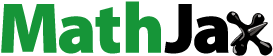
ABSTRACT
In this article, the characteristics and structure of rabbit skin gelatin (RG) films were measured and compared with porcine skin gelatin (PG) films. The RG film was 8–10 μm thinner than that of PG film. RG films had better resistance to water and lower water solubility than did the PG film with the same gelatin and glycerol ratio due to the difference in their amino acid composition. The two types of gelatin films were almost transparent, which could give food a good appearance quality if these are used as packaging films. Both showed excellent barrier properties against UV light and could prevent the lipid oxidation reaction induced by ultraviolet light in the food system. The RG and PG films showed similar trends in mechanical properties as the change of their components. In general, the rigidity of the RG films was slightly lower than that of the PG films, but the flexibility was more prominent. This was due to intense interaction between gelatin molecules and glycerol molecules in RG films, but the dominant interaction was between the gelatin molecules in the PG films. The surfaces and cross-section microstructures of the RG and PG films were smooth and homogeneous, however for the RG films were more compact compared with the PG films.
Introduction
Plastic products made from petroleum-based polymeric materials are widely used in food packaging due to their good structural properties, water and gas barrier properties, low production cost and unique aesthetic properties.[Citation1] However, these materials have some disadvantages, for example, they are difficult to biodegrade, and they are synthesised from a non-renewable source.[Citation2]
There is growing interest in biodegradable packaging materials for solving the problem of environmental pollution caused by plastic packaging products, and scholars are attempting to use biodegradable packaging materials to replace plastic materials. Natural biopolymers have been increasingly gaining attention in the manufacturing of biodegradable films because of their biocompatibility and biodegradability.[Citation3]
Proteins and polysaccharides are two types of macromolecules that are often used to prepare biodegradable membranes. Proteins as homopolymers have a unique structure (based on 20 different monomers) that is contrary to polysaccharides, which confers a wider range of potential functions in natural biopolymers, especially high intermolecular binding potential. [Citation4] Thus, the mechanical and barrier properties of protein-based films are better than those of polysaccharide-based films. [Citation5] Gelatin is a soluble protein derived from the partial hydrolysis of collagen that has attracted attention for the development of biodegradable films due to its abundance, biodegradability and excellent film-forming properties.[Citation6–Citation8] Gelatin has been the most popular studied material for the preparation of biodegradable packaging films because it could be used as an outer coating to protect food against drying, light and oxygen.[Citation9]
Ahmad et al.[Citation10] prepared gelatin films incorporated with lemongrass essential oil (LEO) to wrap sea bass slices, and they found lower changes in colour, K value and total volatile base nitrogen in LEO film-wrapped samples compared with the control. Jang et al.[Citation11] found that strawberries packaged with rapeseed protein-gelatin films have lower amounts of yeast and moulds, better sensory scores and longer shelf life than the control. Antoniewski et al.[Citation12] utilised gelatin films as a coating for fresh meat and discovered a reduction in the colour deterioration for gelatin-coated fresh meat compared with the control because gelatin films act as an oxygen barrier. Benjakul et al.[Citation13,Citation14]found that fish gelatin-based films incorporated with palm oil, basil essential oil and epigallocatechin gallate could inhibit the oxidation of some food components during storage.
Most commercial gelatins are currently sourced from skin and bones of pigs or cows.[Citation15] With the emergence of bovine spongiform encephalopathy (BSE) and due to religious beliefs, the application and safety of traditional mammalian gelatin have been restricted and argued for many years. There has been great interest in gelatin alternatives, and among them, aquatic gelatin could be a potential alternative because of rich raw material resources.[Citation6,Citation16,Citation17] However, compared with porcine or bovine gelatin film, aquatic gelatin film has poorer mechanical properties and higher water solubility [Citation18–Citation20] since their lower amounts of proline and hydroxyproline.[Citation21–Citation23] Therefore, it is necessary to find a potential alternative of traditional mammalian source to prepare high-quality gelatin films.
Rabbit skin is a major by-product of rabbit meat processing industries, and discarding it could cause waste and environmental pollution. As an alternative to traditional mammalian gelatin, rabbit skin gelatin has fewer restrictions on its consumption in the religious and social domain compared with pork and cattle. Yu et al. reported on the amino acid composition and gel properties of rabbit skin gelatin were similar to pork and cattle.[Citation24] Thus, rabbit skin could be a better source of gelatin to replace mammalian sources compared with aquatic resources. However, the characteristics and structure of RG films have not been reported in previous research.
The purpose of this study is to evaluate whether RG film could be a potential alternative of PG film by comparing their properties. RG films was prepared, and their thickness, water vapour permeability, water solubility, transparency and mechanical properties were investigated and compared with PG films. Furthermore, Fourier transform infrared (FT-IR) spectroscopy and scanning electron microscopy (SEM) of RG films were also investigated and compared to determine the difference of their microstructure.
Materials and methods
Materials
Fresh rabbit skin was generously provided by A Xing Ji Industry Co., Ltd. (Chongqing, China) and stored at −20°C before use. Porcine skin gelatin (Type A, powder, gel strength-300 g Bloom) was purchased from Sigma-Aldrich International GmbH (St. Louis, MO 63103, USA). Sodium dodecyl sulfate (SDS), Tris, ammonium persulfate, β-mercaptoethanol (2-ME), Coomassie Blue R-250 and N,N,N’,N’-tetramethylethylenediamine (TEMED) were procured from Bio-Rad Laboratories (Hercules, CA, USA). Glycine, glycerol and bromophenol blue were obtained from Sangon Biotech Co., Ltd. (Shanghai, China), and 30% acrylamide (the mass ratio of acrylamide to bis-acrylamide was 29:1) was obtained from Beijing Solarbio Science and Technology Co., Ltd. (Beijing, China). Page Ruler TM Unstained Protein Ladder was purchased from Fermentas (Beijing, China). All of the other chemicals were of analytical grade.
Extraction of rabbit skin gelatin
The rabbit’s hair was removed and gelatin was extracted from rabbit skin according to the method from Yu et al.[Citation24] and Ma et al.[Citation25] with slight modifications. After removing hair, the rabbit skin was pre-treated for 5 min at room temperature in 1% (w/v) hydrochloric acid at a skin/solution ratio of 1:6 (w/v) with discontinuous stirring. Subsequently, the samples were washed thoroughly with distilled water until the washing water was approximately neutral. The pretreated rabbit skin was mixed with distilled water at 60°C for 6 h in a skin/water ratio of 1:3 (w/v) in a SHZ-B thermostat water bath oscillator (Jiangren Experimental Equipment Co., Ltd., Shanghai, China) to extract the gelatin. The mixture was then filtered and centrifuged at 12,800 g for 20 min using a 5810 Centrifugal Machine (Eppendorf AG, Hamburg, Germany). To improve the purity of the gelatin, a 6% siliceous earth solution was added to the gelatin solution at a volume ratio of 1:50 (siliceous earth: gelatin), followed by vacuum filtration using a microfiltration membrane that was 1.2 μm in size. The solution was dried under vacuum (0.07 MPa) at 50°C until the moisture level was below 15%.
Film preparation
The film-forming solution (FFS) was prepared by dissolving 4 g or 5 g of gelatin powder in 100 mL of distilled water with a designated amount of glycerol (22% or 30% of 4 g of gelatin, and 30% of 5 g of gelatin, respectively) and then incubated at 60°C in a water bath (DZKW-D-2, Yongguangming Co., Ltd, Beijing, China) with slight stirring. The film-forming solution (FFS) (30 mL) was cast onto a disposable plastic petri dish (13 × 13 cm2) and dried in an electric heat drum wind drying oven (DGG-9140A, Zhejiang, China) at 45 ± 1°C for 24 h. The dry films were manually peeled off and stored in a desiccator for 48 h and then subjected to the following analyses.
Characterisation of the gelatin films
Film thickness
The thickness of the film was measured using a micrometer caliper (Shanghai Chuan Lu Measuring Tool Co., Ltd, China) with an accuracy of 0.001 mm. Ten random positions of each film for three films were used for thickness determination, and the mean values were calculated.
Water vapour permeability (WVP)
WVP of the films was measured using a water vapour permeability testing system (W3/060, Labthink Co., Ltd, Shandong, China). The relative humidity and test temperature were 60% and 25°C, respectively. Six films of each sample were tested.
Colour
The colour parameters, Lightness, Redness and Yellowness, were measured using a colourimeter (Ultra Scan Pro, HunterLab Co., Ltd, USA) and expressed as the L*, a* and b* values, respectively. Three replicates of each film were tested.
Light absorption and film transparency
The films were cut into a rectangle piece and directly placed into the test cell of a UV-752 spectrophotometer (JingHai instrument Co., Ltd, Shanghai, China) using an empty test cell as the reference. The measurement was performed in triplicate, and the average of three spectra was calculated. The light absorption at wavelengths ranging from 800 to 200 nm, and the transparency of the films was calculated using the following equation[Citation26]:
Transparency (A/mm) = – log T/X
where A = absorbance at each wavelength, T = transmittance (%) at each wavelength, and x = film thickness (mm). A higher transparency indicates more opaqueness.
Electrophoretic protein patterns
The molecular weight of the gelatin was determined using sodium dodecyl sulfate polyacrylamide gel electrophoresis (SDS-PAGE) as described by Weng et al.[Citation27] with a slight modification. The film sample (50 mg) was immersed in 25 mL of protein denaturing solutions. A 4% stacking gel and 6% separating gel were used. The gel was scanned using a G:BOX EF gel imaging system (Syngene, Cambridge, UK), and the intensities of the bands were analyzed using the Gene Tools software (Syngene).
Water solubility
The water solubility of the gelatin films was determined according to the method of Gontard et al.[Citation28] Film portions of 4 cm2 (2 × 2 cm2) were weighed (±0.0001 g) and subsequently dried in the electric heat drum wind drying oven at 105°C for 24 h. Then, films were recovered and re-weighed (±0.0001 g) to determine the initial dry weight (Wi). The samples were immersed in 30 mL of distilled water with gentle shaking for 24 h at room temperature (25–28°C). The samples were then passed through Whatman no. 1 filter paper. Then, the filter paper together with the undissolved film was dried at 105°C for 24 h and weighed (Wf). All tests were carried out in triplicate. The water solubility (WS%) of the gelatin films was calculated using the following equation:
where Wi = the initial dry film weight and Wf = the final dry film weight.
Mechanical properties
The tensile strength (TS), percentage elongation at break (EAB) and Young’s modulus (YM) were determined using an electronic tensile testing machine (XLW-PC, Labthink Co., Ltd, Shandong, China) at room temperature (25–28°C). The films were cut into rectangles (50 × 15 mm) and fixed on the instrument. The initial gauge and the stretching speed were respectively 15 mm and 50 mm/min. The maximum load and spacing were recorded during the stretching process. TS and EAB were evaluated in six samples from each type of film. TS was calculated according to the follows:
where Fmax is the maximum load (N) needed to pull the sample apart, Φ is the cross-sectional area (m2) of the samples. EAB was calculated by the following equation:
where ΔL is film extension at the point of sample rupture, L0 is the initial length of the film.
FT-IR spectroscopy
FT-IR spectra were obtained from discs containing 1 mg of the films (previously conditioned for 2 days in desiccators containing silica gel) in approximately 1 g of potassium bromide. All of the spectra were obtained using a Spectrum 100 infrared spectrometer (Perkin Elmer, Boston, MA, USA) in the range of 4000–450 cm−1 at a data acquisition rate of 4 cm−1. Curve fitting was subsequently performed using Peak Fit v4.0. All experiments were performed at least in triplicate and are represented as average spectra.
Scanning electron microscopy
Films were observed using scanning electron microscopy (JSM-6610, Shimadzu Co., Ltd, Japan). They were cut into small strips and mounted on copper stubs perpendicular to their surface. Samples were gold-coated and observed using an accelerating voltage of 10 kV.
Statistical analyses
Statistical analysis was performed using SPSS 13.0 for Windows (SPSS Inc., Chicago, IL, USA). Duncan’s multiple range tests were used to compare the difference among the mean values at the level of 0.05. The data was expressed as mean±SD (standard deviation).
Results and discussion
Film thickness
The thickness of the RG and PG films ranged from 65 to 93 and 73 to 103 μm, respectively. Gómez et al.[Citation29] found that the thickness of the bovine gelatin films prepared on a 120 × 120 mm2 plate with 40 mL gelatin solutions at a concentration of 4 g/100 mL were approximately 100 μm, and the thickness was the same as the PG films in the experiment but slightly thicker than the RG films. As the shows, the thickness of the RG films was lower than the PG films. The difference in thickness might be due to the different gelatin sources, resulting in different amino acid composition and the size of the triple helical structure etc.[Citation21]
Table 1. Thickness and water vapour permeabilities of the rabbit skin gelatin films and porcine skin gelatin films.
With increased glycerol concentrations, the thickness of the RG and PG films increased significantly (p < 0.05). More glycerol molecules could penetrate the gelatin network quickly to interact with the gelatin molecules that contributed to forming a thicker gelatin film.[Citation30] With gelatin additions, the thickness of the RG and PG films also increased. This might be due to the gelatin molecules forming a unique gelatin network structure via hydrogen bonds, and the volume of the network structure increased with increasing gelatin additions.[Citation31] However, the increase of RG films is not significant (p > 0.05), which implied that the increase of PG content had a greater effect on the thickness of gelatin film than it for RG.
Water vapour permeability (WVP)
The WVPs of the RG and PG films are shown in , and the values increased with increasing gelatin additions and glycerol concentrations. The WVPs of the RG films were lower than in the PG films, which might be due to the differences in amino acid composition.[Citation22] According to the results of Yu et al.[Citation24], the content of very hydrophobic amino acids (cystine, valine, methionine, isoleucine, leucine, phenylalanine, tryptophan) in rabbit skin gelatin was 137 g/100 g, which was higher than that of porcine skin gelatin with 105 g/100 g, thus increasing the hydrophobicity of the RG films and resulting in lower WVP values. In addition, amino acids (proline and hydroxyproline) could stabilise the ordered triple helical conformation when the gelatin formed the gel network, which was beneficial to the diffusivity of water molecules.[Citation32] The amino acid content of porcine skin gelatin was higher than that of the rabbit skin gelatin.[Citation24] Thus, the porcine gelatin network became more ordered and stabilised, which promoted the diffusivity of water vapour through the gelatin films and resulted in higher WVP values.
Colour
Colour parameters: L*-value, from black (0) to white (100); a*-value, from green (-) to red (+); b*-value, from blue (-) to yellow (+); white standard plate (L*-value = 98.92, a*-value = 0.19, b*-value = 1.02).
The colour of gelatin films can reflect the appearance of food and acceptability by the consumers. As shows the a*-value of all films were very close with white standard plate and had no significant difference (p > 0.05). The L*-values of the resulting films ranged from 96.13 to 96.77, which is close to the white standard plate (L* = 98.92), indicating that the films were almost transparent. The tendency of L*-values from RG and PG films were opposite with increasing gelatin additions and glycerol concentrations. We speculated that more intense interaction between gelatin and glycerol could be in RG films, which might decrease its L*-values slightly.
Table 2. Colour parameters of the rabbit skin gelatin films and porcine skin gelatin films.
The b*-values of all films were slightly lower than the white standard plate (b* = 1.02), which indicated the films were slightly yellowish. The stronger reaction between the glycerol molecules and the reactive group of lysine in the gelatin during the high-temperature (60°C) heating process may also result in the yellowness.[Citation33] The b*-values of the RG films was higher than the PG films, which also indicated that more intense interaction between gelatin and glycerol existed in RG films. In general, shows that the two gelatin films are almost transparent; thus, they will not affect the appearance of food if they are used as packaging films.
The b*-values of the RG and PG films decreased with increasing glycerol concentration from 22 to 30%, which could be due to the effect of the dilution of the proteins because glycerol is an uncoloured compound.[Citation34] Furthermore, the b*-values reached the highest upon increasing the gelatin additions and glycerol concentrations to 5 g/100 mL and 30%, which might be caused by the stronger reaction between the glycerol molecules and lysine.
Light transmission and transparency
The light transmission in the UV and visible region at selected wavelengths from 200 to 800 nm and the transparency at 600 nm of the RG and PG films are shown in . The light transmission of UV light at 280 nm was in the range of 11.99–19.39%. A very low transmission (0.23–0.36%) was found at 200 nm, which means that the two kinds of gelatin films have excellent barrier properties against UV light and could prevent a lipid oxidation reaction in the food system induced by ultraviolet light.[Citation3] Prior research[Citation35] showed that protein components with a high molecular weight (α-chain and β-components) were more likely to absorb ultraviolet light than those with a low molecular weight, and with a higher content of aromatic amino acids, the ultraviolet light absorption ability is stronger. Thus, the transmission in the UV range of RG was higher than for the PG films, which might be due to the molecular weight distribution and amino acid composition. However, according to the electrophoretic protein pattern of the RG and PG films (), the composition of the two gelatin subunits and their band strengths were similar and showed no obvious degradation phenomenon, which indicated that there were no significant difference in molecular weight distribution between the RG and PG films. Furthermore, the aromatic amino acid content of rabbit skin gelatin was higher than in porcine skin gelatin[Citation24]; thus, the difference in transmission in the UV range may be caused by differences in the amino acid composition. Light transmission in the visible range (350–800 nm) of the resulting films was from 80.98 to 92.47%, and the transmission increased with increasing wavelength.
Table 3. Light transmission (%) and transparency of the rabbit skin gelatin films and porcine skin gelatin films.
Figure 2. Protein patterns of the RG films and PG films. RG1, RG2, RG3: RG films 4 g + 22%, 4 g + 30%, 5 g + 30%. PG1, PG2, PG3: PG films 4 g + 22%, 4 g + 30%, 5 g + 30%.
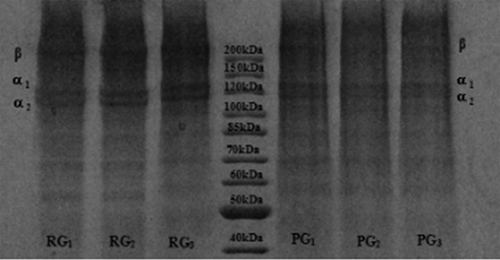
The higher transparency value indicated that the resulting films were less transparent. The transparency value of the RG and PG films at 600 nm was 0.35–0.73, which were lower than those reported by Pereda et al.[Citation36] and Saroat et al.[Citation37] for mammalian gelatin films (0.97 and 3.39, respectively), indicating that the resulting films were quite transparent. In comparison, the transparency of RG film is slightly lower than that of PG film, which was consistent with L*-values of them.
Water solubility
The water solubility of the RG and PG films are shown in . There are no significant difference (p > 0.05) between them, but the former had lower values than the latter. The difference may be related to the differences in amino acid composition.[Citation38] The rabbit skin gelatin contained more hydrophobic amino acids, which increased the hydrophobicity of the RG films. Gómez-Estaca et al.[Citation39] reported that water solubility of gelatin based films derived from bovine-hide and tuna skin were 34.3% and 39.9%, respectively. Hanani et al.[Citation19] also proved that fish skin gelatin film had higher water solubility than it from pork and bovine skin. Compared to fish skin gelatin film, RG film has no defects in water solubility, and is more suitable as a substitute for traditional mammalian gelatin film.
Table 4. Water solubility of the RG and PG films.
The water solubility of the RG and PG films increased upon increasing the glycerol concentration from 22 to 30%. The result could be due to the strong water solubility of glycerol. More glycerol might dissolve in water as its concentration increase to result in the water solubility of the gelatin films increased.
Upon increasing the gelatin content and glycerol concentration to 5 g/100 mL and 30%, the water solubility of the RG and PG films decreased, which might be due to the stronger interaction between the gelatin and glycerol molecules that resulted in an increase in the density of the gelatin films and a decrease in the mobility of molecules.[Citation40,Citation41] Thus, the water solubility decreased. In addition, the decreased tendency of the RG films was significantly higher than for the PG films under the same condition, which implied that the interaction between glycerol and rabbit skin gelatin molecules was stronger and led to a more dense structure of the rabbit skin gelatin network.
Mechanical properties
The tensile strength (TS), elongation at break (EAB) and Young’s modulus (YM) were applied for the characterisation of gelatin film intensity, extensibility and rigidity, respectively. They are usually related to the intermolecular interaction. The mechanical properties of the RG and PG films are shown in . The TSs of RG films were 35, 6.6 and 35 MPa at its composition were 4 g/100 mL of gelatin with 22% glycerol, 4 g/100 mL of gelatin with 30% glycerol, and 5 g/100 mL of gelatin with 30% glycerol respectively. The corresponding values of PG films were 32.8, 9.2 and 54 respectively and their EBA were 6.9%, 156% and 9%. The corresponding EBA values of RG films were 7.2%, 188% and 10% respectively.
Table 5. TS, EAB and YM of the RG films and PG films.
In general, the TSs of the RG films were lower than that for the PG films, whereas the values of EAB were higher than for the PG films. This result indicated that the rigidity of the RG films was slightly lower than that of the PG films, but the flexibility was more prominent. For an increased glycerol concentration from 22 to 30%, the increased trend of EAB in the RG films was more significantly than in the PG films. That could be due to more interaction between gelatin molecules and glycerol molecules in RG films. The glycerol molecules and gelatin molecules could form hydrogen bonds to cause that glycerol was immobilised on the gelatin network skeleton of film. Thus, the network possessed a stronger binding ability of glycerol and water molecules and led to a better flexibility. However, for increased gelatin additions from 4 to 5 g/100 mL, the increased trend of TS in the PG films was more significantly than in the RG films. This result may due to more gelatin chains interacting with each other to form a stronger gelatin network easily via hydrogen bonds in PG films, which resulted in the PG films being harder and more brittle.
As shown in , for increased glycerol concentrations from 22 to 30%, the TS and YM of the RG and PG films decreased significantly (p < 0.05), whereas the EAB increased significantly (p < 0.05). This may due to the glycerol acting as a plasticiser to increase the moisture content of gelatin films and improve the mobility of molecules, simultaneously contributing to reducing the intermolecular interaction of adjacent gelatin chains. Thus, the flexibility of the films increased, that is, the EAB increased.[Citation42] Upon increasing the gelatin additions and glycerol concentrations to 5 g/100 mL and 30% simultaneously, the TS and YM of the RG and PG films increased significantly (p < 0.05), whereas the EAB decreased (p < 0.05).
Some research has also proven that films derived from pork gelatin exhibited the greater mechanical properties than those formed using fish gelatin sources[Citation19,Citation20] The above results proved that the RG film has good mechanical properties and is a better source of gelatin to replace mammalian sources to prepare gelatin films compared with aquatic resources
FT-IR spectroscopy
FT-IR spectra of the RG and PG films are illustrated in and , respectively, and the Amide band position of the RG and PG films are shown in .The amide-A band was due to the stretching vibration of the N-H group. When the N-H groups of the peptide chain participated in the formation of hydrogen bonds, the position shifted to a lower wavenumber.[Citation43] When the RG and PG films had increased glycerol concentrations from 22 to 30%, the wavenumber of the peak in the amide-A region increased. This might be due to the gelatin molecules that did not react with the increased amount of glycerol molecules in the gelatin network structure, thus weakening the interaction and resulting in decreasing amounts of hydrogen bonds. However, upon simultaneously increasing the gelatin additions and glycerol concentrations to 5 g/100 mL and 30%, the amide-A bands shifted toward lower wavenumbers. This result may be associated with the fact that hydrogen bonds were formed by the interaction between the N-H group on the peptide chains and the -OH group of the glycerol molecules. Furthermore, the RG films showed a more significant decreasing trend and stronger vibration peak for the N-H group than for the PG films, which indicated that the interaction between rabbit skin gelatin and glycerol was more complete, and cross-linking mainly via H-bonds between the gelatin and glycerol was increased. That might lead to a decrease in the L*-value and transparency of the RG film, but a better flexibility than PG films, which was consistent with previous results and analysis.
Table 6. Amide band position of the RG films and PG films.
Table 7. Secondary structure contents of the rabbit skin gelatin films and porcine skin gelatin films.
The amide-I band vibration mode is primarily a C=O stretching vibration. The amide-I bands of gelatin films shifted to lower wavenumbers, indicating that the hydrogen bond interactions between water molecules and gelatin were enhanced, that is, the cross-linking between gelatin molecules was stronger. The RG films displayed the amide-I bands at 1636.2, 1636.0, and 1649.8 cm−1 respectively. Upon increasing the glycerol concentration from 22 to 30% when the gelatin addition was 4 g/100 mL, the absorption peaks showed no significant displacement. However, upon increasing the gelatin addition and glycerol concentration to 5 g/100 mL and 30% simultaneously, the absorption peaks shifted to higher wavenumbers. This result indicated that water-mediated hydrogen and cross-linking between gelatin molecules decreased when the concentrations of gelatin and glycerol were both higher. Combined with the amide A data, the RG film contained more interactions between the gelatin peptide chains and the –OH groups of the glycerol molecules; thus, the network structure of the gelatin film was more stable and compact. The PG films displayed the amide-I bands at wavenumbers of 1657.3, 1650.5, and 1651.8 cm−1 respectively. Upon increasing the glycerol concentration to 30% when the gelatin addition was 4 g/100 mL, the adoption peaks shifted to lower wavenumbers; then, the adoption peaks showed no significant displacement upon increasing the gelatin concentrations. This result indicated that the main interaction in the PG gelatin films was hydrogen bonding between the gelatin molecules. Therefore, the network structure of the PG films became denser than the network structure of the RG films, thus, the rigidity of the PG films was stronger. This conclusion was consistent with the results of the tensile strength as one of the mechanical properties.
The amide-I absorption (1700 to 1600 cm−1) is sensitive to the secondary structure of proteins; thus, this region was peak deconvoluted using Peak Fit software, and eight bands were obtained in the range of 1610–1692 cm−1. According to literature reports[Citation44,Citation45], these bands are attributed to the following assignments. RG films: the bands at 1610, 1624 and 1637 cm−1 are characteristic of the β-sheet, the band at approximately 1642 cm−1 corresponds to the random coil, the band at approximately 1658 cm−1 is attributed to the α-helix, and the bands at 1676, 1682 and 1692 cm−1 are due to β-turns (). PG films: the bands at 1610, 1623 and 1636 cm−1 are characteristic of the β-sheet, the band at approximately 1642 cm−1 corresponds to the random coil, the band at approximately 1654 cm−1 is attributed to the α-helix, and the bands at 1663, 1675 and 1692 cm−1 are due to β-turns ().
Figure 5. Fourier second-derivative and curve-fitting of the amide-I region (1700–1600 cm−1) for the RG (Lower case letters) and PG (Uppercase letter) gelatin films.
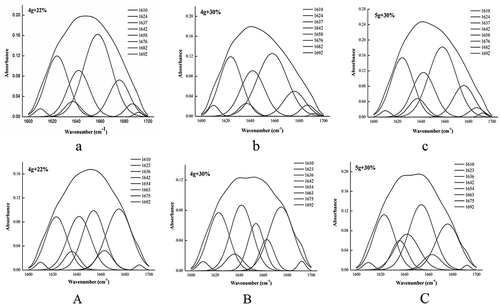
The contents of the secondary structure were calculated according to the peak areas shown in . Upon increasing the glycerol concentrations of the RG films, the β-sheet and random coil contents increased, whereas the α-helix content decreased. This result demonstrated that the interaction between gelatin molecules weakened, and the spatial structure of the protein became loose; thus, the stability of the gelatin films decreased. Upon increasing the gelatin additions, the content of the β-turns increased, the random coil decreased, and the α-helix and β-sheet had no significant changes. This result illustrated that the structure became slightly ordered; however, the main forces included the interaction between the gelatin and glycerol molecules, which meant that change in the orderly transition in the structure was not significant. Upon increasing the glycerol concentrations of the PG films, the structure tended to be disordered. However, upon increasing the gelatin additions, the structure tended to be ordered, which indicated that the interaction between the gelatin molecules dominated in the PG films.
Scanning electron microscopy
The surfaces and cross-sections of the microstructure of the RG and PG films are shown in and , respectively. As shown in , the RG and PG films exhibited smooth and homogeneous surfaces without a grainy structure, which indicated that an ordered matrix was formed and the resulting films had an excellent structural integrity. The surfaces of the RG films were more compact compared with the PG films, which might be due to the abundant interactions between rabbit skin gelatins and glycerol molecules, resulting in a denser structural network. Furthermore, the surface microstructure of the 4 g/100 mL gelatin with 30% glycerol of both the RG and PG films were looser than for other films. This result might be due to the quantities of plasticiser, which produced obvious differences in the morphology of the internal structure.[Citation46] The glycerol decreased the forces between the polymer chains, the free volume increased, and the structure was looser, thus resulting in an increase in the molecular mobility of the films. The cross-sections of the RG and PG films are shown in . The internal structures of the resulting films were regular, discontinuous and homogeneous.
Conclusion
As a potential alternative to traditional mammalian gelatin films, the characteristics and structure of RG films were researched in this study. Compared with PG films, RG films showed similar thicknesses, transparency, UV light barrier properties and better moisture barrier properties. In terms of mechanical properties, the rigidity of the RG films was slightly lower than that of the PG films, but the flexibility was more prominent. The FT-IR spectra confirmed that there were more interactions between the gelatin peptide chains and –OH groups of glycerol molecules in the RG films; thus, the network structure of the RG film was more stable and dense, given its better flexibility. Meanwhile, the main interaction in the PG gelatin films was via hydrogen bonding between gelatin molecules; therefore, the network structure of the PG films became less dense than the network structure of the RG films. This weakened the binding between the glycerol and water molecules, and thus the rigidity of the PG films was stronger. The SEM results showed that the surfaces of the RG and PG films were smooth and homogeneous. Their internal structure was regular and uniform, which indicated that an ordered matrix was formed in the inner layers. Due to religious reasons and the occurrence of BSE, gelatin obtained from skin, bone and scale of aquatic food processing by-products has gained great interest. However, gelatin films prepared from aquatic raw materials showed lower mechanical and higher water solubility than traditional mammalian gelatin films. In light of the results of this study, RG films did not have defects similar to aquatic gelatin films and can be used as a substitution for traditional gelatin films.
Additional information
Funding
References
- González, A.; Igarzabal, C. I. A. Soy protein–Poly (Lactic Acid) Bilayer Films as Biodegradable Material for Active Food Packaging. Food Hydrocolloids 2013, 33, 289–296. DOI: 10.1016/j.foodhyd.2013.03.010.
- Bucci, D. Z.; Tavares, L. B. B.; Sell, I. PHB Packaging for the Storage of Food Products. Polymer Testing 2005, 24, 564–571. DOI: 10.1016/j.polymertesting.2005.02.008.
- Limpisophon, K.; Tanaka, M.; Weng, W.; Abe, S.; Osako, K. Characterization of Gelatin Films Prepared from Under-Utilized Blue Shark (Prionace Glauca) Skin. Food Hydrocolloids 2009, 23, 1993–2000. DOI: 10.1016/j.foodhyd.2009.03.014.
- Gontard, N.; Guilbert, S. Bio-Packaging: Technology and Properties of Edible And/Or Biodegradable Material of Agricultural Origin, in Food Packaging and Preservation; Springer: Boston MA, 1994; 159–181.
- Cuq, B.; Aymard, C.; Cuq, J. L.; Guilbert, S. Edible Packaging Films Based on Fish Myofibrillar Proteins: Formulation and Functional Properties. Journal of Food Science 1995, 60, 1369–1374. DOI: 10.1111/j.1365-2621.1995.tb04593.x.
- Ktari, N.; Jridi, M.; Nasri, R.; Lassoued, I.; Ayed, H. B.; Barkia, A.; Nasri, M. Characteristics and Functional Properties of Gelatin from Zebra Blenny (Salaria Basilisca) Skin. LWT Food Science and Technology 2014, 58, 602–608. DOI: 10.1016/j.lwt.2014.03.036.
- Liu, F.; Antoniou, J.; Li, Y.; Ma, J.; Zhong, F. Effect of Sodium Acetate and Drying Temperature on Physicochemical and Thermomechanical Properties of Gelatin Films. Food Hydrocolloids 2015, 45, 140–149. DOI: 10.1016/j.foodhyd.2014.10.009.
- Jongjareonrak, A.; Benjakul, S.; Visessanguan, W.; Prodpran, T.; Tanaka, M. Characterization of Edible Films from Skin Gelatin of Brownstripe Red Snapper and Bigeye Snapper. Food Hydrocolloids 2006, 20, 492–501. DOI: 10.1016/j.foodhyd.2005.04.007.
- Hosseini, S. F.; Rezaei, M.; Zandi, M.; Ghavi, F. F. Preparation and Functional Properties of Fish Gelatin–Chitosan Blend Edible Films. Food Chemistry 2013, 136, 1490–1495. DOI: 10.1016/j.foodchem.2012.09.081.
- Ahmad, M.; Benjakul, S.; Sumpavapol, P.; Nirmal, N. P. Quality Changes of Sea Bass Slices Wrapped with Gelatin Film Incorporated with Lemongrass Essential Oil. International Journal of Food Microbiology 2012, 155, 171–178. DOI: 10.1016/j.ijfoodmicro.2012.01.027.
- Jang, S.; Shin, Y. J.; Song, K. B. Effect of Rapeseed Protein–Gelatin Film Containing Grapefruit Seed Extract on ‘Maehyang’ Strawberry Quality. International Journal of Food Science and Technology 2011, 46, 620–625. DOI: 10.1111/j.1365-2621.2010.02530.x.
- Antoniewski, M. N.; Barringer, S. A.; Knipe, C. L.; Zerby, H. N. Effect of a Gelatin Coating on the Shelf Life of Fresh Meat. Journal of Food Science 2007, 72, 382–387. DOI: 10.1111/j.1750-3841.2007.00430.x.
- Nilsuwan, K.; Benjakul, S.; Prodpran, T. Properties and Antioxidative Activity of Fish Gelatin-Based Film Incorporated with Epigallocatechin Gallate. Food Hydrocolloids 2018, 80, 212–221. DOI: 10.1016/j.foodhyd.2018.01.033.
- Benjakul, S.; Tongnuanchan, P.; Prodpran, T. Use of Fish Gelatin Based-Films as Edible Pouch to Extend the Shelf-Life of Dried Chicken Powder and Chicken Oil. World academy of science, engineering and technology. International Journal of Industrial Manufacturing Engineering 2017, 11.
- Hanani, Z. N.; McNamara, J.; Roos, Y. H.; Kerry, J. P. Effect of Plasticizer Content on the Functional Properties of Extruded Gelatin-Based Composite Films. Food Hydrocolloids 2013, 31, 264–269. DOI: 10.1016/j.foodhyd.2012.10.009.
- Silva, R. S.; Bandeira, S. F.; Pinto, L. A. Characteristics and Chemical Composition of Skins Gelatin from Cobia (Rachycentron Canadum), LWT. Food Science and Technology 2014, 57, 580–585.
- Sow, L. C.; Yang, H. Effects of Salt and Sugar Addition on the Physicochemical Properties and Nanostructure of Fish Gelatin. Food Hydrocolloids 2015, 45, 72–82. DOI: 10.1016/j.foodhyd.2014.10.021.
- Avena‐Bustillos, R. J.; Chiou, B. S.; Olsen, C. W.; Bechtel, P. J.; Olson, D. A.; McHugh, T. H. Gelation, Oxygen Permeability, and Mechanical Properties of Mammalian and Fish Gelatin Films. Journal of Food Science 2011, 76, 519–524. DOI: 10.1111/j.1750-3841.2011.02312.x.
- Hanani, Z. N.; Roos, Y. H.; Kerry, J. P. Use of Beef, Pork and Fish Gelatin Sources in the Manufacture of Films and Assessment of Their Composition and Mechanical Properties. Food Hydrocolloids 2012, 29, 144–151. DOI: 10.1016/j.foodhyd.2012.01.015.
- Shakila, R. J.; Jeevithan, E.; Varatharajakumar, A.; Jeyasekaran, G.; Sukumar, D. Comparison of the Properties of Multi-Composite Fish Gelatin Films with that of Mammalian Gelatin Films. Food Chemistry 2012, 135, 2260–2267. DOI: 10.1016/j.foodchem.2012.07.069.
- Kołodziejska, I.; Skierka, E.; Sadowska, M.; Kołodziejski, W.; Niecikowska, C. Effect of Extracting Time and Temperature on Yield of Gelatin from Different Fish Offal. Food Chemistry 2008, 107, 700–706. DOI: 10.1016/j.foodchem.2007.08.071.
- Chuaynukul, K.; Prodpran, T.; Benjakul, S. Properties of Thermo-Compression Molded Bovine and Fish Gelatin Films as Influenced by Resin Preparation Condition. The International Food Research Journal 2015, 22, 1095–1102.
- Haug, I. J.; Draget, K. I.; Smidsrød, O. Physical and Rheological Properties of Fish Gelatin Compared to Mammalian Gelatin. Food Hydrocolloids 2004, 18, 203–213. DOI: 10.1016/S0268-005X(03)00065-1.
- Yu, W.; Wang, X.; Ma, L.; Li, H.; He, Z.; Zhang, Y. Preparation, Characterisation and Structure of Rabbit (Hyla Rabbit) Skin Gelatine. International Journal of Food Science and Technology 2016, 51, 574–580. DOI: 10.1111/ijfs.13012.
- Ma, M.; Ma, L.; Yu, W.; Zhang, X.; Shen, Y.; Zhang, Y. Research on Rapid Gelatinization of Rabbit Skin Collagen as Effect of Acid Treatment. Food Hydrocolloids 2018, 77, 945–951. DOI: 10.1016/j.foodhyd.2017.11.042.
- Han, J. H.; Floros, J. D. Casting Antimicrobial Packaging Films and Measuring Their Physical Properties and Antimicrobial Activity. Journal of Plastic Film & Sheeting 1997, 13, 287–298. DOI: 10.1177/875608799701300405.
- Weng, W.; Zheng, H. Effect of Transglutaminase on Properties of Tilapia Scale Gelatin Films Incorporated with Soy Protein Isolate. Food Chemistry 2015, 169, 255–260. DOI: 10.1016/j.foodchem.2014.08.012.
- Gontard, N.; Guilbert, S. CUQ, J.L., Edible Wheat Gluten Films: Influence of the Main Process Variables on Film Properties Using Response Surface Methodology. Journal of Food Science 1992, 57, 190–195. DOI: 10.1111/j.1365-2621.1992.tb05453.x.
- Gómez-Estaca, J.; Gómez-Guillén, M. C.; Fernández-Martín, F.; Montero, P. Effects of Gelatin Origin, Bovine-Hide and Tuna-Skin, on the Properties of Compound Gelatin–Chitosan Films. Food Hydrocolloids 2011, 25, 1461–1469. DOI: 10.1016/j.foodhyd.2011.01.007.
- Abdorreza, M. N.; Cheng, L. H.; Karim, A. A. Effects of Plasticizers on Thermal Properties and Heat Sealability of Sago Starch Films. Food Hydrocolloids 2011, 25, 56–60. DOI: 10.1016/j.foodhyd.2010.05.005.
- Wu, J.; Liu, H.; Ge, S.; Wang, S.; Qin, Z.; Chen, L.; Zhang, Q.; Liu, Q.; Zhang, Q. The Preparation, Characterization, Antimicrobial Stability and in Vitro Release Evaluation of Fish Gelatin Films Incorporated with Cinnamon Essential Oil Nanoliposomes. Food Hydrocolloids 2015, 43, 427–435. DOI: 10.1016/j.foodhyd.2014.06.017.
- Avena‐Bustillos, R. J.; Olsen, C. W.; Olson, D. A.; Chiou, B.; Yee, E.; Bechtel, P. J.; McHugh, T. H. Water Vapor Permeability of Mammalian and Fish Gelatin Films. Journal of Food Science 2006, 71, E202–E207. DOI: 10.1111/jfds.2006.71.issue-4.
- Hoque, M. S.; Benjakul, S.; Prodpran, T. Effect of Heat Treatment of Film-Forming Solution on the Properties of Film from Cuttlefish (Sepia Pharaonis) Skin Gelatin. Journal of Food Engineering 2010, 96, 66–73. DOI: 10.1016/j.jfoodeng.2009.06.046.
- Paschoalick, T. M.; Garcia, F. T.; Sobral, P. D. A.; Habitante, A. M. Q. B. Characterization of Some Functional Properties of Edible Films Based on Muscle Proteins of Nile Tilapia. Food Hydrocolloids 2003, 17, 419–427. DOI: 10.1016/S0268-005X(03)00031-6.
- Mark, H. F.; Bikales, N.; Overberger, C. G.; Menges, G.; Kroschwitz, J. I. Encyclopedia of Polymer Science and Engineering. In Aiche Journal; Kroschwitz, J. I., Eds.; Wiley: New York, 1987; pp 1051–1052.
- Pereda, M.; Ponce, A. G.; Marcovich, N. E.; Ruseckaite, R. A.; Martucci, J. F. Chitosan-Gelatin Composites and Bi-Layer Films with Potential Antimicrobial Activity. Food Hydrocolloids 2011, 25, 1372–1381. DOI: 10.1016/j.foodhyd.2011.01.001.
- Rawdkuen, S.; Sai-Ut, S.; Benjakul, S. Properties of Gelatin Films from Giant Catfish Skin and Bovine Bone: A Comparative Study. European Food Research and Technology 2010, 231, 907–916. DOI: 10.1007/s00217-010-1340-5.
- Tu, Z.; Huang, T.; Wang, H. Comparison on Filming Properties of Three Fish Water Fish Scale Gelatins. Food and Fermentation Industries 2014, 40, 151–154.
- Gómez-Estaca, J.; Montero, P.; Fernández-Martín, F.; Gómez-Guillén, M. C. Physico-Chemical and Film-Forming Properties of Bovine-Hide and Tuna-Skin Gelatin: A Comparative Study. Journal of Food Engineering 2009, 90, 480–486. DOI: 10.1016/j.jfoodeng.2008.07.022.
- Kavoosi, G.; Rahmatollahi, A.; Dadfar, S. M. M.; Purfard, A. M. Effects of Essential Oil on the Water Binding Capacity, Physico-Mechanical Properties, Antioxidant and Antibacterial Activity of Gelatin Films. LWT Food Science and Technology 2014, 57, 556–561. DOI: 10.1016/j.lwt.2014.02.008.
- Bigi, A.; Cojazzi, G.; Panzavolta, S.; Rubini, K.; Roveri, N. Mechanical and Thermal Properties of Gelatin Films at Different Degrees of Glutaraldehyde Crosslinking. Biomaterials 2001, 22, 763–768. DOI: 10.1016/S0142-9612(00)00236-2.
- Bergo, P.; Sobral, P. J. A. Effects of Plasticizer on Physical Properties of Pigskin Gelatin Films. Food Hydrocolloids 2007, 21, 1285–1289. DOI: 10.1016/j.foodhyd.2006.09.014.
- Jridi, M.; Souissi, N.; Mbarek, A.; Chadeyron, G.; Kammoun, M.; Nasri, M. Comparative Study of Physico-Mechanical and Antioxidant Properties of Edible Gelatin Films from the Skin of Cuttlefish. International Journal of Biological Macromolecules 2013, 61, 17–25. DOI: 10.1016/j.ijbiomac.2013.06.040.
- Prystupa, D. A.; Donald, A. M. Infrared Study of Gelatin Conformations in the Gel and Sol States. Polymer Gels and Networks 1996, 4, 87–110. DOI: 10.1016/0966-7822(96)00003-2.
- Surewicz, W. K.; Mantsch, H. H.; Chapman, D. Determination of Protein Secondary Structure by Fourier Transform Infrared Spectroscopy: A Critical Assessment. Biochemistry 1993, 32, 389–394. DOI: 10.1021/bi00053a001.
- Andreuccetti, C.; Carvalho, R. A.; Grosso, C. R. Effect of Hydrophobic Plasticizers on Functional Properties of Gelatin-Based Films. Food Research International 2009, 42, 1113–1121. DOI: 10.1016/j.foodres.2009.05.010.