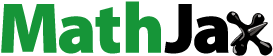
ABSTRACT
The composite bilayer film based on polyvinyl alcohol, sodium alginate, and chitosan were developed in this study, and the effects of the related coating on internal-quality changes of salted duck eggs (SDEs) during storage were determined. The results showed that the overall properties of the bilayer film were better than those of monolayer films, including storage stability. Coatings could significantly (p < 0.05) reduce quality deterioration of SDEs such as water loss, oil exudation, and gritty. The bilayer coating was more effective in inhibiting the increase of egg yolk pH and total bacterial counts than monolayer coatings. After storage for 60 days, water content of bilayer coated SEDs was 19.7% higher than that of the control SDEs, oil exudation was 38% higher, and gritty texture was 27.9% higher. Moreover, bilayer coating could prolong the shelf life of SDEs to more than 60 days at 25°C and 50% relative humidity according to the results of total bacterial count experiment, while the shelf life of control SDEs was less than 45 days. This study indicated the potential application of bilayer composite coating for preservation of SDEs.
Introduction
Salted duck egg (SDE) is one of the most popular preserved egg products in China and Southeast Asia.[Citation1] The orange color, high oil exudation, and gritty texture of egg yolk (GE) are attractive to customers. In order to prolong the shelf life of SDEs, manufacturers always adopt vacuum-packing and high-temperature sterilization process.[Citation2] However, the above process not only increases breakage of the product but produces a large amount of plastic waste. Biodegradable film/coating is a potential alternate of plastic packaging, and it can retard deterioration and enhance the product quality and stability as well.[Citation3] Many degradable materials have been applied on shell eggs to preserve the internal qualities, including synthetic polymers such as polysaccharides and proteins.[Citation3–Citation8] However, little information is available about the preservation effect of degradable coatings on SDEs. Wang et al.[Citation9] reported that composite coating based on nano-SiO2-modified polyvinylidene chloride could significantly (p < 0.05) reduce quality deterioration of SDEs such as weight loss, total volatile basic nitrogen (TVB-N), and total bacterial counts.
Among the commonly used polymers, polyvinyl alcohol (PVA) is a widely applied raw material with excellent film forming property, high tensile strength (TS) and nice chemical stability, but poor water barrier ability.[Citation4] Chitosan (CHI) is another promising film-forming substance due to its antimicrobial activity, nontoxicity, and low oxygen permeability.[Citation5,Citation6] CHI contains free hydroxyl and amine groups, making it miscible with PVA by hydrogen bonds,[Citation7] which improves the characteristics of PVA film. It was reported that the composite films based on PVA and CHI had enhanced physical properties and high antioxidant activities.[Citation7,Citation8] Sodium alginate (SA) is a natural polysaccharide that can produce insoluble film by crosslinking of polyvalent metal cations.[Citation10] Like CHI, SA has some special features, such as biocompatibility, chelating ability, and high hydrophilicity. And, the resultant film/coating could maintain the qualities of foods well such as reducing water loss and shrinkage distortion, maintaining flavor, and retarding fat oxidation.[Citation11] Tang et al.[Citation12] stated that the incorporation of nano-cellulose into SA-based film led to significant increase of water barrier ability, TS, and Young’s modulus.
Compared to the limited properties of monolayer film, bilayer film can take better advantages of the unique characteristics of the substrates and achieve better functional performances. Ferreira et al.[Citation13] who classified bilayer films of FucoPol and CHI showed favorable optical, mechanical, and barrier properties than FucoPol monolayer films. Thu et al.[Citation14] reported that bilayer films based on alginate can be used as slow-release wound healing vehicles, in which the drug-free inner layer acts as a rate-controlling membrane.
Our research group has studied properties of bilayer film based on PVA, CHI, and SA, and quality changes of fresh hen eggs coated with the bilayer film during 15 days storage at 25°C.[Citation15] Results indicated that the developed bilayer film had good water barrier, mechanical and antibacterial properties, and bilayer coating could reduce weight loss, maintain Haugh unit and yolk index of eggs. The aim of present work was to further verify the application of the composite bilayer film by evaluating its storage stability and determining the preservation effect on SDEs.
Material and methods
Materials
SDEs (unwashed, non-feces, 65–70 g) were obtained from the Red Sun Company (Gaoyou, Jiangsu, China). Once the SDEs are produced, they are sent to the lab as soon as possible. Chemical grade PVA and nano-TiO2 were obtained from Shanghai Aladdin Chemical Reagent Co. Ltd. (China). CHI was purchased from Nantong Xingcheng Biological Industrial Co. Ltd. (China). SA, ethyl paraben sodium, nano-cellulose, and Tween 80 were obtained from Sinopharm Chemical Reagent Co. Ltd. (China). Calcium chloride, anhydrous acetic acid, and glycerol were purchased from Shanghai Lingfeng Chemical Reagent Co. Ltd. (China).
Preparation of coating solutions
Outer layer film forming solution: CHI solution (2%, w/v) was prepared by dissolving CHI in acetic acid (1%, w/w) with addition of 25% (w/w CHI).[Citation15] PVA solution (5%, w/v) was prepared by dissolving PVA in distilled water with addition of 25% (w/w PVA) and stirred in a water bath (THM-2865, Thermo Fisher Scientific, UN) at 85°C for 2 h.[Citation15] When PVA solution cooled to room temperature, CHI and PVA solutions were stirred together for 15 min. The pH of the mixed solution was adjusted to pH 4.5 with acetic acid, and then 0.1% (w/w) of TiO2 was added to enhance the mechanical and barrier properties of resulted film. The solution was homogenized (Polytron PT 10-35GT, Kinematica AG, Switzerland) and vacumized (−0.1 MPa) for 1 h to remove air bubbles.
Inner layer film forming solution: SA solution (1.25%, w/v) was prepared by dissolving SA in distilled water,[Citation15] with addition of 20% glycerol (w/w SA). Nano-cellulose (10%, w/w SA), Tween 80 (0.175%, w/v), and ethyl paraben sodium (0.125%, w/v) were added to enhance the properties of resulted film. The above mixture was stirred at 25°C and the pH was adjusted to 4.5. The solution was vacumized (−0.1 MPa) for 1 h to remove air bubbles.
Film preparation
For SA or PVA–CHI monolayer films, 350 mL of SA solution or 200 mL of PVA–CHI solution was poured onto a horizontal glass plate (25 × 25 cm2) and dried at room temperature for 24 h. The film was then quickly (<30 s) wetted with 10 mL of calcium chloride solution (0.5% CaCl2, w/v) to enhance the cross linking of matrix and improve macro-properties of films[Citation15] and dried again at room temperature for 12 h.
Bilayer film was prepared using a two-step technique.[Citation15] The inner layer was prepared by casting SA solution (220 mL) on a horizontal glass plate and drying at room temperature for 24 h. Subsequently, PVA–CHI solution (100 mL) was casted on the inner layer and dried at room temperature for 24 h. The resultant bilayer film was quickly (<30 s) wetted with 10 mL of 0.5% of CaCl2 solution (w/v) and dried at room temperature for 12 h. The dried films were stored at 25°C and 50% relative humidity for 60 days. Film properties were measured for three replicates per treatment other than mentioned every 15 days.
SDEs sample treatment
Before coating, the total of 240 medium-sized (65–70 g) SDEs were wiped to avoid water condensation on the SDEs surface. For monolayer coating, the eggs were dipped into the solutions for 60 s and dried out for 4 h. For bilayer coating, the eggs were first dipped into SA solution for 30 s and then in CaCl2 solution for 15 s. The coated eggs were dipped into PVA–CHI solution for another 30 s and dried out for 4 h. The SDEs were divided into four groups: P-group, coated with PVA–CHI solution; S-group, coated with SA solution; B-group, coated with bilayer coatings; and C-group, non-coated SDEs as the control. The eggs were then stored at 25°C and 50% relative humidity to simulate the climate of Shanghai at spring and summer. Quality measurements were performed by three replicates after mixing 10 SDEs per treatment every 15 days.
Measurement of film properties
Water content and water solubility
The water content and water solubility (WS) were determined according to literature.[Citation15] The weight change of the film fragment was measured after drying for 24 h at 105°C and was recorded as water content. WS was calculated as following:
where M0 is the initial weight of dried film (g) and M1 is the weight of undissolved dried film (g).
Water vapor permeability
Water vapor permeability (WVP) was determined following the procedure described by Zhuang et al.[Citation15] The WVP (g mm m−2 d−1 kPa−1) was then calculated as following:
where m is the weight of water permeated through the film (g), L is the thickness of the film (m), A is the permeation area (m2), t is the time of permeation (s), and ΔP is the water vapor pressure difference across the film (Pa).
Mechanical properties
Mechanical properties including TS and elongation at break (EB) were determined by Texture Analyser (TA.XTPLUS, StableMicroSystem, UK) as described by Zhuang et al.[Citation15] Measurements were performed on eight replicates per treatment. TS and EB could be calculated as following:
where Fm is the measured maximum load (N); L and W are the thickness and width of the film (m), respectively.
where lmax is the distance at break (m); l0 is the original length (m).
Measurement of SDEs quality
Water content and pH of SDEs
SDEs were mashed and water content was measured after drying at 105°C for 20 h. pH of SEDs was measured as described by Dou et al.[Citation16] The mashed sample (~1 g) was diluted in a 1:10 (w/v) ratio with distilled water and homogenized for 2 min at 15,000 rpm. The pH value of the homogenized solution was measured using a pH meter (Mettler-Toledo MP 220, Schwerzenbach, Switzerland).
Oil exudation of egg yolk
Oil exudation of egg yolk (OE) was measured as described by Harlina et al.[Citation17] Briefly, SDE yolk (5 g) was homogenized with 35 mL of n-hexane/2-propanol (3:2, v/v) at 5000 rpm for 10 min. The filtrate was evaporated in a water bath at 55°C and then dried at 105°C until constant weight was obtained. The residue was weighed and taken as total lipid content. To determine free lipid content, yolk (5 g) was mixed with 25 mL of distilled water and homogenized at 5000 rpm for 30 s. The homogenate was centrifuged at 10,000 rpm for 30 min at 25°C, and the supernatant was added with 25 mL of n-hexane/2-propanol (3:2, v/v) to dissolve the float. The solvent-lipid layer obtained was separated using a separating funnel. Thereafter, the solvent was evaporated in a water bath at 80°C and dried at 105°C to constant weight. The dried residue was weighed and taken as free lipid. The OE (%) was calculated as following:
where FC is the free lipid content (g); TC is the total lipid content (g).
GE
Gritty texture of SDE yolk was measured as described by Wang.[Citation18] Briefly, yolk (~5 g) was stirred with petroleum ether (50 mL) for 10 min and then filtered using a standard 20-mesh sieve. The filtered yolk on the standard mesh was rinsed using petroleum ether (100 mL) and dried to constant weight at 105°C. The GE (%) was calculated as follows:
where G1 is the weight of the SDE yolk (g), G2 is the weight of the standard sieve (g), G3 is the weight of standard sieve with egg yolk residue (g).
SDE microbiological determination
SDE was mashed on aseptic console. Samples (~1 g) were mixed with sterile saline (9 mL), and the number of colonies was measured as described by Liao et al.[Citation19]
Sensory evaluation of SDE yolk
Sensory evaluation of SDE yolk was evaluated for likeness by 10 panelists.[Citation1] The panelists were the students in Department of Food Science and Technology, School of Agriculture and Biology, Shanghai Jiao Tong University of age ranging from 20 to 25 years. Panelists had sensorial acquaintance with SDE yolk. A 10-point hedonic scale, in which a score of 1 = dislike extremely, 5 = neither like nor dislike, and 10 = like extremely, was used for evaluation. The panelists were asked to evaluate each sample for color, texture, odor, and oil exudation.
Statistical analysis
The results were expressed as average ± standard deviation, and the variance was analyzed with SPSS Statistics 19 (version 13.0, Statistical Package for the Social Sciences Inc., USA) using ANOVA procedures. Multiple comparisons were conducted by LSD, and p < 0.05 was considered to be statistically significant.
Results and discussion
Properties stability of films during storage
Water content and water solubility
The average film thickness was ca. 0.1 mm in present study. Water content and solubility changes of films were presented in . Water content of bilayer film was between the values of SA and PVA–CHI films at initial, and water content gradually increased within 45 days which might be due to water absorption from the external atmosphere.[Citation20] Water content of SA and PVA–CHI films decreased after 45 days while the value of bilayer film kept increasing until 60 days. Kim et al.[Citation6] stated that the increase of water content generally led to the enhancement of TS and decline of EB. Zhuang et al.[Citation21] also indicated that high water content could accelerate the degradations of bilayer film and increase the WVP as well.
Table 1. Film property changes during storage.
Water solubility of SA film was more than 80% due to its hydrophilicity in nature, whereas water solubility of the other films was less than 50%. Water solubility is an important indicator for film performance, with low value that is necessary for protection of foodstuffs at high or intermediate water activity.[Citation22] After the storage of 60 days, water solubility of SA films decreased from 91.52% to 53.43%. And, water solubility of bilayer film remained constant for 60 days, indicating that the film solubility was relatively stable. Ortegatoro et al.[Citation23] also pointed out that water solubility of bilayer changed little after 5 weeks of storage.
WVP
WVP results were shown in . The WVP value of bilayer was the lowest as compared with monolayer films at day 0, with values of 156.9, 290.5, 224.6 g mm m−2 d−1 kPa−1, respectively, which was coherent with the study of Jridi et al.[Citation24] The result indicated that the interfacial interaction among PVA, CHI, and SA led to the formation of a more close-knit matrix, and the transfer of water molecules through the network was limited. WVP is an important parameter for food packaging, with low value representing better water barrier ability, which is a benefit for the preservation effect. In present study, the WVP of bilayer film was significantly lower than those of other films within the first 15 days, but the value gap was reduced gradually. And, there were no obvious value differences among films at the end of 60 days. It was reported that WVP depended on water diffusivity and solubility in a polymeric matrix.[Citation25] As water molecular was harder to diffuse through bilayer film, WVP of bilayer film was low at initial. With the extension of time, film adsorbed more water () and WVP of each film approached gradually. Ortegatoro et al.[Citation23] reported, after 5 weeks of storage, no noticeable changes in the WVP values were observed and the bilayer films still maintained their low WVP values.
Mechanical properties
Food packaging is required of certain mechanical properties to withstand the stress that occurs during shipping, handling, and storage. The mechanical properties of films were showed in . The flexibilities of bilayer and SA films were similar (EB ca.108%), while the EB of PVA–CHI film was higher with value of 150%. During storage, the EB values of all the films were still higher than 100%, which was flexible enough for biodegradable films. As for TS values, no differences were observed between the PVA–CHI and SA films, while TS of the bilayer film was 30% lower. According to Ferreira et al.[Citation13] and Sanyang et al.,[Citation26] the mechanical properties of bilayer films were between the values of each monolayer film and were close to the smaller values. For example, TS of sugar palm starch (SPS) monolayer film, poly(lactic acid) (PLA) monolayer film, and SPS–PLA bilayer film at ratio of 1 was 7.6, 18.5, and 11.9 MPa, respectively. And, TS values of FucoPol monolayer film, CHI monolayer film, and FucoPol–CHI bilayer film at ratio of 1 were 7.7, 24.8, and 13.6 MPa respectively. The reduced TS of bilayer film in present study might be caused by the heterogeneity of the polybasic.[Citation15] In addition, the TS values remained constant after the storage of 60 days, which indicated mechanical stability.
Quality of SDEs during storage
Water content and pH of SDEs
Changes of water content of SDEs during storage were presented in . Water content of SDEs generally decrease during storage. These results indicated that dehydration occurred in SDEs during storage because of the osmotic pressure by salts, and water migrated from yolk to albumen and then to the environment through the pores of the eggshells.[Citation27] However, there was an inflection point at 45 days, and water content of control group increased to 64.9%, which might be resulted from the spoilage of the products. Water content of SDEs in B- and P-groups was higher than that of other products, and the change rangeability during storage was small as well, implying the barrier roles of the related coatings. At the end of 60 days, water content of control group decreased to 54.3%, significantly lower than that of B-group (p < 0.05).
Figure 2. The water content (a) and pH (b) of SDEs during storage.
C: Non-coated SDEs; B: bilayer-coated SDEs; P: PVA–chitosan-coated SDEs; S: sodium alginate-coated SDEs.
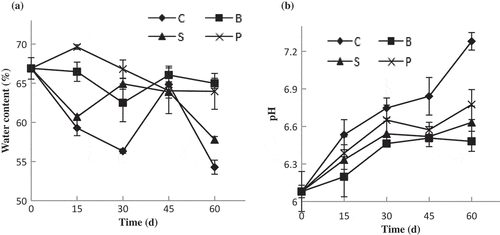
As shown in , the pH values of the studied SDEs increased during storage, which was coherent with the study of Wang et al.[Citation9] In addition, the observed pH of coated SDEs changes was mild () especially for coated SDEs with bilayer film. After 60 days, the pH of non-coated SDEs was 7.28, while the values were 6.48, 6.63, and 6.77, respectively, for B-, S-, and P-groups.
OE and GE
Oil exudation (OE) during storage was shown in . The OE value of SDE at day 0 was 68.7%, and it gradually decreased with time going. After 60 days, the OE of non-coated SDEs decreased by 50%. OE is one of the most important sensory characteristics of SDEs for consumers, and a higher OE value is preferred.[Citation28] During production of SDEs, salting solution changed the structure of low-density lipoprotein micelles and induced protein denaturation, leading to the release of free lipid and the OE phenomenon.[Citation29] During storage, free lipids might be agglomerated and refastened by protein and cause the reducing of OE.[Citation30] However, the coatings, especially bilayer coating, obviously delayed the reduce of OE, with values of the B-, S-, and P-groups decreasing by 31%, 37%, and 44%, respectively, at the end of storage.
Figure 3. The egg yolk oil exudation (a) and egg yolk gritty (b) during storage.
C: Non-coated SDEs; B: bilayer-coated SDEs; P: PVA–chitosan-coated SDEs; S: sodium alginate-coated SDEs
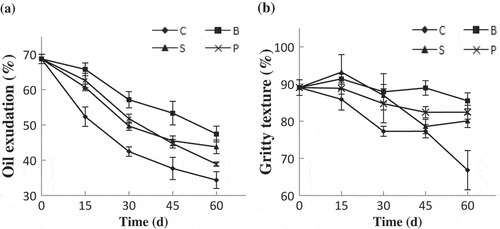
During production of SDEs, the interaction between lipoproteins can occur with the product being dehydrated, and the yolk protein becomes more concentrated, giving the yolk a gritty texture, which is favorite by consumers.[Citation31] Yang and Hsu[Citation32] reported that yolk formed polyhedral granules with diameters of 23–127 µm and provided the gritty texture sensation. showed that the GE decreased during storage. According to Kaewmanee et al.,[Citation1] SDEs with good eating qualities have GE values of more than 80%. In our experiment, the GE value of non-coated SDEs was significantly lower than those of coated SDEs, decreasing to 66.83% after 60 days. The GE values for the B-, S-, and P-groups were 85%, 80%, and 82%, respectively.
Microbiological analyses
Microorganism is an important factor to determine if the product is spoiled. When the total amount of microorganism in food is close to 500 CFU/g, the food will be not suitable to eat.[Citation19] The numbers of colonies in SDEs were shown in . It was found that the total number of colonies in the C- and S-groups increased as storage progressed, and the increase trends were more significantly after 30 days. The total number of colonies in the C-group was already over 500 CFU/g after 45 days, and the value of the S-group exceeded the national standard after 60 days. However, no visible bacterial colonies were observed in the B- and P-groups during the storage time. In present study, the antibacterial effect was mainly caused by the synergistic actions of ethyl paraben sodium, CHI, and nano-TiO2.[Citation15] Moreover, coatings could also block the pores on shells of SDEs and reduce the entrance of microorganisms, but the effect was not obvious.
Sensory evaluation of egg yolk
The sensory evaluation of non-coated and coated SDE yolk during storage was presented in . The overall sensory score of egg yolk decreased with time, and this was probably due to a combination of water loss, lipid decomposition, and microorganisms growth of SDEs. During storage, the color and odor of egg yolk changed more quickly, and both scores in control samples were sharply reduced to less than 2.00 after 45 days. Compared with the other three groups, B-group got the highest score during storage. Moreover, after 60 days, the score of B-group was 30.51, even higher than that of C-group at 15 days, which indicated that bilayer coating was effective in inhibiting the deterioration of sensory quality of egg yolk, especially on the performances of OE (seen in ).
Table 2. Sensory evaluation of SDEs yolk during storage.
Conclusion
Bilayer film composed of PVA, CHI, and SA possessed better storage stability considering mechanical property, solubility, and water barrier ability as compared with that of the monolayer films. Bilayer coating was efficient in maintaining the interior qualities of SDEs when stored at room temperature and could prolong the shelf life of SDEs at least to 60 days, while the control SDEs with shelf life within 45 days. Further work is needed about the effects of truck-simulation vibration on breakages and quality changes of coated SDEs to investigate the protection performance of bilayer coatings during transportation.
Additional information
Funding
References
- Kaewmanee, T.; Benjakul, S.; Visessanguan, W. Effect of Acetic Acid and Commercial Protease Pretreatment on Salting and Characteristics of Salted Duck Egg. Food Bioprocess Technology 2012, 5(5), 1502–1510. DOI: 10.1007/s11947-011-0510-1.
- Forson, A.; Ayivor, J. E.; Banini, G. K.; Nuviadenu, C.; Debrah, S. K. Evaluation of Some Elemental Variation in Raw Egg Yolk and Egg White of Domestic Chicken, Guinea Fowl and Duck Eggs. Annalen Biology Research 2011, 2(6), 676-680.
- Koide, S.; Shi, J. Microbial and Quality Evaluation of Green Peppers Stored in Biodegradable Film Packaging. Food Control 2011, 18, 1121–1125. DOI: 10.1016/j.foodcont.2006.07.013.
- Tang, X.; Alavi, S. Recent Advances in Starch, Polyvinyl Alcohol Based Polymer Blends, Nanocomposites and Their Biodegradability. Carbohydrate Polymers 2011, 5(1), 7–16. DOI: 10.1016/j.carbpol.2011.01.030.
- No, H. K.; Meyers, S. P.; Prinyawiwatkul, W. Applications of Chitosan for Improvement of Quality and Shelf Life of Foods: A Review. Journal of Food Science 2007, 72(5), R87–R100. DOI: 10.1111/j.1750-3841.2007.00383.x.
- Kim, J. Y.; Choi, Y. G.; Kim, S. R. B.; Lim, S. T. Humidity Stability of Tapioca Starch-Pullulan Composite Films. Food Hydrocoll 2014, 41(41), 140–145. DOI: 10.1016/j.foodhyd.2014.04.008.
- Bonilla, J.; Fortunati, E.; Atares, L.; Chiralt, A. Physical Structural and Antimicrobial Properties of Polyvinyl Alcohol-Chitosan Biodegradable Films Food Hydrocolloid 2014, 35, 463–470. DOI: 10.1016/j.foodhyd.2013.07.002.
- Hajji, S.; Chaker, A.; Jridi, M.; Maalej, H.; Jellouli, K.; Boufi, S.; Nasri, M. Structural Analysis, and Antioxidant and Antibacterial Properties of Chitosan-Poly Vinyl Alcohol Biodegradable Films. Environment Science Pollution Research 2016, 23(15), 15310–15320. DOI: 10.1007/s11356-016-6699-9.
- Wang, F.; Yan, W.; Liang, Y.; Zhao, J.; Nano-SiO2, Z. J. Modified PVDC Coating Preserves the Quality of Salted Duck Eggs. Food Science 2016, 37(24), 306-312.
- Liakos, I.; Rizzello, L.; Scurr, D. J.; Pompa, P. P.; Bayer, I. S.; Athanassiou, A. All-Natural Composite Wound Dressing Films of Essential Oils Encapsulated in Sodium Alginate with Antimicrobial Properties. International Journal of Pharmaceutics 2014, 463(2), 137–145. DOI: 10.1016/j.ijpharm.2013.10.046.
- Navanietha, K. R.; Karthikeyan, R.; Berchmans, S. Functionalization of Electrochemically Deposited Chitosan Films with Alginate and Prussian Blue for Enhanced Performance of Microbial Fuel Cells. Electrochimica Acta 2013, 112(12), 465–472. DOI: 10.1016/j.electacta.2013.08.180.
- Tang, Q.; Pan, D.; Sun, Y.; Cao, J.; Guo, Y. Preparation, Characterization and Antimicrobial Activity of Sodium Alginate Nanobiocomposite Films Incorporated with Ε-Polylysine and Cellulose Nanocrystals. Journal Food Processing Preservation 2016, 45(5), e13120. DOI: 10.1111/jfpp.13120.
- Ferreira, A. R. V.; Torres, C. A. V.; Freitas, F.; Sevrin, C.; Grandfils, C.; Reis, M. A. M.; Alves, V. D.; Coelhoso, I. M. Development and Characterization of Bilayer Films of FucoPol and Chitosan. Carbohydrate Polymers 2016, 147, 8–15. DOI: 10.1016/j.carbpol.2016.03.089.
- Thu, H. E.; Zulfakar, M. H.; Ng, S. F. Alginate Based Bilayer Hydrocolloid Films as Potential Slow-Release Modern Wound Dressing. International Journal of Pharmaceutics 2012, 434(1–2), 375–383. DOI: 10.1016/j.ijpharm.2012.05.044.
- Zhuang, C. J.; Jiang, Y. L.; Zhong, Y.; Zhao, Y. L.; Deng, Y.; Yue, J.; Wang, D. F.; Jiao, S. S.; Gao, H. Y.; Chen, H. J.;, et al. Development and Characterization of Nano-Bilayer Films Composed of Polyvinyl Alcohol, Chitosan and Alginate. Food Control 2018, 86, 191–199. DOI: 10.1016/j.foodcont.2017.11.024.
- Dou, H.; Magnusson, E.; Choi, J.; Duan, F.; Nilsson, L.; Lee, S. Study on Aggregation Behavior of Low Density Lipoprotein in Hen Egg Yolk Plasma by Asymmetrical Flow Field-Flow Fractionation Coupled with Multiple Detectors. Food Chemistry 2015, 192, 228–234. DOI: 10.1016/j.foodchem.2015.07.019.
- Harlina, P. W.; Shahzad, R.; Ma, M.; Geng, F.; Wang, Q.; He, L. Effect of Garlic Oil on Lipid Oxidation, Fatty Acid Profiles and Microstructure of Salted Duck Eggs. Journal Food Processing Preservation 2015, 39(6), 2897–2911. DOI: 10.1111/jfpp.12541.
- Wang, T. H.;. Salting Yolks Directly Using Fresh Duck Egg Yolks with Salt and Maltodextrin. Journal Pollution Science 2017, 54(1). DOi: 10.2141/jpsa.0160027.
- Liao, M. B.; Chen, X. B.; Lai, M. H. Notes of Test of Food Total Number of Colonies by GB Methed and Microbial Rapid Test Card. Food and Nutrition in China 2013, 19(7), 16-18.
- Ortegatoro, R.; Morey, I.; Talens, P.; Chiralt, A. Influence of Citric Acid on the Properties and Stability of Starch-Polycaprolactone Based Films. Journal of Applied Polymer Science 2015, 133(2), e42220. DOI: 10.1002/app.42220.
- Zhuang, J.; Dai, W.; Tian, Q.; Li, Z.; Xie, L.; Wang, J. Photocatalytic Degradation of RhB over TiO2 Bilayer Films: Effect of Defects and Their Location. Langmuir 2010, 26(12), 9686. DOI: 10.1021/la100302m.
- González, A.; Igarzabal, C. I. A. Soy Protein – Poly (Lactic Acid) Bilayer Films as Biodegradable Material for Active Food Packaging. Food Hydrocolloid 2013, 33(2), 289–296. DOI: 10.1016/j.foodhyd.2013.03.010.
- Ortegatoro, R.; Morey, I.; Talens, P.; Chiralt, A. Active Bilayer Films of Thermoplastic Starch and Polycaprolactone Obtained by Compression Molding. Carbohydrate Polymers 2015, 127, 282–290. DOI: 10.1016/j.carbpol.2015.03.080.
- Jridi, M.; Hajji, S.; Ayed, H. B.; Lassoued, I.; Mbarek, A.; Kammuon, M. Physical, Structural, Antioxidant and Antimicrobial Properties of Gelatin–Chitosan Composite Edible Films. International Journal of Biological Macromolecules 2014, 67(3), 373–379. DOI: 10.1016/j.ijbiomac.2014.03.054.
- Zhong, Y.; Li, Y. Effects of Surfactants on the Functional and Structural Properties of Kudzu (Pueraria Lobata) Starch/Ascorbic Acid Films. Carbohydrate Polymers 2011, 85(3), 622–628. DOI: 10.1016/j.carbpol.2011.03.031.
- Sanyang, M. L.; Sapuan, S. M.; Jawaid, M.; Ishak, M. R.; Sahari, J. Development and Characterization of Sugar Palm Starch and Poly(Lactic Acid) Bilayer Films. Carbohydrate Polymers 2016, 146, 36–45. DOI: 10.1016/j.carbpol.2016.03.051.
- Lian, Z.; Qiao, L.; Zhu, G.; Deng, Y.; Qian, B.; Yue, J. Use of Sodium Dodecyl Sulfate Pretreatment and 2-Stage Curing for Improved Quality of Salted Duck Eggs. Journal of Food Science 2014, 79(3), 354–361. DOI: 10.1111/1750-3841.12361.
- Harini, S. T.; Padmavathi, S.; Satish, A.; Raj, B. Food Compatibility and Degradation Properties of Pro-Oxidant-Loaded LLDPE Film. Journal of Applied Polymer Science 2014, 131(2), 218–224. DOI: 10.1002/app.39756.
- Thammarat, K.; Soottawat, B.; Wonnop, V. Changes in Chemical Composition, Physical Properties and Microstructure of Duck Egg as Influenced by Salting. Food Chemistry 2009, 112(3), 560–569. DOI: 10.1016/j.foodchem.2008.06.011.
- Upadhyaya, I.; Yin, H. B.; Surendran, N. M.; Chen, C. H.; Lang, R.; Darre, M. J. Inactivation of Salmonella Enteritidis on Shell Eggs by Coating with Phytochemicals. Poultry Science 2016, 95(9), pew152. DOI: 10.3382/ps/pew152.
- Dang, K. L. M.; Le, T. Q.; Songsermpong, S. Effect of Ultrasound Treatment in the Mass Transfer and Physical Properties of Salted Duck Eggs. Food Innovation Asia Conference 2014 “Science and Innovation for Quality of Life. BITEC, Bangkok, Thailand, June 12-13, 2014, Vol.48.
- Yang, S. C.; Hsu, H. K. Scanning Electron Microstructure of the Yolk of Duck Egg and Duck Egg Products. Journal of Chinese Agriculture Chemical Society 1989, 27(4), 460472.