ABSTRACT
In this study, the effects of heat treatment (45°C and 65°C, respectively) on the quality of Patinopecten yessoensis adductor muscle (PYAM) were investigated. Water mobility in PYAM samples was analyzed using low-field nuclear magnetic resonance. The texture of treated PYAM was analyzed using texture profile analysis. Protein degradation was characterized using SDS-PAGE. Activities of cathepsin L (CL), superoxide dismutase (T-SOD), glutathione peroxidase (GSH-Px), and catalase (CAT) were determined using chemical analysis methods. The production of free radicals was measured using electron spin resonance. It was revealed that water mobility in PYAM samples increased with the extension of heated time. Cohesiveness of PYAM was higher in samples heated at 65°C than at 45°C, while hardness showed an opposite trend, higher in the samples heated at 45°C than at 65°C. The degradation of structural proteins was more severe in the samples heated at 65°C than at 45°C, with the greater CL activity being observed. It was also found that heating caused elevation in T-SOD, GSH-Px, and CAT enzyme activities. Considering the chemical changes in the PYAM samples, contents of carbonyl and malonaldehyde increased, but sulfhydryl content decreased with heating. Level of free radicals increased significantly from 6 h on after heat treatment, with higher level at 65°C than at 45°C. These results suggested that oxidative stress is directly involved in quality changes during heat treatment of PYAM.
Introduction
Yesso scallop Patinopecten yessoensis is one of the most important shellfish species naturally distributed along the coasts of China, Japan, Russia, and the Korean peninsula. The total output of scallop landings has reached nearly 1.88 million tons in China.[Citation1] On a weight basis, scallops are composed of shell (52%), adductor muscle (13%), mantle lobe (9%), and gonad (ovary and testis, 3–9%).[Citation2] P. yessoensis adductor muscle (PYAM) is the main edible part, which has high nutritional value, and is traditionally regarded as a delicacy.[Citation3]
The effects of heat treatment on meat quality (e.g. the hardness, taste, flavor and nutritional value, etc.) have been widely studied.[Citation4] Different heating temperatures may cause meat proteins to undergo different degrees of denaturation and degradation (including degradation of muscle fiber protein and sarcoplasmic protein, as well as shrinking and stretching of collagens), which in turn impart products with different micro and macro qualities.[Citation5] Furthermore, heating at low temperatures (usually at 40–70°C) reduces the cooking loss compared to higher temperatures, which leads to a juicier product.[Citation6–Citation8] Studies have shown that meat is tenderer and has less cooking loss when heated at low temperature.[Citation9]
One of the effects of heat treatment on biological systems is to induce oxidative stress. Oxidative stresses are resulted from the accumulation of reactive oxygen species (ROS) beyond the capacity of the cells to quench them.[Citation10] Molecular oxygen, even though essential for most living organisms, serves as a precursor of ROS.[Citation11] At low concentrations, ROS play important roles in cell signaling processes. However, at excessive amount, they damage cellular macromolecules, such as proteins, lipids, and nucleic acids.[Citation12] ROS-induced protein damage and degradation may be a major contributor to the heat-induced changes in meat quality. After all, the sensory attributes such as tenderness, juiciness, and hardness are directly related to protein properties in meat muscles.
Similar effects of oxidative stress-induced changes in proteins may be expected in PYAM. The objective of this study was to investigate the effect of long-time low-temperature (LTLT) treatment on the chemical, physical, and textural properties of PYAM. And it was to explore the correlation between oxidative stress in PYAM and LTLT treatments by analyzing the activation of cathepsin L and changes in antioxidant enzyme activities, such as total superoxide dismutase (T-SOD), glutathione peroxidase (GSH-Px), and catalase (CAT). The findings of this study will help to reveal the key quality control mechanism to improve PYAM processing.
Materials and methods
Materials and chemicals
P. yessoensis were purchased from a local aquatic market in Dalian (China). Sodium dodecyl sulfate (SDS), N,N,N,N-tetramethylethylenediamine, Coomassie Brilliant Blue R-250, DL-dithiothreitol (DTT), and bovine serum albumin were obtained from Sangon Biotech Co., Ltd. (Shanghai, China). Malondialdehyde (MDA) assay kit, total sulfhydryl assay kit, T-SOD activity kit, CAT activity kit, and GSH-Px activity kit were purchased from Nanjing Jiancheng Bioengineering Institute (Nanjing, China). All chemicals used in this study were of analytical grade and used without further purification.
Heat treatment of PYAM samples
PYAM samples (each about 1.5 cm × 1.5 cm × 1.3 cm) were dissected on ice from P. yessoensis. Samples were then vacuum-packed and heated in water bath at 45°C and 65°C from 10 min to 32 h, respectively. All the treated PYAM samples and control (untreated) samples were quickly frozen at −80°C until further analysis.
Water content analysis
A low-field nuclear magnetic resonance (LF-NMR) (MesoMR23-060V-1, Niumag Electric Corporation, Shanghai, China) with a 0.5 T permanent magnet corresponding to a proton resonance frequency of 21.16 MHz was used to determine the water mobility described by our previous work.[Citation13] The PYAM was thawed to the room temperature, and then blotted with absorbent paper to remove excess water on their surface. Each sample was placed on the NMR bed, and a 60 mm diameter radio frequency coil was used to collect Carr–Purcell–Meiboom–Gill (CPMG) decay signals, with 90 and 180 pulses of 13 and 26 ms, respectively, and a τ-value (time between 90 and 180 pulses) of 100 ms. The data from 3000 echoes were acquired as 4 scan repetitions. The repetition time between subsequent scans was 2 s, and each measurement was performed in triplicate. Distributed multi-exponential fitting of CPMG decay curves were performed using Multi Exp Inv analysis software (Niumag Electric Corporation, Shanghai, China). The multi-exponential fitting analysis performed on the relaxation data with the SRIT software algorithm to obtain improved fitting. From the analysis, time constants for each process were calculated from the peak position, and the total area under each peak (corresponding to the proportion of water molecules exhibiting that relaxation time) was determined by cumulative integration. Moisture content was calculated as the ration of total peak area to samples mass.
Instrumental texture profile analysis (TPA)
After LTLT processing, the PYAM samples were trimmed into cylindrical pieces with height of 1.3 cm and diameter of 1.5 cm and assayed by a TA-XT2i texture analyzer (Stable Micro Systems, Surrey, UK). P/100 probe was used to perform TPA, and parameters measured were hardness and cohesiveness, which are the most important quality attributes for PYAM. Each sample underwent two cycles of compression analysis with a compression level of 60% and a compression speed of 1.0 mm/s, with 5 s relaxation time between the two cycles.
Colorimetric assay
The color (L*, a*, and b* values) of PYAM samples was measured three times on the fresh cut surface with an Ultra Scan PRO colorimeter (Hunter Lab, USA). Mean values from 10 repetitions on each LTLT treatment were calculated.
SDS-PAGE gel electrophoresis
Samples were lysed on ice in a lysis buffer (20 mM Tris–HCl (pH 7.4), 2 mM Ethylenediaminetetraacetic acid (EDTA), 2 mM Ethylene glycoltetraacetic acid (EGTA), 150 Mm NaCl, 10 mM DTT, 10 mM NaF, 1 mM Na3VO4, 1% Trion X-100, 0.1% SDS, 10 μg/mL leupeptin, 10 μg/mL aprotinin, 1 mM Phenylmethanesulfonylfluoride (PMSF) at 4°C by 1:3 (w/v). Lysates were centrifuged at 12,000 × g for 15 min, at 4°C. The supernatants were collected and diluted by SDS-PAGE sample buffer (0.25 M Tris–HCl, pH 7.4, 8 M urea, 5% SDS, 5% mercaptoethanol) by 1:1 (v/v), and then boiled for 5 min. Also, 5 μL sample was subsequently loaded onto a polyacrylamide gel made of 10% running gel and 5% stacking gel. After separation and staining, the gel was documented using Gel Capture software (DNR Bio-Imaging Systems, Jerusalem, Israel).
Cathepsin L activity assay
Cathepsin L activity was measured following reported fluorescence method.[Citation14,Citation15] Protein concentrations of sample supernatants used in the activity assays were determined using a Bradford assay.[Citation16] Results were expressed in U/mg protein where 1 unit (U) was defined as the increased fluorescence value of sample compared to blank during the reaction at 37°C.
Assessment of protein oxidation
Carbonyl and sulfhydryl groups in proteins were measured to evaluate the protein oxidation level. Carbonyl groups were estimated using the method[Citation17], with slight modifications. Five PYAM samples (about 0.9 g) were homogenized in 4.5 mL Tris buffer (pH 7.4, 50 mM, 1 mM EDTA) containing 0.01% butylated hydroxyltoluene (BHT). After centrifugation (12,000 × g, 10 min) the supernatant was incubated in darkness with 500 µL dinitrophenylhydrazine (DNPH) in 2.5 M HCl for 1 h. For each sample, a blank incubated in 400 µL 2.5 M HCl and without DNPH was run in parallel. The samples were precipitated with 20% TCA (v/v), and the pellets were washed three times with 300 µL ethanol/ethyl acetate 1:1 (v/v). The pellets were redissolved in 6 M guanidine hydrochloride at 37°C for 15 min.[Citation18] The carbonyl content was calculated from the absorbance measured at 370 nm, calculated from DNPH standard line, and expressed as nmol DNPH/mg protein.
Total sulfhydryl content was determined by using commercially available kits. The PYAM sample were homogenized in 0.9% NaCl solution at 4°C by 1:9 (w/v). The homogenate was centrifuged at 4000 rpm for 20 min at 4°C and the supernatant was collected. All procedures completely complied with the manufacture’s instructions. Sulfhydryl content was then calculated from by absorbance at 405 nm, after reacting with 5,5'-dithiobis (2-nitrobenzoic acid) to produce a yellow compound. Values of sulfhydryl level were expressed as nmol sulfhydryl/mg protein.
Assessment of lipid oxidation
The content of MDA was determined by using commercially available kits. Samples were homogenized in ice cold phosphate buffer (10 mM sodium phosphate at pH 7.0, 2% SDS, 0.01% BHT) at 4°C by 3:20 (w/v). The homogenates were centrifuged at 12,000 × g for 10 min at 4°C. The supernatants were collected and frozen at −30°C. MDA content was measured at a wavelength of 532 nm by reacting with thiobarbituric acid to form a stable chromophoric product.[Citation19] Values were expressed as nmol MDA/mg protein extracted.
Antioxidant enzymes activities assay
SOD activity measurement was performed according to the manufacture’s instructions. The activities of enzymes were expressed as nano units per milligram protein. The assay of SOD activity was based on its ability to inhibit the oxidation of hydroxylamine by O2−∙ produced from the xanthine–xanthineoxiase system. One unit of SOD activity was defined as the amount reducing the absorbance at 550 nm by 50%. CAT activity was measured, according to Greenwald[Citation20] following the decomposition of H2O2. The assay was carried out at 240 nm in 100 mM Na-phosphate buffer pH 7.0 with 12 mM H2O2. GPx activities were measured followed Regoli et al.’s procedure[Citation21] in a coupled enzyme system where the GSSG formed in the GPx reaction was converted to the reduced form by glutathione reductase.
Electron spin resonance (ESR) spectroscopy
A modified version of an ESR-based assay[Citation22] was used for evaluation of the ROS production in the PYAM. PYAM samples were homogenized with 50 mM phosphate buffer (pH 5.7) containing 40 mM water-soluble spin trap α-(4-pyridyl n-oxide)-n-tert-butylnitrone (POBN, TCI). The PYAM emulsion with POBN was treated at 55°C and at selected times, and then the samples were centrifuged at 12,000 × g for 10 min at 4°C. The supernatants were transferred immediately into a glass capillary tube (Blaubrandintra MARK, Brand, Germany), and at room temperature ESR spectra were recorded using ESR spectrometer A200 (Bruker, Karisruhe, Germany). ESR conditions were as follows: microwave power of 1.99 mW, sweep width 50 Gauss, modulation frequency 100 kHz, modulation amplitude 1.0 Gauss, conversion time 360 ms, time constant 5242.88 ms, and number of scans per sample was three. The trapping of ROS in samples by POBN gave rise to adduct with six-line ESR-spectra (triplets of doublets). The signal height of the first line of the center field doublet was used as a measure of the relative concentration of ROS adducts formed in samples at a given time.
Statistical analysis
Statistical analysis was conducted with SPSS statistics program (version 16.0) (SPSS Inc., 2001, Chicago, IL, USA). Differences between means were evaluated by one-way analysis of variance, and p values <0.05 were considered significant.
Results and discussion
Changes of physical, chemical, and textural properties of PYAM during heat treatment
shows the results of changes in moisture content, TPA attributes, and color. As shown in , samples treated at 65°C had significantly lower moisture contents compared to samples treated at 45°C for the same time. No changes in moisture content of PYAM treated at 45°C was observed before 4 h; however, with the time prolonging, the values significantly decreased from 4 to 32 h. For the samples treated at 65°C, the moisture content obviously decreased in the whole heating treatment. This result was consistent with the increasing cook loss of skeletal muscle in pork[Citation23] and beef[Citation8] by heat treatment.
Figure 1. Changes of quality-related events in Patinopecten yessoensis adductor muscle (PYAM) during the heat treatment of 45°C and 65°C. (A) Moisture content; (B) hardness; (C) cohesiveness; (D) L*; (E) a*; (F) b*. Data is reported as mean ± SD based on 10 replicates. Different letters indicated significant differences (p < 0.05).
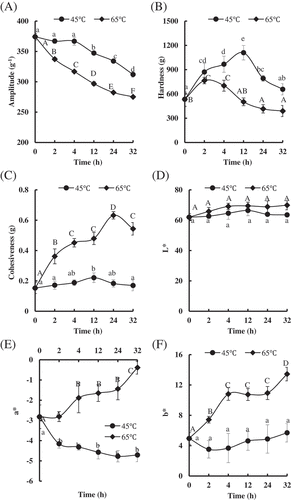
The effects of heat treatment on hardness and cohesiveness in the PYAM are shown in and 1C, respectively. The values of harLJFP_A_1476379dness and cohesiveness increased during early stage of thermal treatment, and then decreased down to the original level or even lower as heating continued at 45°C and 65°C. The hardness value of PYAM treated at 65°C reached the maximum value much faster than the sample treated at 45°C (2 h vs. 12 h), and the maximum hardness seemed to be negatively correlated to heating temperature, which was, the higher the temperature, the lower the maximum hardness (i.e. 1109.82 ± 89.39 g at 45°C vs. 765.46 ± 39.03 g at 65°C). Furthermore, the opposite trend was observed in cohesiveness, where lower temperature seemed to bring the samples to their maximum cohesiveness faster, but the maximum value itself was lower (45°C, 0.22 ± 0.03 g at 12 h vs. 65°C, 0.63 ± 0.02 g at 24 h). A similar phenomenon that hardness increased at an early stage and decreased over time in instant scallop during storage at 20–40°C was observed.[Citation24]
Another important quality parameter for PYAM is color. The color indexes (L*, a*, b*) are shown in , respectively. Neither temperature nor time affected the L* values (lightness) of PYAM. However, temperature did affect a* value (redness) and b* value (yellowness) significantly. At 65°C heating, a* and b* showed similar trend of rapid increasing in hours 0–4, stabilizing in hours 4–24, and another round of increasing in hours 24–32. At 45°C on the other hand, an almost opposite trend was observed for both a* and b*, with an initial rapid drop in hours 0–2, followed by not much change as time continues. Color changes in heat-treated PYAM are related to the denaturation of myoglobin[Citation25; these observations suggest that myoglobin in PYAM may undergo different changes under different heating conditions.
Changes of protein degradation and cathepsin L activity during heat treatment
Previous study indicated that changes in meat juiciness and tenderness during cooking are attributed to heat-induced denaturation and shrinkage of myofibrillar proteins and collagens.[Citation26] And the water loss mostly comes from water being expelled by protein denaturation and contraction.[Citation27] Therefore, we investigated the protein degradation of PYAM heated at 45°C and 65°C, respectively (A and 2B). The protein pattern of raw PYAM sample partially agreed with Goetz and Whisman,[Citation28] with more protein bands identified. Heat treatment at 45°C led to gradual reduction in densities of the protein bands of 208 kDa (myosin heavy chain (MHC)) and 97 kDa (paramyosin), especially after 6 h. The densities of actin bands (43 kDa) increased within the first two hours, and then stabilized until 32 h. In comparison, much rapid changes were observed in PYAM samples heated at 65°C. Bands of MHC and proteins of molecular mass of 35–40 kDa started to disappear at 2 h, and the paramyosin band was nearly invisible after only 10 min. It is believed that both textural and color changes in heat-treated PYAM are related to the denaturation of myoglobin.[Citation25] These results were consistent with their earlier report.
Figure 2. Changes in protein degradation and endogenous enzyme activities in Patinopecten yessoensis adductor muscle (PYAM) during the heat treatment of 45°C and 65°C. (A) SDS-PAGE photographs of protein degradation under the heat treatment of 45°C; (B) SDS-PAGE photographs of protein degradation under the heat treatment of 45°C; 5 μL sample was loaded in 10% (w/v) SDS-PAGE gel; HM: high MARK; LM: low MARK; (C) cathepsin L activity measured by a fluorospectrophotometer. Data is reported as mean ± SD based on three replicates. Different letters indicated significant differences (p < 0.05).
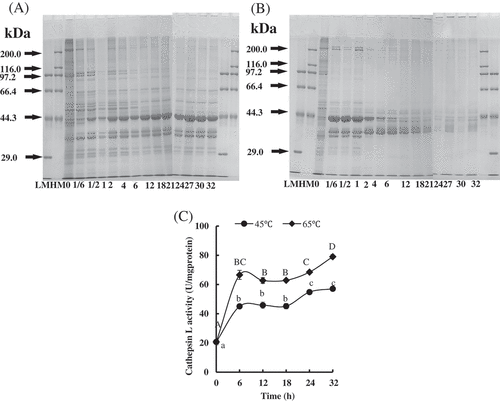
It has been reported that cathepsin L plays a key role in the proteolysis of sea cucumber, a traditional ocean animal usually consumed by Asian people.[Citation29] To assess whether it is also the case for PYAM, cathepsin L activity was measured. Overall, cathepsin L activity rose rapidly within the first six hours of heat treatment, regardless of temperature, and the activity leveled off between 6 and 18 h. It rose again after 18 h, until maximum reached at 32 h. Furthermore, higher temperature induced higher cathepsin L activity, with the maximum value of 57.09 ± 1.57 and 79.05 ± 1.53 at 32 h, respectively, for 45°C and 65°C. Cathepsin L is a endopeptidase that is inside the lysosomes of muscle cells. They catalyze hydrolysis of internal peptide bonds of proteins when they are activated.[Citation30] In our results, cathepsin L was released from lysosome and degraded the PYAM protein structure. The optimal reaction temperature of cathepsin L is about 55–65°C,[Citation31] which is also one of the reasons that cathepsin L at 65°C is higher than that at 45°C.
Oxidants production during heat treatment
The level of protein oxidation was quantified by measuring the contents of carbonyl and sulfhydryl. As shown in , the carbonyl content in the PYAM was significantly increased in the first six hours of heating regardless of the temperature. It leveled off in 6–12 h, and rose again from 12 to 27 h, until maximum values were reached at 1.79 ± 0.07 nmol/mg protein and 2.45 ± 0.22 nmol/mg protein, respectively, for 45°C and 65°C. Traore et al.[Citation32] observed a significant increasing in carbonyl content from pork longissimus thoracis muscle after heating. Santélhoutellier et al.[Citation33] also found a threefold increase in carbonyl content from bovine myofibrillar proteins after cooking (100°C, 45 min). Consistent with this report, an increase of nearly sevenfold in carbonyl level was detected in PYAM after 27 h heating (). It indicated that massive amounts of amino acid residues like arginine, proline, lysine in PYAM are converted to carbonyl derivatives like α-aminoadipic (AAS) and γ-glutamic semialdehydes (GGS).[Citation34]
Figure 3. Changes in oxidation in Patinopecten yessoensis adductor muscle (PYAM) during the heat treatment of 45°C and 65°C. (A) Carbonyl content; (B) total sulfhydryl group content; (C) MDA content. They were detected by a spectrophotometry. Data is reported as mean ± SD based on three replicates. Different letters indicated significant differences (p < 0.05).
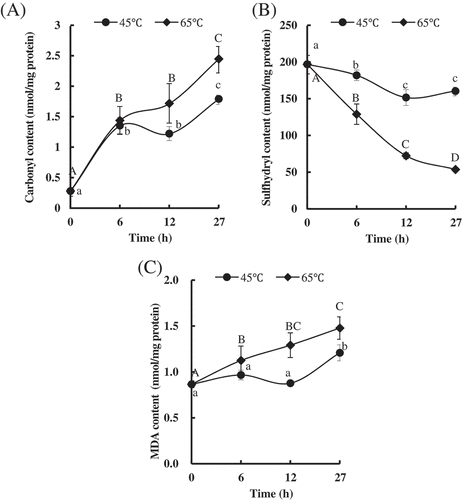
Sulfhydryl contents of PYAM treated at 45°C and 65°C are shown in . Compared with PYAM heated at 45°C, samples heated at 65°C showed significantly lower sulfhydryl content for the same time. Sulfhydryl content dropped as heating went on; for samples treated at 65°C, the drop continues throughout the process, while for samples heated at 45°C, the sulfhydryl content stabilizes after 12 h. Similar phenomena to sulfhydryl decreasing at both 45°C and 65°C were observed in threadfin bream (Nemipterus bleekeri) actomyosin.[Citation35] Furthermore, Ko et al.[Citation36] also found that the reactive sulfhydryl of tilapia actomyosin would decline by being oxidized to disulfides in inter- and intra-molecules.
Except for protein oxidation, lipid peroxidation was also evaluated by MDA quantification, as shown in . For 45°C, initially no significant changes of the MDA content were observed in PYAM within 12 h, but eventually a increase was seen in MDA value (from 0.87 ± 0.04 nmol/mg protein to 1.21 ± 0.09 nmol/mg protein) by hours 27. In contrast, the MDA content of PYAM gradually increased when treated at 65°C and reached the maximum value of 1.48 ± 0.12 nmol/mg protein after 27 h. The increased MDA concentration was considered to be an indicator of increasing oxidative damage levels. The increased MDA concentrations were considered to be a consequence of increasingly oxidative damage levels.
Changes of T-SOD, CAT and GSH-Px activities during heat treatment
Activities of the three antioxidant enzymes, T-SOD, CAT, and GSH-Px, were studied. Increasing temperature from 45°C to 65°C did not affect the T-SOD activity until 27 h of processing time. With the time prolonging, no obvious change was found in T-SOD activities of PYAM heated at 45°C. However, the significant difference was observed until 27 h compared to the raw samples (). By increasing temperature from 45°C to 65°C significantly decreased CAT activities at all time points (). The CAT activities significantly decreased after PYAM were treated at 45°C or 65°C for 6 h and then remained same level, respectively. Neither temperature nor time significantly affected the GSH-Px activities of PYAM treated at 45°C or 65°C before 12 h (). Until 27 h, the GSH-Px activities were significantly decreased at 45°C and 65°C. These results suggested that the CAT activity was most sensitive to treated temperature, and the GSH-Px activity was more sensitive to time than the T-SOD activity. Abele et al.[Citation37] suggested that bivalves produce ROS (such as O2∙- and H2O2) and activate antioxidative defense systems under acute temperature stress. SOD is the first and most important enzymatic defense factor among these antioxidants. SOD catalyzes the disproportionation of O2∙- to H2O2 and O2 and, in turn, H2O2 is converted by CAT into H2O and O2 in cells. A significant decrease was found in SOD, CAT, and GSH-Px activities, consistent with temperature elevation being followed by an increase in ROS generation,[Citation38] which may be a signal to active protective reactions. Therefore, we thought that heating treatment induced ROS production and oxidative stress appearing in the PYAM.
Figure 4. Changes in antioxidant enzymes in Patinopecten yessoensis adductor muscle (PYAM) during the heat treatment of 45°C and 65°C. (A) Total superoxide dismutase activity; (B) catalase activity; (C) GSH-Px activity. Data is reported as mean ± SD based on three replicates. Different letters indicated significant differences (p < 0.05).
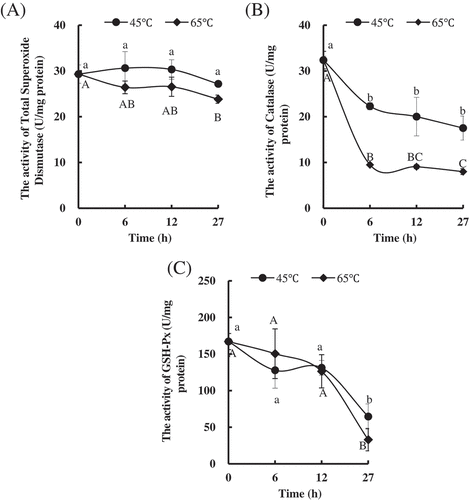
ROS production during heat treatment
The ROS content of the PYAM during heat treatment was evaluated by ESR spin trapping. and 5B suggest the ESR spectra of the observed spin adducts in PYAM processed at 45°C and 65°C, respectively. The ESR spectra had hyperfine coupling constants (aN = 15.8 G, aH = 2.6 G), which are typical values for spin adducts formed by addition of carbon-centered radicals to POBN.[Citation39] It indicated that ROS centered by carbon were produced in the heat-treated PYAM. The ROS level is shown in . The ROS content of PYAM treated at 45°C significantly increased after 6 h, while the ROS content of samples treated at 65°C started obvious increasing after 6 h and rapidly increased up to the maximum value at 27 h, which was higher by more than fourfold compared to the value of samples treated at 45°C at 27 h.
Figure 5. Changes in production of free radicals in Patinopecten yessoensis adductor muscle (PYAM) during the heat treatment of 45°C and 65°C. (A) ESR spectra of the PYAM sample homogenate with 40 mM POBN during the heat treatment of 45°C; (B) ESR spectra of the PYAM sample homogenate with 40 mM POBN during the heat treatment of 65°C; (C) diagram of ESR absorption of the PYAM sample homogenate with 40 mM POBN. (a) 0 h, (b) 1/6 h, (c) 4 h, (d) 6 h, (e) 12 h, (f) 24 h, and (g) 27 h. Different letters mean differences (p < 0.05).
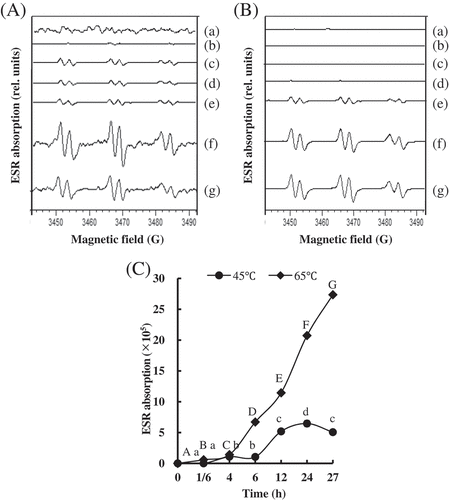
Both protein oxidation and lipid peroxidation are believed to be via a free radical chain reaction.[Citation40,Citation41] The abstraction of a hydrogen atom by a ROS leads to the generation of a carbon-centered radical. Similar results were reported on processed pork,[Citation42] thermal treated beef and chicken.[Citation43] Moreover, free radicals formation and carbonyl groups’ increase were detected on salted herrings during ripening,[Citation22] which was consistent with our results. It was also reported that the generation mechanism of specific carbonyl derivatives like AAS and GGS was initiated by ROS and transition metal ions.[Citation44]
Conclusion
The quality of PYAM could be affected variously by heating at 45°C than at 65°C, which included moisture content, texture, and color. The higher temperature could induce more severe degradation of structural proteins and stronger activity of CL. Meanwhile, the level of oxidation showed in the content of carbonyl, MDA, sulfhydryl, and free radicals was increased with the raising temperature in the PYAM. Therefore, ROS-induced oxidation was an important reason to damage the PYAM quality during heat treatment. Oxidative stress was involved in the PYAM during the heat treatment.
Additional information
Funding
References
- Fisheries and Aquaculture Department. (2016) http://www.fao.org/figis/servlet/SQServlet?file=/usr/local/tomcat/8.5.16/figis/webapps/figis/temp/hqp_6923670017345227323.xml&outtype=html (accessed April 17, 2018).
- Oyamada, C.; Kaneniwa, M.; Ebitani, K.; Murata, M.; Ishihara, K. Mycosporine-Like Amino Acids Extracted from Scallop (Patinopecten Yessoensis) Ovaries: UV Protection and Growth Stimulation Activities on Human Cells. Marine Biotechnology 2008, 10(2), 141–150. DOI: 10.1007/s10126-007-9043-z.
- Wu, H. T.; Jin, W. G.; Sun, S. G.; Li, X. S.; Duan, X. H.; Li, Y.; Zhu, B. W. Identification of Antioxidant Peptides from Protein Hydrolysates of Scallop (Patinopecten Yessoensis) Female Gonads. European Food Research and Technology 2016, 242(5), 713–722. DOI: 10.1007/s00217-015-2579-7.
- Xiong, Y. L. Protein Oxidation and Implications for Muscle FoodQuality. In Eric A. Decker, Cameron Faustman, Clemente J. Lopez-Bote Antioxidants in Muscle Foods Nutritional Strategies to ImproveQuality; Wiley and Sons: New York, 2000; 85–90.
- García-Segovia, P.; Andrés-Bello, A.; Martínez-Monzó, J. Effect of Cooking Method on Mechanical Properties, Color and Structure of Beef Muscle (M. Pectoralis). Journal of Food Engineering 2007, 80(3), 813–821. DOI: 10.1016/j.jfoodeng.2006.07.010.
- Aaslyng, M. D.; Bejerholm, C.; Ertbjerg, P.; Bertram, H. C.; Andersen, H. J. Cooking Loss and Juiciness of Pork in Relation to Raw Meat Quality and Cooking Procedure. Food Quality & Preference 2003, 14(4), 277–288. DOI: 10.1016/S0950-3293(02)00086-1.
- Christensen, L.; Gunvig, A.; Tørngren, M. A.; Aaslyng, M. D.; Knøchel, S.; Christensen, M. Sensory Characteristics of Meat Cooked for Prolonged Times at Low Temperature. Meat Science 2012, 90(2), 485–489. DOI: 10.1016/j.meatsci.2011.09.012.
- Vaudagna, S. R.; Sánchez, G.; Neira, M. S.; Insani, E. M.; Picallo, A. B.; Gallinger, M. M.; Lasta, J. A. Sous Vide Cooked Beef Muscles: Effects of Low Temperature-Long Time (LT-LT) Treatments on Their Quality Characteristics and Storage Stability. International Journal of Food Science and Technology 2002, 37(4), 425–441. DOI: 10.1046/j.1365-2621.2002.00581.x.
- Bejerholm, C.; Aaslyng, M. D. The Influence of Cooking Technique and Core Temperature on Results of a Sensory Analysis of Pork-Depending on the Raw Meat Quality. Food Quality and Preference 2004, 15, 19–30. DOI: 10.1016/S0950-3293(03)00018-1.
- Lesser, M. P.;. Oxidative Stress in Marine Environments: Biochemistry and Physiological Ecology. Annual Review of Physiology 2006, 68(1), 253–278. DOI: 10.1146/annurev.physiol.68.040104.110001.
- Chuang, S. C.; Chen, J. H. Photooxidation and Antioxidant Responses in the Earthworm Amynthas Gracilis Exposed to Environmental Levels of Ultraviolet B Radiation. Comparative Biochemistry and Physiology, Part A 2013, 164, 429–437. DOI: 10.1016/j.cbpa.2012.11.006.
- Stadtman, E. R.;. Oxidation of Free Amino Acids and Amino Acid Residues in Proteins by Radiolysis and by Metal-Catalyzed Reactions. Annual Review of Biochemistry 1993, 62, 797–821. DOI: 10.1146/annurev.bi.62.070193.004053.
- Dong, X. P.; Li, Y.; Song, L.; Wang, Y.; Tan, M. Q.; Zhu, B. W. Changes of Water Distribution and Physicochemical Properties of Abalone (Haliotis Discus) Myofibrillar Proteins during Heat‐Induced Gelation. Journal of Food Processing & Preservation 2017, 41, e13069. DOI: 10.1111/jfpp.2017.41.issue-4.
- Dong, X. F.; Zhang, J. Y.; Xi, Q.; Liu, L.; Li, N.; Fu, H.; Qi, H. Photooxidation and Antioxidant Responses in the Gut of Sea Cucumber Stichopus Japonicus Autolysis Exposed to UVC Radiation. Journal of Food Agriculture & Environment 2014, 12, 207–211.
- Qi, H.; Fu, H.; Dong, X. F.; Feng, D. D.; Li, N.; Wen, C.; Zhu, B. Apoptosis Induction Is Involved in UVA-induced Autolysis in Sea Cucumber. Stichopus Japonicus. Journal of Photochemistry & Photobiology B Biology 2016, 158, 130–135. DOI: 10.1016/j.jphotobiol.2016.02.034.
- Bradford, M. M.;. A Rapid and Sensitive Method for the Quantitation of Microgram Quantities of Protein Utilizing the Principle of Protein-Dye Binding. Analytical Biochemistry 1976, 72(s 1–2), 248–254. DOI: 10.1016/0003-2697(76)90527-3.
- Oliver, C. N.; Ahn, B. W.; Moerman, E. J.; Goldstein, S.; Stadtman, E. R. Age-Related Changes in Oxidized Proteins. Journal of Biological Chemistry 1987, 262, 5488–5491.
- Zakrys-Waliwander, P. I.; O’Sullivan, M. G.; O’Neill, E. E.; Kerry, J. P. The Effects of High Oxygen Modified Atmosphere Packaging on Protein Oxidation of Bovine M. Longissimus Dorsi Muscle during Chilled Storage. Food Chemistry 2012, 131(2), 527–532. DOI: 10.1016/j.foodchem.2011.09.017.
- Lepperblilie, A. N.; Berg, E. P.; Buchanan, D. S.; Keller, W. L.; Maddockcarlin, K. R.; Berg, P. T. Effectiveness of Oxygen Barrier Oven Bags in Low Temperature Cooking on Reduction of Warmed-Over Flavor in Beef Roasts. Meat Science 2014, 96(96), 1361–1364. DOI: 10.1016/j.meatsci.2013.11.012.
- Greenwald, R. A.; Handbook of Methods for Oxygen Radical Research; CRC Press: Boca Raton, FL, 1985
- Regoli, F.; Nigro, M.; Orlando, E. Lysosomal and Antioxidant Responses to Metals in the Antarctic Scallop. Adamussium Colbecki. Aquatic Toxicology 1998, 40(4), 375–392. DOI: 10.1016/S0166-445X(97)00059-3.
- Christensen, M.; Andersen, E.; Christensen, L.; Andersen, M. L.; Baron, C. P. Textural and Biochemical Changes during Ripening of Old-Fashioned Salted Herrings. Journal of the Science of Food and Agriculture 2011, 91(2), 330–336. DOI: 10.1002/jsfa.v91.2.
- Becker, A.; Boulaaba, A.; Pingen, S.; Röhner, A.; Klein, G. Low Temperature, Long Time Treatment of Porcine M. Longissimus Thoracis Et Lumborum in a Combi Steamer under Commercial Conditions. Meat Science 2015, 110, 230–235. DOI: 10.1016/j.meatsci.2015.07.024.
- Li, W.; Wang, J.; Sun, J.; Li, W.; Wang, Y.; Zhang, G. Shelf Life Prediction Modeling of Vacuum-Packaged Scallops on the Kinetics of Total Volatile Base Nitrogen. International Journal of Food Engineering 2011, 7, 457–461.
- Christensen, L.; Ertbjerg, P.; Aaslyng, M. D.; Christensen, M. Effect of Prolonged Heat Treatment from 48°C to 63°C on Toughness, Cooking Loss and Color of Pork. Meat Science 2011, 88(2), 280–285. DOI: 10.1016/j.meatsci.2010.12.035.
- Sánchez, D. P. J.; Gázquez, A.; Ruiz-Carrascal, J. Physico-Chemical, Textural and Structural Characteristics of Sous-Vide Cooked Pork Cheeks as Affected by Vacuum, Cooking Temperature, and Cooking Time. Meat Science 2012, 90(3), 828–835. DOI: 10.1016/j.meatsci.2011.11.024.
- Kondjoyan, A.; Oillic, S.; Portanguen, S.; Gros, J. B. Combined Heat Transfer and Kinetic Models to Predict Cooking Loss during Heat Treatment of Beef Meat. Meat Science 2013, 95(2), 336–344. DOI: 10.1016/j.meatsci.2013.04.061.
- Goetz, D. W.; Whisman, B. A. Occupational Asthma in a Seafood Restaurant Worker: Cross-Reactivity of Shrimp and Scallops. Annals of Allergy Asthma and Immunology 2001, 85(6 Pt 1), 461–466. DOI: 10.1016/S1081-1206(10)62572-0.
- Zhou, D. Y.; Chang, X. N.; Bao, S. S.; Song, L.; Zhu, B. W.; Dong, X. P.; Liu, Y. X. Purification and Partial Characterisation of a Cathepsin L-Like Proteinase from Sea Cucumber (Stichopus Japonicus) and Its Tissue Distribution in Body Wall. Food Chemistry 2014, 158(8), 192–199. DOI: 10.1016/j.foodchem.2014.02.105.
- Agarwal, S. K.;. Proteases Cathepsin_S — A View. Biochemical Education. 2010, 18(2), 67–72. DOI: 10.1016/0307-4412(90)90176-O.
- Zhu, B. W.; Zhao, L. L.; Sun, L. M.; Li, D. M.; Murata, Y.; Yu, L.; Zhang, L. Purification and Characterization of a Cathepsin L-Like Enzyme from the Body Wall of the Sea Cucumber. Stichopus Japonicus. Bioscience Biotechnology & Biochemistry 2008, 72(6), 1430–1437. DOI: 10.1271/bbb.70741.
- Traore, S.; Aubry, L.; Gatellier, P.; Przybylski, W.; Jaworska, D.; Kajak-Siemaszko, K.; Santé-Lhoutellier, V. Effect of Heat Treatment on Protein Oxidation in Pig Meat. Meat Science 2012, 91(1), 14–21. DOI: 10.1016/j.meatsci.2011.11.037.
- Santélhoutellier, V.; Astruc, T.; Marinova, P.; Greve, E.; Gatellier, P. Effect of Meat Cooking on Physicochemical State and in Vitro Digestibility of Myofibrillar Proteins. Journal of Agricultural & Food Chemistry 2008, 56(4), 1488–1494. DOI: 10.1021/jf072999g.
- Roldan, M.; Antequera, T.; Armenteros, M.; Ruiz, J. Effect of Different Temperature-Time Combinations on Lipid and Protein Oxidation of Sous-Vide Cooked Lamb Loins. Food Chemistry 2014, 149(149), 129–136. DOI: 10.1016/j.foodchem.2013.10.079.
- Yongsawatdigul, J.; Park, J. W. Thermal Denaturation and Aggregation of Threadfin Bream Actomyosin. Food Chemistry 2003, 83(3), 409–416. DOI: 10.1016/S0308-8146(03)00105-5.
- Ko, W. C.; Yu, C. C.; Hsu, K. C. Changes in Conformation and Sulfhydryl Groups of Tilapia Actomyosin by Thermal Treatment. LWT - Food Science and Technology. 2007, 40(8), 1316–1320. DOI: 10.1016/j.lwt.2006.10.002.
- Downs, C. A.; Fauth, J. E.; Woodley, C. M. Assessing the Health of Grass Shrimp (Palaeomonetes Pugio) Exposed to Natural and Anthropogenic Stressors: A Molecular Biomarker System. Marine Biotechnology 2001, 3(4), 380–397. DOI: 10.1007/s10126-001-0008-3.
- Boveris, A.; Martino, E.; Stoppani, A. O. Evaluation of the Horseradish Peroxidase-Scopoletin Method for the Measurement of Hydrogen Peroxide Formation in Biological Systems. Analytical Biochemistry 1977, 80(1), 145–158. DOI: 10.1016/0003-2697(77)90634-0.
- Buettner, G. R.;. Spin Trapping: ESR Parameters of Spin Adducts. Free Radical Biology & Medicine 1987, 3(3), 259–303. DOI: 10.1016/S0891-5849(87)80033-3.
- Caroline, P. B.; Henrik, J. A. Myoglobin-Induced Lipid Oxidation. A Review. Journal of Agricultural & Food Chemistry 2002, 50(14), 3887–3897. DOI: 10.1021/jf011394w.
- Michael, J. D.;. Protein Oxidation and Peroxidation. Biochemical Journal 2016, 473(7), 805–825. DOI: 10.1042/BJ20151227.
- Carlsen, C. U.; Andersen, M. L.; Skibsted, L. H. Oxidative Stability of Processed pork-Assay Based on ESR-detection of Radicals. European Food Research and Technology 2001, 213(3), 170–173. DOI: 10.1007/s002170100357.
- Ma, H. J.; Ledward, D. A.; Zamri, A. I.; Frazier, R. A.; Zhou, G. H. Effects of High Pressure/Thermal Treatment on Lipid Oxidation in Beef and Chicken Muscle. Food Chemistry 2007, 104(4), 1575–1579. DOI: 10.1016/j.foodchem.2007.03.006.
- Lund, M. N.; Heinonen, M.; Baron, C. P.; Estévez, M. Protein Oxidation in Muscle Foods: A Review. Molecular Nutrition & Food Research 2011, 55(1), 83–95. DOI: 10.1002/mnfr.201000453.