Crapiste, G. H.; Carrín, M. E. Study of Acyl Migration during Enzymatic Interesterification of Liquid and Fully Hydrogenated Soybean Oil. Journal of Molecular Catalysis B: Enzymatic 2015, 122, 117–124. DOI: 10.1016/j.molcatb.2015.08.023.
- Brys, J.; Wirkowska, M.; Gorska, A.; Ostrowska-Ligeza, E.; Brys, A. Application of the Calorimetric and Spectroscopic Methods in Analytical Evaluation of the Human Milk Fat Substitutes. Journal of Thermal Analysis and Calorimetry 2014, 118, 841–848. DOI: 10.1007/s10973-014-3893-1.
- Zou, X. Q.; Huang, J. H.; Jin, Q. Z.; Guo, Z.; Cheong, L.; Xu, X.; Wang, X. Preparation of Human Milk Fat Substitutes from Lard by Lipase-Catalyzed Interesterification Based on Triacylglycerol Profiles. The Journal of the American Oil Chemists’ Society 2014, 91, 1987–1998.
- Kim, S.; Kim, I. H.; Ako, C. C.; Kim, B. H. Enzymatic Production of Cocoa Butter Equivalents High in 1-Palmitoyl-2-Oleoyl-3-Stearin in Continuous Packed Bed Reactors. The Journal of the American Oil Chemists’ Society 2014, 91, 747–757. DOI: 10.1007/s11746-014-2412-7.
- Ruan, X.; Zhu, X. M.; Xiong, H.; Wang, S. Q.; Bai, C. Q.; Zhao, Q. Characterisation of Zero-Transmargarine Fats Produced from Camellia Seed Oil, Palm Stearin and Coconut Oil Using Enzymatic Interesterification Strategy. International Journal of Food Science & Technology 2014, 49, 91–97. DOI: 10.1111/ijfs.12279.
- Omar, K. A.; Gounga, M. E.; Liu, R.; Mwinyi, W.; Aboshora, W.; Ramadhan, A. H.; Khadija Sheha, A. Triacylglycerol Composition, Melting and Crystallization Profiles Lipase Catalyzed Hydrolysis of Anhydrous Milk Fats. International Journal of Food Properties 2017, 1–16. DOI: 10.1080/10942912.2017.1301954.
- Yu, D.; Qi, X.; Ren, Y.; Wang, W.; Suna, L.; Xu, D.; Zhang, H.; Hu, L.; Jiang, L.; Elfalleh, W. Thermal and Crystal Characteristics of Enzymatically Interesterified Fats of Fatty Acid-Balanced Oil and Fully Hydrogenated Soybean Oil in Supercritical CO2 System. International Journal of Food Properties 2016, 1–11. DOI: 10.1080/10942912.2016.1248846.
- Zhao, S. Q.; Hu, J. N.; Zhu, X. M.; Bai, C. Q.; Peng, H. L.; Xiong, H.; Hu, J. W.; Zhao, Q. Characteristics and Feasibility of Trans-Free Plastic Fats through Lipozyme TL IM Catalyzed Interesterification of Palm Stearin and Akebia Trifoliata Variety Australis Seed Oil. Journal of Agricultural and Food Chemistry 2014, 62, 3293–3300. DOI: 10.1021/jf500267e.
- Fernandez-Lafuente, R.;. Lipase from Thermomyces Lanuginosus: Uses and Prospects as an Industrial Biocatalyst. Molecular Catalysis B: Enzymatic 2010, 62, 197−212.
- Rodrigues, R. C.; Ortiz, C.; Berenguer-Murcia, A.; Torres, R.; Fernandez-Lafuente, R. Modifying Enzyme Activity and Selectivity by Immobilization. Chemical Society Reviews 2013, 42, 6290—6307. DOI: 10.1039/C2CS35231A.
- Lee, Y. Y.; Tang, T. K.; Phuah, E. T.; Karim, N. A. A.; Alwi, S. M. M.; Lai, O. M. Palm-Based Medium-And-Long-Chain Triacylglycerol (P-MLCT): Production via Enzymatic Interesterification and Optimization Using Response Surface Methodology (RSM). Journal of Food Science and Technology 2015, 52, 685–696. DOI: 10.1007/s13197-013-1065-0.
- Ifeduba, E. A.; Martini, S.; Akoh, C. C. Enzymatic Interesterification of High Oleic Sunflower Oil and Tripalmitin or Tristearin. Journal of the American Oil Chemists’ Society 2016, 93, 61–67. DOI: 10.1007/s11746-015-2756-7.
- Ribeiro, M. D. M. M.; Ming, C. C.; Silvestre, I. M.; Grimaldi, R.; Gonçalves, L. A. G. Comparison between Enzymatic and Chemical Interesterification of High Oleic Sunflower Oil and Fully Hydrogenated Soybean Oil. European Journal of Lipid Science and Technology 2017, 119, 1–9.
- [AOCS] American Oil Chemist’s Society. Official and Recommended Methods of the American Oil Chemist’s Society; 15th. AOCS Press: Champaign, IL, 2009.
- Grimaldi, R.; Gonçalves, L. A. G.; Ando, M. Y. Otimização Da Reação De Interesterificação Química De Óleo De Palma. Química Nova 2005, 28, 633–636. DOI: 10.1590/S0100-40422005000400015.
- Hartman, L.; Lago, R. C. A. Rapid Preparation of Fatty Acid Methyl Esters from Lipids. Laboratory Practice 1973, 22, 475–494.
- Antoniosi Filho, N.; Mendes, O. L.; Lanças, F. M. Computer Predition of Triacylglycerol Composition of Vegetable Oils by HRGC. Chromatographia 1995, 40, 557–562. DOI: 10.1007/BF02290268.
- Dobarganes, M. C.; Velasco, J.; Dieffenbacher, A. Determination of Polar Compounds, Polymerized and Oxidized Triacylglycerols, and Diacylglycerols in Oils and Fats. Pure and Applied Chemistry 2000, 72, 1563–1575. DOI: 10.1351/pac200072081563.
- Vlahov, G.; Giuliani, A. A.; Del, R. P. 13C NMR Spectroscopy for Determining the Acylglycerol Positional Composition of Lampante Olive Oils. Chemical Shift Assignments and Their Dependence on Sample Concentration. Analytical method 2010, 2, 916–923. DOI: 10.1039/c0ay00028k.
- Chae, M. H.; Park, H. K.; Kwon, K. I.; Kim, J. W.; Hong, S. I.; Kim, Y.; Kim, B. H.; Kim, I. H. Lipase-Catalyzed Interesterification in Packed Bed Reactor Using 2 Different Temperatures. Journal of Food Science 2011, 76, 555–559. DOI: 10.1111/j.1750-3841.2011.02115.x.
- Xu, X.; Skands, A. R. H.; Hoy, C. E.; Mu, H.; Balchen, S.; Nissen, J. A. Production of Specific-Structured Lipids by Enzymatic Interesterification: Elucidation of Acyl Migration. The Journal of the American Oil Chemists' Society 1998, 9, 1179–1185. DOI: 10.1007/s11746-998-0132-6.
- Kadivar, S.; Clercq, N.; Van deWalle, D.; Dewettinck, K. Optimisation of Enzymatic Synthesis of Cocoa Butter Equivalent from High Oleic Sunflower Oil. Journal of the Science of Food and Agriculture 2014, 94, 1325–1331. DOI: 10.1002/jsfa.2014.94.issue-7.
- Lopes, T. I. B.; Ribeiro, M. D. M. M.; Ming, C. C.; Grimaldi, R.; Gonçalves, L. A. G.; Marsaioli, A. J. Comparison of Regiospecific Distribution from Triacylglycerol after Chemical and Enzymatic Interesterification of Sunflower Oil and Sunflower Hydrogenated Oil Blend by Carbon-13 Nuclear Magnetic Resonance. Food Chemistry 2016, 212, 641–647. DOI: 10.1016/j.foodchem.2016.06.024.
- Teichert, S.; Akoh, C. C. Characterization of Stearidonic Acid Soybean Oil Enriched with Palmitic Acid Produced by Solvent-Free Enzymatic Interesterification. Journal of Agricultural and Food Chemistry 2011, 59, 9588–9595. DOI: 10.1021/jf201992k.
- Kim, I.-H.; Ko, S.-N.; Lee, S.-M.; Chung, S.-H.; Kim, H.; Lee, K. T.; Ha, T. Y. Production of Structured Lipids by Lipase-Catalyzed Acidolysis in Supercritical Carbon Dioxide: Effect on Acyl Migration. The Journal of the American Oil Chemists' Society 2004, 81, 537–541. DOI: 10.1007/s11746-006-0937-0.
- Lida, A. M. D. N.; Sundram, K.; Siew, W. L.; Aminah, A.; Mamot, S. TAG Composition and Solid Fat Content of Palm Oil, Sunflower Oil and Palm Kernel Olein Blends before and after Chemical Interesterification. The Journal of the American Oil Chemists’ Society 2002, 79, 1138–1143.
- Guedes, A. M. M.; Ming, C. C.; Ribeiro, A. P. B.; Silva, R. C.; Gioielli, L. A.; Gonçalves, L. A. G. Physicochemical Properties of Interesterified Blends of Fully Hydrogenated Crambe Abyssinica Oil and Soybean Oil. The Journal of the American Oil Chemists' Society 2014, 91, 111–123. DOI: 10.1007/s11746-013-2360-7.
- Danthine, S.; Clercq, N. D.; Dewettinck, K.; Gibon, V. Monitoring Batch Lipase Catalyzed Interesterification of Palm Oil and Fractions by Differential Scanning Calorimetry. Journal of Thermal Analysis and Calorimetry 2014, 115, 2219–2229. DOI: 10.1007/s10973-014-3645-2.
- Ribeiro, A. P. B.; Grimaldi, R.; Gioielli, L. A.; Santos, A. O.; Cardoso, L. P.; Gonçalves, L. A. G. Thermal Behavior, Microstructure, Polymorphism, and Crystallization Properties of Zero Trans Fats from Soybean Oil and Fully Hydrogenated Soybean Oil. Food Biophysics 2009, 4, 106–118. DOI: 10.1007/s11483-009-9106-y.
- Rousseau, D. R.; Marangoni, A. G. The Effects of Interesterification on the Physical Properties of Fats. In Physical Properties of Lipids; Marangoni, A. G., Narine, S., Eds; CRC Press: New York, USA, 2002.
- Narine, S. S.; Humphrey, K. L. A Comparison of Lipid Shortening Functionality as a Function of Molecular Ensemble and Shear: Microstructure, Polymorphism, Solid Fat Content and Texture. Food Research International 2004, 37, 28–38. DOI: 10.1016/j.foodres.2003.09.013.
- Ribeiro, A. P. B.; Basso, R. C.; Grimaldi, R.; Gioielli, L. A.; Santos, A. O.; Cardoso, L. P.; Gonçalves, L. A. G. Influence of Chemical Interesterification on Thermal Behavior, Microstructure, Polymorphism and Crystallization Properties of Canola Oil and Fully Hydrogenated Cottonseed Oil Blends. Food Research International 2009, 42, 1153–1162. DOI: 10.1016/j.foodres.2009.05.016.
- Shi, Y.; Liang, B.; Hartel, R. W. Crystal Morphology, Microstructure and Textural Properties of Model Lipid Systems. The Journal of the American Oil Chemists' Society 2005, 82, 399–408. DOI: 10.1007/s11746-005-1084-3.
- Marangoni, A. G.; Narine, S. S. Identifying Key Structural Indicators of Mechanical Strength in Networks of Fat Crystals. Food Research International 2002, 35, 957–969. DOI: 10.1016/S0963-9969(02)00158-8.
- Marangoni, A. G.; Wesdorp, L. H. Crystallography and Polymorphism. In Structure and Properties of Fat Crystal Networks; 2 rd. CRC Press Taylor & Francis: Boca Raton, FL, 2013; pp. 1–24.
- Omar, Z.; Let, C. C.; Seng, C. C.; Rashid, N. A. Crystallization and Rheological Properties of Hydrogenated Palm Oil and Palm Oil Blends in Relation to Crystal Network. European Journal of Lipid Science and Technology 2005, 107, 634–640. DOI: 10.1002/ejlt.200501180.
- Hartel, R. W.;. Cristallization in Foods; Aspen Publication: Maryland, 2001.
- Lee, J. H.; Akoh, C. C.; Lee, K. T. Physical Properties of Trans-Free Bakery Shortening Produced by Lipase-Catalyzed Interesterification. The Journal of the American Oil Chemists’ Society 2008, 85, 1–11. DOI: 10.1007/s11746-007-1155-0.