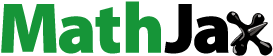
ABSTRACT
This paper presents a study of the processing of collagen-containing raw material and its changes in the course of targeted complex processing by hydrolysis, including freeze-drying. The pH, chemical composition, penetration magnitude, and critical shear stress were determined. The dried samples were examined using Fourier-transform infrared spectroscopy, and their microstructures were characterized. The characteristic property of the product developed was determined to be the presence of a relatively homogeneous fibrillar structure that promotes the functional capacity of the proteinoid-forming fibres in the compositions of foods from different groups.
Introduction
The achievements of biotechnology and its increasing application in food production allow not only expanded assortments but also compliances with adequate and functional nutrition. Because of the current economic situation, enterprises that produce food products from raw materials of animal origin are seeking non-traditional sources of raw materials, namely, wastes obtained in animal-skin processing, boning, and desinewing. [1,2] Researchers are developing protein-containing products and, specifically, fat substitutes for meat products. [3–5] This research focuses on a related problem: derivatives of animal skin are known to have a heterogeneous structure with all tissue classes (connective, epithelial, smooth muscle, and nervous) represented.
The skin of agricultural animals, especially pigskin, contains differentiated main matrix substances, including cells, hog hair, follicles, and accompanying micro-components, that not only determine the rheological properties of the raw material but also correlate with the strength properties of the fibres and the capillary-porous structure of the matrix under investigation. The formation of raw material capillaries is associated with various structures, e.g., lipocytes, which form fibrous bands that run along the entire thickness of the raw material. Therefore, processing should be aimed at removing excess fat.
The purpose of the research was to develop technological solutions and recipes for jelly products and meat farce systems based on the identification of individual technological processes, including the alkaline-salt preparation of collagen-containing raw materials, which involves purification from unwanted fractions (fat and keratin derivatives), freeze-drying, and other effects on the structures and properties of the raw material.
Materials and methods
At the research stage, technological solutions that were previously developed were applied, including methods [6–8] involving the controlled hydrolysis of the protein product samples. The skin samples obtained from the pig carcasses were the objects of investigation. The samples (25 × 25 mm) were cut from two symmetrical portions of a pig skin.
These samples were treated in solutions of chemically pure sodium hydroxide (food additive E524) in sodium chloride (high-grade cooking salt) with alkali concentrations of 3% (solution pH = 12.13) protein module PM-3 sample; 5% (pH = 12.59) – PM-5 sample; and 7% (pH = 12.77) – PM-7 sample (). To produce a protein product, hydrolytic treatment of raw materials was performed using an alkaline-salt method. [8] The modification of pigskins enables several successive operations. The raw material was frozen and crushed into 25 × 25 mm2 pieces in special devices and immersed in an alkaline-saline solution with sodium hydroxide concentrations of 3, 5, and 7% as well as a sodium chloride concentration of 6%. During the alkaline-salt treatment, the raw materials were thawed and dehaired and the structure was dispersed. The alkaline salt treatment lasted 24 h. Next, the raw material was subjected to salt washing with a solution of 6% sodium chloride for 2 h. The liquid coefficient in the alkaline-salt treatment step was 2.5. The samples were neutralized with a 7% acetic acid food solution (index E260) to the pH values indicated in . This acetic acid method is one of the best ways to disperse and dissolve fibrillar proteins of connective tissue. The raw material was washed with water, and the excess moisture was forced out. The control raw material was not hydrolytically modified. In the infrared (IR) spectral analysis, the results were compared to those for a gelatin control sample.
Table 1. Changes in physicochemical parameters of the raw material in processing.
In the NaCl + NaCl solution, there was a loosening of the bundles of collagen fibres and dissolution of the organic substances of the keratin structures, which improved the sensory perception of products as a result of heat treatment. In addition, the effects of various salts on the structures of type I collagen, which allows them to be dispersed and extracted directly, are known. [9]
At the solution concentrations indicated, a fixation in the level of hydroxyl ions was observed, and no significant pH changes were registered with the alkali concentration increase. The 6% NaCl concentration was fixed, and the destruction of proteinoid structures is usually contained. The liquid coefficient during alkaline-salt treatment was 2.5. The samples were neutralized with an edible acetic acid solution to the pH values given in . A specific NaOH action is protein deamination, in which the basic electrolyte affects the change in the structure and properties of collagen, causing a strong anisotropic swelling, accompanied by moisture absorption and an increase in sample weight. The alkali effect promotes the following transformations in collagen fibrils: interactions between the amine and carboxyl protein groups; disruption of hydrogen intra- and interchain bonds; the destruction of covalent interchain bonds in the primary structure with the formation of free –C– and –N– end groups; and the destruction of intra- and intermolecular covalent bonds in the tertiary and quaternary structures. The mechanisms of these transformations comprise the dissolution of collagen fibrils under the influence of alkali caused by the rupture of hydrogen bonds with the exothermic effect. When a similar excess (positive or negative) charge occurs, this leads to electrolytic repulsive forces, and the biopolymer structure is loosened. The polypeptide chains move apart and are penetrated by water molecules, leading to an increase in water cut (collagen filaments thicken and decrease in length). Among the main advantages of the developed collagen modification method, which is intended for the rational use of available raw materials of animal origin to widen the field of their application, it is worth highlighting its simplicity, its low cost (attributable to lower consumption of reagents and temperature), and its standard technological process for the production of meat and meat products. This method allows the handling of substandard pigskins, which are obtained when pigs are slaughtered in a native state and not dehaired at meat-processing plants or slaughter stations. In addition, such treatment does not lead to the formation of chemical compounds in the structure of the raw material, which can adversely affect the living organism, since inorganic compounds permitted by the health authorities in the Russian Federation as additives in the food industry are used in alkaline-salt treatment, neutralization, and salt washing. The use of a hydrochloric acid solution to neutralize sodium hydroxide leads to the formation of only sodium chloride (which is evenly distributed in the interstructural space of the dermis) and water. Consequently, the complex hydrolytic effect accelerates the process of jelly formation on a meat and protein basis.
The chemical composition, pH, and rheological properties of samples were determined. The hydrolytic processing of the raw material to produce a protein product was performed using a patent-protected alkaline-salt procedure. [7,8]
At the end of the hydrolytic treatment and neutralization, the samples were subjected to vacuum freezes using the СВП-0.36 research bench, [10] which was developed at the Moscow State University of Applied Biotechnology/Moscow State University of Food Production and was intended for the study of the vacuum dehydration processes of a wide range of thermolabile materials, including raw meat materials. The principal features of the bench are its vacuum and cooling systems. The first allows traditional vacuum freeze-drying through the “ice-steam” phase transition, and the second enables dewatering in the vacuum evaporation mode. A view of the bench and raw material samples is presented in (). The drying parameters were chosen using the following prerequisites. From the freeze-drying, high-quality thermolabile materials were achieved when 75–90% of moisture was removed by the ice-steam phase transition. At the same time, as the sublimation temperature decreased, the quality of the freeze-dried thermolabile materials increased, but the specific energy consumption for drying increased proportionally.
In the obtained samples, the chemical composition, pH, and rheological properties were determined. Moisture content was determined using a gravimetric method (drying in a laboratory drying oven at a temperature of 100 ± 5°C). A semi-automatic “Kjeltec” (“Tecator,” Sweden) analyser was used to determine the protein (determination of total nitrogen in the mineralized sample) content. The Soxhlet method uses multiple extractions with a hot solvent (chemically pure CCl4) in a standard glass instrument to determine fat content. The pH was determined using a “pH-420” (Aquilon, Russia) pH meter with a pH range of –0.5 – 14.
Microstructural analysis was performed according to the methods of Sarkisov and Perov [11] and Pchelkina and Khvilya [12], using a C. Zeiss AxioImager research microscope with photo and video systems to study the histological images. For scanning electron microscopy, a high-resolution FEI VERSA 3D (Netherlands) instrument with a computer image analysis system (“Systems for Microscopy and Analysis” company) was used.
The penetration degree was measured with a semi-automatic PN 10 penetrometer with a cone indentor weighing 70 g and an angle of 2 α 60 degrees, and the values were recalculated as ultimate shear stress according to the Rebinder formula (1):
where m is the mass of the cone indenter, kg; k is a coefficient that considers the geometry of the indenter; and h is the penetration degree, m. The dried samples were examined using Fourier-transform infrared spectroscopy [13] using the ALPHA Fourier-IR spectrometer (Bruker) with a single attenuated total internal reflection module with a diamond crystal designed for universal basic spectral analysis in the mean IR range from 375 to 7500 cm−1. The preparation of samples for IR spectroscopy was minimal: the protein modules (PMs) powder samples were applied to a diamond crystal of the interferometer and fixed with a clamping device; the spectrum was obtained in an automated mode. The RockSolid interferometer ensured stable and accurate results and did not require adjustment. The OPUS/Mentor specialized software (Bruker, Germany) complied with the GLP (good laboratory practice) and GMP (good manufacturing practice) standards and guided the user in the analysis from measuring spectra to evaluating their results, including the identification and (quantitative) analysis of single components in the mixture spectrum and visualization of the spectra coincidence with those in the data libraries of the food components and additives.
The test technology and recipe were standard [ here] and involved cooking of the raw meat materials (beef) for 3–3.5 h. One hour before the meat raw material was cooked, carrots, onions, and spices were added according to the recipe. The cooked meat was removed from the container and ground in a meat grinder. The minced meat was combined with broth, salted (2% sodium chloride), brought to a boil, and stirred. Upon grinding in the meat grinder, a rehydrated PM based on the collagen-containing raw material and prepared according to published methods [7,8] was added.
Table 2. Test jelly formulations.
Results and discussion
Physicochemical and rheological changes in raw materials during hydrolytic treatment
The raw material studied had a pH of 5.94, making it suitable for food and culinary production. The raw material penetration by the ПН-10 apparatus revealed average values of 29.6 × 10–1 mm (16.8 kPa) that could be compared at various processing stages in a protein product (module). The individual physicochemical parameters at different stages of processing are presented in .
According to , there were differences in the samples according to their rheological parameters, i.e., the penetration degree and critical shear stress caused by differences in hydration properties and substrate and moisture interactions under the swelling principle. For other parameters, the differences were less significant. The data in show the results of the raw animal material conversion, including pH. Because of the alkaline-salt dispersion and neutralization, the pH is weakly acidic (5–5.6), eliminates microbiological risks, and corresponds to the range of acidity for raw animal materials. The degrees of penetration and the shear stress values varied substantially, but decreasing rheological indices were observed, which assisted in loosening the solid structure of the collagen proteinoids. The processing of collagen-containing raw materials included freeze drying, which yielded a PM with a residual moisture content of 1.5–2% and contributed to the production of semi-finished products that are more desirable by enterprises and provide a maximum prolongation of shelf life. Because the properties of raw materials often change, the rheological parameters of the PM produced have been studied for scientific purposes but can also be used for the lot-size control of semi-finished products in production.
According to current food production regulations, the PM obtained should be stored in a cooled state, which limits its use to no more than 14 days. To ensure long-term unregulated temperature conditions and to enable the grinding of samples to a powdered state that extended the technological possibilities for their use in various formulations, we vacuum freeze-dried the PM samples.
At various freezing temperatures (tfr, °C), the frozen moisture amounts (ω, %) in the samples under study were calculated using Eq. (1):
The cryoscopic temperature (tcr) included in Eq. (1) was determined experimentally, and its values for the samples studied amounted to −1.4 to −1.6°C. In accordance with the calculations in Eq. (1), sublimation temperatures of −20, −30, and −35°C were used in the vacuum freeze-drying experiments. The peak heating temperature was chosen to preserve the thermal stability of the samples under study and was 37 ± 2°C in all experiments. The samples were primarily fibrillar proteins, namely, collagen, whose molecules were characterized by a denaturation temperature of approximately 43°C and a fibre-melting temperature of approximately 65°C.
In the experiments, the material was dried to the final moisture content of 1.5–2%. The freeze-drying resulted in PMs that are convenient for storage and subsequent use. The terms of storage were not less than one year. When used in meat production, preliminary rehydration performed in a short time can be used.
For the next step of the research study, the influence of sublimation temperature on the complex of quality parameters of the dried products was evaluated. The rational level was the sublimation temperature at −20°C. The regime parameters (sublimation temperature of −20 ± 2°C and heating temperature of 37 ± 2°C) may be fully implemented in industrial freeze-drying units.
The preliminary freezing and subsequent vacuum dewatering using the “ice-steam” phase transition, which was used during freeze-drying, inevitably led to changes in the structure of the capillaries and fibres. These changes had a decisive effect on the rehydration of the dried samples and the preservation of protein structure. [14] Since these parameters were essential for the quality of the dried modules, we performed microstructure analysis according to these methods. [11,12]
Effect of vacuum freeze-drying on structure and properties of PMs
A solid “fixed” capillary-porous structure of xerogel-type samples resulted from freeze-drying. The critical shear stress sharply increased after drying [ here]. The architectonics of the fibrils were generally preserved, but small deformations of the collagen fibre fascicles and the emergence of pores and vacuoles accompanied by generally tight arrangements of microsections were observed.
Since one of the tasks was to justify the possibility of including vacuum freeze-drying into the raw material processing to form specific microdisperse the food systems, the structure of the semi-finished products was studied after this step [ here].
Figure 2. Microstructure of the control samples of raw material, lens × 20 (A and B); × 40 (C); the PM microstructure after hydrolytic treatment, lens × 20 (D and E); × 40 (F); the PM microstructure after freeze-drying (G and H are different layers), lens × 20.
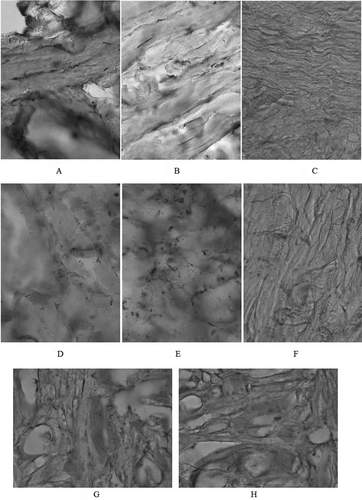
In particular, there were positive changes in the raw material caused by the preparation of the raw material for further processing into foodstuffs, namely, enhanced lyophilic properties, fat extraction, and bleaching attributable to the combination of the impacts of hydrolytic compounds, which can be used as food additives by regulating the pH.
Visual control and microstructural analysis showed the destruction of the hog-hair derivatives [ and here] to give relatively homogeneous samples of a semi-finished product, which, in the low-fat and dried state, could serve as a PM for use in industry.
In the control sample [ and here], there were large dense fascicles of tangential collagen fibres (FCF); in some parts, a capillary-porous structure of the tissue was visualized. The tinctorial properties of the sections were relatively pronounced. At high magnification [ here], fragmentary elastin fibres were observed, whose direction almost coincided with that of the FCF, along with various subcutaneous fat layer structures, including lipocytes, which loosened the tissue architectonics.
Visual inspection and microstructural analysis indicated the hydrolytic destruction of hog hair derivatives (, D, E, and F), which led to the production of relatively homogeneous samples of semi-finished product, which in a low-fat, dried state can serve as a PM applicable to industry.
Photomicrographs of samples after hydrolytic treatment are shown in (D, E, and F). The section arrangement was changed; in particular, the fibre fascicles swelled and aggregated to contribute to the thermotropic gelation during heat treatment.
Deformations, loosening, and hydration were apparently observed for collagen and elastin fibres that were positively correlated with a more homogeneous texture. However, it was more appropriate to confirm these changes using a special histochemical method. [11] Hydrolytic changes were indicated by preparations with less saturated colour.
Swelling and differentiation of the FCF led to loss of some initial features, and the dispersion of certain fibre fascicles were revealed. The intervals between the FCF almost disappeared, which was assumed to improve the texture, including the monolithic nature of the food materials being developed.
The revealed features of the microstructure demonstrated the preservation of the fibrillar structure. The presence of capillaries could promote the rapid rehydration of samples. If necessary, these materials can be ground to a powdery or fibre-like state for more efficient uses as an ingredient, i.e., as a fibre source.
The PMs, which are convenient for long-term storage, resulted from freeze-drying. To reveal the potential of biopolymers and dietary fibre, the possibility of combining them with other ingredients in food products and of predicting the quality of the meat products containing a PM developed according to the technologies proposed, the changes at the level of the molecular structure were studied using infrared spectroscopy with an ALPHA-P Fourier-IR spectrometer.
IR spectral analysis [ and here] established that according to the spectrometer database in the computer, the samples studied (control, PM-3, PM-5, and PM-7) corresponded to skin proteinoids and the control to gelatin proteins. The characteristic peaks for the valence and deformation vibrations of various groups, i.e., ОН, СНn, >С=O, ethereal, and others, can be detected in the infrared spectra. The IR spectra obtained for the control and PM (for example, PM-5) were similar, but the peak altitudes varied. Therefore, taking into account the specificity of the raw material, it was expedient to compare the results to those of known protein structures.
Figure 3. Infrared absorption spectrum: A – the control sample; B – the PM-5 sample; C – the edible gelatin imported.
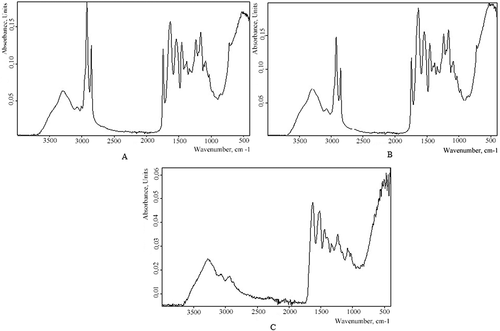
The IR spectra of the polypeptides, the proteinoids, and the products of their modification are known to contain several intense absorption bands. The changes associated with the vibrations of the amide CONH-groups, which were common structural elements of the proteinoid molecules, were revealed. There are nine recognized bands, including the amide A (absorption band ≈ 3300 cm−1), amide B (≈3100 cm−1), amide I (1600–1700 cm−1), and amide II (≈ 1500–1600 cm−1) bands. The corresponding absorption peaks of the infrared radiation were observed in these ranges of wave numbers in the PM samples. However, with intensified raw material treatment, a shift of some peaks to the right was observed for some samples [ and here].
The amide I region (1600–1700 cm−1) indicated the initial structure of the proteins. This part of the spectrum was the most sensitive for detecting changes in secondary protein structure. [15] The amide II (1500–1600 cm−1) band was used to interpret the conformation of the secondary structure (α-helix) of proteinoids, considering the overlap of the absorption bands (peaks) that led to errors in interpretation.
In the amide III spectral range (1350–1200 cm−1), the bands emerged as a result of planar deformation of the N–H bond and C–N stretching, which was sensitive to changes in the spiral structure. For example, at 600–800 cm−1, it was possible to reveal an interaction with sulphates during “soft” acid hydrolysis. [16] In our case, integrated processing with alkali was followed by acid (all the treatments were performed in 1M NaCl) and possibly increased the polarity of the protein groups and the corresponding amplitude of the absorption band.
In comparison to the spectrometer database, the PM proteinoids were assigned to the “reference” groups: skin of various types and treatments, gelatin, and protein-foam generating agents. For comparison, we obtained the IR spectrum for edible gelatin [ here] represented by a smoothed line because of both purification during ashing and fractional melting of proteins on the automated line to produce higher-grade gelatin.
Thus, several strong absorption bands corresponding to peptide bonds were visualized in the IR spectra. The bands were associated with complex valence and deformation vibrations for the N–H and C–H types. IR spectra are thought to adequately reflect the secondary structures of proteins, and thus, the properties of the tropocollagenic particle or collagen molecule should be retained.
Compared to the gelatin food additive, the differences were associated with a more complex chemical composition of the raw material studied and the supramolecular-oriented architectonics of the fibrils and collagen fibre fascicles. [17] However, a comparison of the study results and references showed that the spiral conformation of the proteinoids retained [18] enabled the fullest potential of the functional and technological properties of the raw material. [19]
Consequently, positive changes were observed in the raw materials as a result of the preparation of the raw material for further processing into foodstuffs—in particular, the enhancement of lyophilic properties, fat extracting, bleaching, and “opening” of the chemical bonds of the protein. The maximum PM shelf life resulted from freeze-drying, complying with our recommendations. In this paper, the best processing method for the raw material was PM-5 [ here].
Development of a technology for production meat jelly
Since fibrillar animal proteins and their modification products can form jellies of considerable strength, approbation of the technological solution using jelly-like food materials was attempted because the substance in the jelly state was a matrix for meat and other food products after heat treatment. [20]
The results of the study clearly demonstrate that the resulting PM could be used as an ingredient for a wide range of food and culinary products to increase their consumer and functional properties. [21] We considered the recipe for jelly from grade ΙΙ beef compared to the “Collection of recipes for dishes and culinary products for public catering establishments”. [22] In this formulation, the edible gelatin was replaced with a PM to intensify the gelation and enrich the product with dietary fibre. This effect increased the activity of the functional groups of biopolymers of the collagen group via its modification.
The results of allowed us to emphasize that the PM has increased consumer value because of the dispersion of the structure of the raw animal material through hydrolytic conversion. It is possible to eliminate uncharacteristic inclusions (for example, hair follicles and bristle particles), i.e., to stabilize the quality of the products. With respect to protein or fat, the PM may not have an advantage over gelatin, but the enhanced hydrophilic properties of the modified preparation help form a food matrix that increases the moisture-binding capacity of the products, providing a more dense and relatively homogeneous product, and the energy of the product of the jelly-like structure is moderate. The edible gelatin (10 g) was added to the control jelly sample and a PM in an amount of ≈34 g was added to the test sample, considering the mass fraction of moisture in both, and the samples were boiled for a short time.
The casting of the food material was performed in moulds and placed in a refrigerating chamber for thermotropic gelation. The spice formulations are variable, and garlic, parsley, and spicy aromatic compositions, among others, can be also added. The gelation occurred at 15.7–16.5°C, which roughly corresponded to A. Weiss’s data for various types of gelatin. Starting at 16.5°C, the test jelly formed faster; the control sample gelled more slowly, and started at 15.7°C. This was especially noticeable in a thin layer of jelly (≈10 mm).
The microstructure of the jelly was a dense mass with sufficient electron density. Insignificant pores with a diameter of approximately 35 μm were found on the surface [ here]. The formation of the porous structure was apparently related to the molecular structure of the dispersed phase. The gelation was initiated by the formation of a framework of biopolymer molecules, the cells of which enclose water, solutions, and minor food compounds that can enrich production with biologically active substances.
The control image analysis of the same samples at the level of light microscopy showed that the main part was represented by a homogeneous basophilic-coloured mass of hydrolysed collagen and elastin. The connective tissue particles overall retained the structural organizations of the fibres (cell elements were not detected and fibrils strongly loosened), along with separate fragments of muscle fibres. Simultaneously, elements of connective and muscular tissue in the control were visualized better than in the test sample.
Some physicochemical parameters of the jellies are presented in . The energy values of these jellies were as follows: 130.2 (kcal/100 g) for the control sample and 140.6 (kcal/100 g) for the test sample. These may serve as a source of dietary fibre analogues and impart dietary properties to products. The critical fracture stress approximately corresponded to similar parameters for meat systems structured with functional animal protein. [6] For this parameter, the advantage was noted for the test sample. At the final stage, the dispersed PM could replace natural meat raw material in the model systems. Thus, the model meat systems were shown to have high sensory parameters.
Table 3. Physicochemical parameters of jellies.
The result of the research studies performed has demonstrated the possibility and expediency of using secondary sources of collagen-containing raw materials to develop a polyfunctional biopolymer module. The complex of physicochemical parameters for this PM enables the development of a wide range of food products; the important quality indicator is the presence of a stable, relatively homogeneous structure.
Conclusion
A comprehensive analysis of the research results, including data from physicochemical, histological, and spectral methods, led to the conclusion concerning the formation of dispersed food systems in accordance with the formulations. In particular, the dispersed PM was shown to be able to replace meat raw material in the meat jelly formulations. The model jellies were established to have good appearance, physico-structural properties, composition, and other consumer characteristics. The studies demonstrated the possibility and expediency of using secondary resources of collagen-containing raw material to develop a polyfunctional biopolymer module. The formation of a very dense dispersed phase of the module with food substances, spices, and other ingredients, along with a complex of its physicochemical parameters, have shown that the experimental development of a wide range of meat products is possible.
Acknowledgments
The authors declare that they have no conflicts of interest. This study did not involve any human or animal testing. Written informed consent was obtained from all study participants.
Additional information
Funding
References
- Gomez-Guillen, M. C.; Gimenez, B.; Lopez-Caballero, M. E.; Montero, M. P. Functional and Bioactive Properties of Collagen and Gelatin from Alternative Sources: A Review. Food Hydrocolloids 2011, 25, 1813–1827. DOI: 10.1016/j.foodhyd.2011.02.007.
- Nygaard, M.;. How Can Collagen Peptides Help You Meet the Latest Consumer Demands? Agro Food Industry Hi-Tech 2016, 27, 20–21.
- Ashrafie, N. T.; Azizi, M. H.; Taslimi, A.; Mohammadi, M.; Neyestani, T. R.; Mohammadifar, M. A. Development of Reduced-Fat and Reduced-Energy Dark Chocolate Using Collagen Hydrolysate as Cocoa Butter Replacement Agent. Journal of Food and Nutrition Research 2014, 53, 13–21.
- Lee, C. H.; Chin, K. B. Effects of Pork Gelatin Levels on the Physicochemical and Textural Properties of Model Sausages at Different Fat Levels. LWT-Food Science and Technology 2016, 74, 325–330. DOI: 10.1016/j.lwt.2016.07.032.
- Sousa, S. C.; Fragoso, S. P.; Penna, C. R. A.; Arcanjo, N. M. O.; Silva, F. A. P.; Ferreira, V. C. S.; Barreto, M. D. S.; Araujo, I. B. S. Quality Parameters of Frankfurter-Type Sausages with Partial Replacement of Fat by Hydrolyzed Collagen. LWT-Food Science and Technology 2017, 76, 320–325. DOI: 10.1016/j.lwt.2016.06.034.
- Kurchaeva, E. E.; Kitsuk, S. V. Use of the Plant and Animal Origin Raw Materials for the Production of Meat Functional Products. Izvestia Vuzov. Pishevaya Tekhnologia [News Institutes Higher Education of Food Technology] 2012, 2-3, 55–58.
- Lukin, A. A.; Merenkova, S. P. A Method for Producing a Protein Stabilizer Sausage. Tehnologija I Tovarovedenie Innovacionnyh Pishhevyh Produktov [Technology Merchandising of the Innovative Foodstuff] 2016, 1(36), 15–19.
- Titov, E. I.; Apraksina, S. K.; Mitaseva, L. F.; Sokolov, A. Y.; Litivinova, E. V. Development of a Method for Producing a Biomodified Collagen Preparation to Create a Biologically Active Complex on Its Basis. The invention application # RU 2015109790. 2016 http://www.fips.ru/Archive4/PAT/2016FULL/2016.10.10/Index_ru.htm ( accessed Aug 23, 2017).
- Li, Y.; Douglas, E. P. Effects of Various Salts on Structural Polymorphism of Reconstituted Type I Collagen Fibrils. Colloids and Surfaces B: Biointerfaces 2013, 112, 42–50. DOI: 10.1016/j.colsurfb.2013.07.037.
- Semenov, G. V.;. Vacuum Freeze Drying DeLi Plus: Moscow, 2013; pp 264.
- Sarkisov, D. S.; Perov, Y. L. Microscopic Technology Medicine: Moscow, 1996; pp 544.
- Pchelkina, V. A.; Khvilya, S. I. Practical Aspects of Application of GOST 31474-2012 Meat and Meat Products. Histological Method of Plant Protein Additive Identification. Tehnika Tehnologija Pishhevyh Proizvodstv Food Processing Technology 2015, 3(38), 51–55.
- Vidal, B. D. C.;. Using the FT-IR Linear Dichroism Method for Molecular Order Determination of Tendon Collagen Bundles and Nylon 6. Acta Histochemica 2013, 115, 686–691. DOI: 10.1016/j.acthis.2013.02.002.
- Santana, R. C.; Perrechil, F. A.; Sato, A. C. K.; Cunha, R. L. Emulsifying Properties of Collagen Fibers: Effect of pH, Protein Concentration and Homogenization Pressure. Food Hydrocolloids 2011, 25, 604–612. DOI: 10.1016/j.foodhyd.2010.07.018.
- Drobota, M.; Grierosu, I.; Radu, I.; Vasilescu, D. S. The Effect of Silver Nanoparticles on the Collagen Secondary Structure. Key Engineering Materials 2014, 638, 8–13. DOI: 10.4028/www.scientific.net/KEM.638.8.
- Pielesz, A. Temperature-Dependent FTIR Spectra of Collagen and Protective Effect of Partially Hydrolysed Fucoidan. Spectrochimica Acta Part A: Molecular and Biomolecular Spectroscopy 2014, 118, 287–293. DOI: 10.1016/j.saa.2013.08.056.
- Liu, D.; Nikoo, M.; Boran, G.; Zhou, P.; Regenstein, J. M. Collagen and Gelatin. Annual Review of Food Science and Technology 2015, 6 6, 527–557. DOI: 10.1146/annurev-food-031414-111800.
- Li, B. F.; Guo, M.; Hou, H.; Wang, S. S. Physicochemical Properties of Acid-Soluble Collagen from Skin of Clarias Fuscus. Modern Food Science & Technology 2013, 29(11), 2580–2585.
- Wang, W.; Zhang, Y.; Ye, R.; Zhao, W. Physicochemical Characteristics and Gelation Properties of Collagen Superfine Powder from Swine Skin: The Effects of Preheating Treatment. International Journal of Food Science and Technology 2016, 51, 1291–1297. DOI: 10.1111/ijfs.13073.
- Banerjee, S.; Bhattacharya, S. Food Gels: Gelling Process and New Applications. Critical Reviews in Food Science and Nutrition 2012, 52, 334–346. DOI: 10.1080/10408398.2010.500234.
- Ferraro, V.; Anton, M.; Sante-Lhoutellier, V. The “Sisters” Alpha-Helices of Collagen, Elastin and Keratin Recovered from Animal By-Products: Functionality, Bioactivity and Trends of Application. Trends in Food Science and Technology 2016, 51, 65–75. DOI: 10.1016/j.tifs.2016.03.006.
- Collection of Recipes for Dishes and Culinary Products for Public Catering Establishments. Economics, Moscow. 1981 https://edu.tatar.ru/upload/images/files/%D1%81%D0%B1%D0%BE%D1%80%D0%BD%D0%B8%D0%BA%281%29.pdf (accessed Aug 23, 2017).