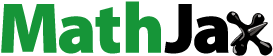
ABSTRACT
Potato peel, as the outermost tissue of the fruit, playing a role of protection and fresh keeping on the pulp tissue, is crucial for the design of potato harvesting machines. In this study, two types of potato ‘Yangyu 4’ and ‘Helan 14’ were selected to conduct friction, tensile and tear tests on their peel. Longitudinal and transverse tensile tests were conducted on the peels of two potato cultivars using an electronic universal testing machine, and tear tests were carried out on peels as well. The static friction coefficient for different materials, stress-strain curves and tear force-deformation curves of the peels were obtained and the tensile strength, elastic modulus, failure strain tear strength of the peels were measured. The results showed that the maximum value of the static friction coefficient against metallic materials was and
against rubber materials, respectively. The maximum values of the tensile strength, elastic modulus, fracture strain, tear strength were 1.648 MPa, 0.388 MPa, 4.5%, 0.326 kN·m-1, respectively. Scanning electron microscopy images show that many compact and regular polygonal similar-grain grid structures distributed onty the epidermis surface and microcrack basically did not exist on the peel. The spherical micro-convex structures arranged closely and linearly at the boundary of the grid structure. Hierarchical structure surface with spherical micro-convex structures was one of the reasons for its outstanding friction coefficient. The bearing capacity of the peel depended on the number and distribution of grid structures on the surface, and the size and shape of the epidermal cells.
Introduction
Facts show that vast majority of mechanical damages to potatoes come from the process of harvesting.[Citation1–Citation3] Mechanical damage and collision abrasion seriously affect potato yield and cause double decline of potatoes’ appearance and quality so as to implicate the development of the entire potato industry.[Citation4–Citation6] However, In the entire process of harvesting, packaging, handling, transportation, storage and sale, there are static load, squeeze, vibration, collision, impact as well as other forms of load, formed the immediate damage of potato based on the form of plastic or brittle failure[Citation7–Citation10], and it is inevitable that the first to be damaged is potato peel. Facts have proved that when the potato peel was broken, the potato pulp tissue would be gradually darken and then damaged to completely not edible as food. Potato peel, as the outermost tissue of the fruit, playing a protective and fresh keeping role on the potato pulp tissue, has strong resistance to microbial infection and mechanical damage. Moreover, the potato peel is also crucial for the designing of potato harvesting machines as the mechanical properties of peel are an important reference for harvesting, processing, packaging, storing, and transporting the fruit. Studies [Citation11–Citation13] have shown that mechanical properties of fruits and vegetables peel, the outermost protective tissue of fruitage, are important part of the physical properties of fruit.
In the past, as the result of difficulties in the preparation of peel samples and load applying, there were few studies on the mechanical properties of fruit and vegetables peel. However, the research of pericarp has drawn more and more attention in recent years.[Citation13] In addition, studies on fruits and vegetables peel focused on the fruits whose peel and pulp are easy to separate, such as lychee, grape, orange and so on. [Citation14,Citation15] For example, Juxia et al.[Citation11–Citation13] conducted a research where the mechanical properties of varieties of apple peel were tested, and the microstructure of apple peel was simulated and analyzed. Wang et al.[Citation15] investigated grape and tomato peels in tensile test and concluded that grape skin and tomato skin are isotropic materials. The strength of the grape skin and the tomato peel could reduce mechanical damage. Andrews et al.[Citation16] stretched tomato peel samples and obtained the tensile strength and elastic modulus of different types. Hetzroni et al.[Citation17] examined the mechanical properties of ripe tomato peels under uniaxial tension and puncture tests. Allende et al.[Citation18] isolated different sections of tomato pericarp and evaluated the skin tissue with light microscopy to determine its geometrical properties. Grimm et al.[Citation19] found that strips of fruit skin from regions with skin spots had increased maximum force and modulus of elasticity by tensile tests on apple peel regions with and without skin spots. Singh and Reddy[Citation20] investigated the mechanical properties of orange peel under ambient and refrigerated storage conditions and carried out tensile tests and cutting tests.
However, for the fruits and vegetables whose peel and pulp are not easy to peel off, such as apples, pears, potatoes and so on, the study on the mechanical properties mainly focused on the compression, impact and other aspects of the test upon the whole fruitage.[Citation21–Citation24] At present, the research on potato mainly focused on mechanical damage of pulp tissue or the effect of storage time on the quality of the pulp. [Citation22,Citation25–Citation27] For instance, the study of turgor pressure and the compressive stiffness of apple and potato tissues was conducted to understand their fracture strength against external and internal pressures.[Citation28] Annalisa et al.[Citation5] studied tuber starch characteristics and chemical-thermal properties of 21 types potatoes, and determined their genetic diversity through SSR markers. Nadine Strehmel et al.[Citation6] analyzed the previously neglected nature and sequence of responses in primary metabolism and compared the cultivars ‘Afra’ and ‘Milva’ that differ in tissue elasticity and susceptibility to blackspot bruising. Mechanical stress application was highly standardized and differential formation of bruising damage and occurrence of small cracks in the outer tuber layers were demonstrated in paper.[Citation6] Li et al.[Citation29] studied that ClO2 could greatly against the pathogen of F. sulphureum, which could provide scientific theoretical basis for the safe and efficient application of ClO2 in the prevention and control of potato diseases after harvest.
At present, the study on the microstructure of fruit and vegetable peel mainly focuses on the microstructure and its storage mechanism, such as the function of cuticle on storage and fresh-keeping of fruits and vegetables or the relationship between epidermal microstructure and storability. Agata et al.[Citation30] found that the cuticle and epicuticular wax formed on the apple peel, plays a crucial role in preserving the fruit life by preventing water evaporation and the penetration of pathogen, as well as maintaining fruit firmness. Maguire et al.[Citation31] used experimental data and developed a model based on diffusion of gases to explain the major role of cuticular cracks in determining apple fruit water vapor permeance. Juxia et al.[Citation11] systematically studied the differences between mechanical properties and microstructure of three different varieties of apple peels as the storage time changed. They found that mechanical properties were closely related to peel microstructures and strengths and weaknesses mainly depended on the number and width of microcracks on the cuticle, cuticle form, cell aspect radio, and space between cells. There is a close relationship between the microstructure of biological tissues and their macroscopic mechanical properties. A large number of facts have shown that on the basis of fully understanding the biological microstructures and its functions, designing and constructing materials with similar structures and functions has become a reality in many fields.[Citation32–Citation35]
The microstructure of potato peel in relation to its mechanical properties has not yet been investigated. For the purpose of building a data platform for the application of bionic engineering technology in potato harvesting machine and providing necessary material data for the design, simulation, and optimization of bionic harvesting components. In this study, the friction testing, tensile and tearing testing for the potato peel were conducted. The mechanical properties such as friction coefficient, tensile strength, elastic modulus, tear strength, and microstructure of the peel of two kinds of potatoes were analyzed, which provided friction parameters for surface feature design, material selection and performance simulation analysis of bionic components on digging device, separating device and collecting device of potato harvesting machine. Through the tensile and tearing test of potato peel, the tensile fractures were obtained as well as the fracture mechanisms and tensile properties, which provided mechanical parameters for the process optimization and bionic configuration design of the components that tended to damage potato peel on the potato harvesting machine. The objectives of this study were (1) to provide reference for the selection method in relation to the harvesting, processing, packaging, storing and transporting of potatoes; the designing of related mechanical equipment; and depth development of potato products; (2) to provide knowledge for better cultivation of the potato fruit and evaluation of the potato peel; and (3) to do some fundamental work in support of the prototype selection of biomimetic materials for water resistance and fresh-keeping of fruit and vegetable skin packaging.
Materials and methods
Experimental materials
As shown in , potatoes of ‘Yangyu 4’ and ‘Helan 14’ without any damage and disease were obtained from the fields in Fulongquan town of Nongan county, the hometown of potato in Jilin Province in September 25, 2016.
As the materials of potato harvesting machine are mainly plain carbon steel and rubber, experiments were conducted using friction plates made from these two varieties of materials. The plain carbon steel plates with the thickness of 8mm and smooth surface without rust were made from Q235 steel, while the rubber plates were possessed with thickness of 8 mm and smooth surface without burr, scratch and concave-convex structure.
Test methods
The friction test was conducted on the mechanical static friction test platform developed by the College of Biology and Agricultural Engineering, Jilin University. Its structure is shown in (a) and working principle in , where B was the test bed length in mm and H was the height of the stage in mm. The friction coefficient was tested by the following steps: (1) the friction plate was embedded in the stage and then the sample was placed on the friction plate; (2) the screw was turned to push the slider forward so as to rise the stage slowly; (3) the screw-turning was stopped when the sample started to slide; (4) the static friction coefficient could be calculated through the rising height by Equation (2). Just as shown in , the peel samples of various parts were obtained in the sequence of longitudinal end, central part and then the side.
According to T 1040.3-2006/T 1040.3–2006 Plastics – Determination of Tensile Properties–Part 3: Test Conditions for Films and Sheets[Citation36], tensile properties of potato peel samples, obtained from the biggest area on potatoes in longitudinal and transverse directions, respectively ()), were measured by tensile sensor on the Meters MTS-C43.104 microcomputer control electronic universal testing machine, where the samples were gripped with upper and lower clamps. During sampling, the peel was removed from the fruitage and placed on a smooth rubber pad, and the pulp portion was gently scraped off the peel under a magnifying glass and then made into long strips with the size of 40 mm × 15 mm × t mm ()), where t was the thickness of the sample rind. The number of samples in the same part of the same direction was 10. The thickness of potato peel samples was measured with vernier caliper and the thickness range of ‘Helan 14’ was 0.22–0.38 mm while that of ‘Yangyu 4’ was 0.22–0.36 mm. To prevent water loss of the samples, the test was conducted immediately on the machine (). Juxia et al. [Citation13] and Hetzroni et al.[Citation17] used razor blades to obtain mechanically peeled tomato skin, which was then made into specimens for a tensile test. To avoid stress concentration, the apple, grape, tomato, and orange peels in Ref. [Citation8,Citation16,Citation27] were cut into rectangular samples for tensile test.
Tear test was conducted based on T 1040.3-2006/T 16578.1–2008 Plastics–Film and Sheeting–Determination of Tear Resistance–Part 1: Trouser Tear method[Citation37], and samples were obtained in the longitudinal direction on potatoes and made into long strips with the size of 30 mm × 15 mm × t mm, where t is the thickness of the peel sample. The thickness of the tested samples was 0.22–0.38 mm for ‘Helan 14’ potato while that was 0.22–0.36 mm for ‘Yangyu 4’ potato. The number of two varieties of potato peel samples and test equipment were the same as the tensile test, as shown in .
Experiment design
Friction performance test. The friction behaviors occurred mainly between the harvesting equipment and potato peel in the process of potato harvesting, so it was obligatory to repeat the static friction coefficient tests in various parts of the potato peel for 10 times with the average as the final value. The calculation formula of static friction coefficient (μ) is as follows:
where
F1 is the pulling force component,
F2 is the pressure component, G is gravity, f is the friction force, N is the supporting force, and θ is the angle between the base and object stage.
Tensile properties test. The tensile behaviors of potatoes occurred mainly when potatoes were separated from the soil, so it was measured 10 times and the average value was the final result. The loading speed of the tensile test was set at 1 mm/min. The original gauge distance of the sample was measured by the vernier caliper, which was 9.98–10.06mm of ‘Helan 14’ and 9.98–10.08 mm of ‘Yangyu 4’. The measurement accuracy was 0.02 mm.
For the potato peel being the outermost layer of the fruit, its mechanical properties, such as elastic modulus, tensile strength and other indicators, can be used to predict the extent of damage and destruction to the fruit in the process of harvesting, packaging, storage and transportation, etc. The modulus of elasticity was taken as an important indicator of the peel’s elastic deformation. From the peel stress–strain curve, the maximum slope value can be obtained. The calculation formula is expressed as[Citation17]
where represents the axial tensile specimen force,
represents the original cross-sectional area of the sample, and
,
represent the elongation of the specimen when it is stretched and the standard distance, respectively,
represents the width of the sample. The tensile strength
is an important evaluation index of peel damage or destruction. The corresponding stress values can be obtained from the breaking point of the peel force–displacement curve[Citation19]. The calculation formula is given by[Citation20]
whererepresents the amount of force at which the specimen starts to fracture.
Tear test. The fruit peel is the outermost layer of a thin film material and is under the effect of internal and external load in the process of the growth of the fruit. The pros and cons of internal fruit quality can not only be characterized by the tensile strength index of the peel but also peel tear strength can be used. In order to further compare the quality of the peel material, a tear test was carried out to investigate the peel in this study.
According to the document T 1040.3-2006/T 16578.1–2008 Plastics—Film and Sheeting –Determination of Tear Resistance—Part 1: Trouser tear method[Citation37],when the tear strength was measured by the single-slit method[Citation38], a notch parallel to the longitudinal direction was cut in the middle of the sample’s width direction and the two ‘trousers’ formed at the site of the incision were subjected to a tear test as shown in . The specimens with a size of 30 mm × 15 mm × t mm were prepared (t denotes the peel specimen’s thickness).
The sample was tested with a universal testing machine. A 15-mm incision was made in the direction parallel to the longitudinal axis of the sample, after which the formed two leg notches were subjected to a tensile test (as shown in ). According to the standard document T 1040.3-2006/T 3917.2–2009 Textile Fabric–Tearing Performance–Part 2: Breeches Sample (Single Slit) Determination of the Tear Strength [Citation38], if the tear curve has many peaks, it can be divided into four areas from the first peak to the last peak. In regions 2, 3, and 4, the two lowest peak and two highest peaks were selected, and the average value of 12 peak loads was the tear force of the sample. The calculation formula for the tear strength () is given by
where represents the force of the tear sample and
represents the original thickness of the sample. The sample was prepared with a magnifying glass to ensure that the edge was smooth and no gap. In order to prevent water loss of the sample, the potato peel samples were immediately put on the electronic universal testing machine controlled by microcomputer to test. The loading speed during the test was 1 mm/min and maintained throughout the test.
Results and analysis
Static friction test
From , it is clear that the material coefficients of the contact parts have great influence on the static friction coefficient of the potato peel. Under the same pressure, rubber materials can exert more static friction force on the potato peel so as to maintain a relatively static state between potato peel and rubber sheet. When potato peel and friction plate were in contact with each other, the static friction coefficient of ‘Yangyu 4’ potato peel was and
against metallic materials and rubber materials, respectively. While the static friction coefficient of ‘Helan 14’ potato peel was
and
against metallic materials and rubber materials, respectively.
In the process of friction performance test, it was observed that the friction coefficient of potato peel against rubber materials was larger than that against metallic materials, which can be used as an index to evaluate the friction performance of potato peel. Through the analysis of the static friction coefficient of the two kinds of potatoes, it was obvious that the static friction force between the metallic material and potato peel was small.
In order to reduce the contact time and friction between the excavation device and the potato peel during the harvest process or cleaning process, the coefficient of friction of the excavation device and the cleaning device of the potato harvester can be lowered with coating or by using the biomimetic technology. Thus, reducing the mechanical damage to the potato peel can ensure the excellent quality of the potato. In addition, the delivery capacity of potatoes was enhanced by increasing the force of friction on the delivery device during the harvest process when the delivery device in the potato harvesting machine can be covered with a rubber material.
Tensile test
and depict the stress–strain curve of transverse sampling and longitudinal sampling of ‘Yangyu 4’ and ‘Helan 14’. It can be seen that there is a nonlinear relationship without obvious biological yield point between the stress and strain of ‘Yangyu 4’ and ‘Helan 14’. The curves were S-shape and divided into two stages bounded by fracture strain, which was 3.5–4.5% for ‘Yangyu 4’ and 2.0–3.2% for ‘Helan 14’.
,) are composed of two sections. The first part is an approximately straight line segment (oa section and oc section, corresponding to oe section in ) and og section in )), where the strain increases faster than the stress. In the early stages of stretching, the unstretched pericarp was in a state of micro-buckling, which resulting in a larger tensile strain and uneven stress distribution. Moreover, due to the existence of interstitial cells in the pericarp, tissue cells reoriented in the early stage of stretching.[Citation39]
For ab section and cd section in ,), respectively, showing a arched curve, the peel force displays a more uniform stress distribution with an increase of stress and strain. At point b (or point d, corresponding to point f in ) and point h in )), the stress reached maximum value. After that, the peel sample fractured gradually, but the stress did not decrease to zero rapidly. It decreased gradually and the curve has a smaller extension.
In the tensile test process, the potato peel specimens broke at fruit spots and then gradually extended, that shown no simultaneity in fracture. This is consistent with the observed phenomena during the test by Yu[Citation40] and Juxia.[Citation11] Similar results have also been validated in apple peel experiments conducted by Wang Juxia.[Citation13] On the peel spots being stress concentration points, the fracture in the surface of the specimens will pass through the various fruit spots, as shown in ).
As depicted in ,), the same characteristics of the stress–strain curves were observed in ‘Helan 14’ potato samples. Two types of potato peels all showed significant brittle fracture. It was indicated by comparing the tensile test curves of two varieties of potato peel that the maximum tensile stress of the two kinds of potato peel sampled in longitudinal direction was basically coincident as well as the trend of the frontal section of the curve. Besides, the fracture velocities of the two varieties of potato peel were also different. ‘Helan 14’ was faster than ‘Yangyu 4’ and the maximum fracture stress of ‘Helan 14’ was lower than that of ‘Yangyu 4’. Therefore, the tensile fracture parameters of ‘Yangyu 4’ can be used as an index to evaluate the tensile fracture properties of potato peel.
By comparing the fracture of ‘Yangyu 4’ in ,), it was observed that the potato peel rapidly fractured at the stress of about 1.53 MPa (corresponding to 1.35 MPa for Helan 14) for longitudinal sampling and 1.37 MPa (corresponding to 1.27 MPa for Helan 14) for transverse sampling, which indicated that the peel of ‘Yangyu 4’ had character of serious brittleness and fractured immediately. For two parts of peel—i.e., Yangyu 4 longitudinal, Helan 14 longitudinal, Yangyu 4 transverse, and Helan 14 transverse—the results obtained in the maximum load test, tensile strength test, elastic modulus test, and failure strain test are shown in .
Table 1. Mean value and standard error of mechanical properties of potato peels under tensile loading.
By comparing the fracture of ‘Yangyu 4’ in and the fracture of ‘Helan 14’ in , it is clear that the maximum fracture stress of transverse sampling was significantly lower than that of longitudinal sampling which indicates that the tensile properties of ‘Helan 14’ in the longitudinal direction was stronger than that in the transverse direction.
shows the distribution of maximum tensile load. By comparing maximum tensile load of the longitude sampling and transverse sampling in both two kinds of potatoes, it can be obtained obviously that the maximum tensile load of peel were different for different varieties or sampling orientation. While the mean values of maximum tensile load of ‘Yangyu 4’ presented a greater value compared that of ‘Helan 14’ at both sampling orientation.
Distribution tensile strength. The fracture strength of peel can be used as an important evaluation index of fruit damage or destruction. As shown in , the range of mean values of tensile strength was 1.35–1.41 MPa for ‘Yangyu 4’ and 1.22–1.31 MPa for ‘Helan 14’. By comparing the mean values of tensile strength of the longitude sampling and transverse sampling in both two kinds of potatoes, it can be obtained obviously that the tensile strength of peel are different for different varieties or sampling orientation. While the mean values of tensile strength of ‘Yangyu 4’ presented a greater value compared ‘Helan 14’ at both sampling orientation. The tensile strength of longitudinal samples was greater than the transverse samples for both the two kinds of potatoes.
Distribution of elastic modulus. Elastic modulus can characterize the material’s resistance to deformation, which is an important indicator to measure the mechanical properties of the material. As shown in , the range of mean values of elastic modulus was 0.18–0.24 MPa for ‘Yangyu 4’ and 0.14–0.18 MPa for ‘Helan 14’. Distribution of fracture strain: By comparing the mean values of fracture strain of the longitude sampling and transverse sampling in both two kinds of potatoes, it can be obtained obviously that the fracture strain of peels are different for different varieties or sampling orientation. While the mean values of fracture strain of ‘Yangyu 4’ presented a greater value compared ‘Helan 14’ at both sampling orientation. The mean values of fracture strain of the longitude sampling presented greater value than that of transverse sampling. shows that the longitudinal specimens were more extensible than transverse specimens for the both two kinds of potatoes.
Overall, as shown in and , it can be obtained obviously that for longitude sampling and transverse sampling, their tensile curve characteristics were basically the same, tending to coincide. The difference of tensile strength or modulus of elasticity was not significant, as listed in . Therefore, in the subsequent finite element simulation study of the potato collision, the peel can be considered as an isotropic material. Furthermore, ‘Yangyu 4’ presented greater resistance to deformation than the ‘Helan 14’ for longitudinal and transverse sampling and less susceptible to damage during harvesting, handling, storage and transportation than ‘Helan 14’. Therefore, it is necessary to clamp potatoes in longitudinal direction when designing transporting and storing machines.
Tear test
The tear load–displacement curves of the ‘Yangyu 4’ and ‘Helan 14’ potato peels are shown in and , respectively. As the figures show, the peel tear curves are with many peaks. The destruction of the sample started from the incision in the process of the tear test and there was a linear stretch back from the incision. The incision as the place of stress concentration was the starting point of the tearing of the peel. Tearing sample is shown in ).
presents the tear force and tear strength values of two kinds of peel samples which sampling in both of the longitude and transverse directions. Comparing with , it can be concluded that the tear force of two kinds of peel specimens were much smaller than the tensile fracture force. It can be inferred that the failure mode of peel tear was mainly cell and cell peeling off, while the failure mode of tensile fracture was mainly the simultaneous fracture caused by stress between cells. This is consistent with the conclusion of Ref.[Citation13]
Table 2. Mean value and standard error of torn strength of potato peels.
shows that the average tear strength values of the transverse sampling were slightly greater than those of the longitudinal sampling for both two kinds of potato peels, but no significant effect was observed and no significant effect was observed between both cultivars either.
Meanwhile, it can be seen that the average tear strength of ‘Helan 14’ potato peel was stronger than that of ‘Yangyu 4’ which indicates that the tear strength of ‘Helan 14’ can be used as an index to evaluate the tearing performance of potato peel.
Microscopic structure of peel
shows field emission scanning electron microscopy (FESEM) micrographs of the epidermis surface of ‘Yangyu 4’ potato peel. It can be seen that many compact and regular polygonal similar-grain structures distributed on the epidermis surface and stoma, and microcrack basically did not exist on the peel. As shown in ), the similar-grain structures were polygons such as square or rectangular structures. The size range of the narrow portion of the grain was and
for the wider portion size range, as shown in ). We conclude that the compact and regular polygonal grain structure leaded to the tensile fracture resistance of the pericarp.
Moreover, at the central region of the observed epidermis, many spherical micro-convex structures distributed in the similar-grain structures, as depicted in . Especially at the grain grid boundaries, the spherical micro-convex structures arranged closely and linearly, as shown in ). The size distribution of the spherical micro-convex structures was . It is deduced that rough surface texture with spherical micro-convex structures was one of the reasons for its outstanding friction coefficient.
depicts the microscopic structure of cross-sections of the potato peel. In the cross-section images it can be seen that the peel consists of multi-layer cells followed by cuticle, epidermis cells and epithelial cells. A corneous layer covered the outer surface epidermis cells, with the epidermis cells protecting and preserving the fruit.[Citation41] As can be seen from ), the cuticle layer of the peel was bumpy, while the epidermis layer was composed of a single layer and its cells were shaped like long strips. From ), it can be observed that the cuticle of the peel had a uniform and consistent arrangement. The circular or elliptic cells of the peel had higher tensile strength than the elongated cells. A more round cell formation may provide higher rupture stress than peel formed by elongated cells.[Citation13] It is deduced that the bearing capacity of the peel depended on the number and distribution of grid structures on the surface, and the size and shape of the epidermal cells. The organization and connections between the cells determined the strength of the connections between cells.[Citation18]
Combined the results of tensile test with the scanning electron micrographs, it can be concluded that the mechanical properties were closely related to the peel microstructure. As shown in ), the fracture surface of the peel presented an irregular cross section and lots of wavy line shape, showing incomplete brittle fracture, possess tear-resistant viscoelastic characteristics. That consistent with that the stress–strain curve does not quickly become zero but has a small extension when the maximum tensile strength starts to break, as seen in and . It can be inferred that the breakdown process of the potato peel is mainly carried out through the cell’s fracture mode.
shows the tear fracture morphology microstructure of potato peel, at both ends of the crack. It was obvious that some of the cuticle was pulled out and peeled off. That’s to mean, peeling tears was mainly carried out in the form of cell-cell exfoliation. This is why the force–displacement curve obtained exhibits a multimodal curve in the tear test. At the beginning of the tear test, the crack at the incision was first torn apart as the stress concentration. Although there is a medium between cell and cell, relative sliding can be achieved, the surface of the skin is covered with a non-flowable stratum corneum which caused epidermal cells were locked by the stratum corneum and weaken the relative sliding between cells. Thence, as the stressed cells were peeled from each other, one of the highest peaks in the tear curve was obtained during the process of tearing and then followed by other peaks. Valleys in the curve represent the load caused by the deformation between the unpeeled-off cells during the tearing process. This strongly explains the results of and .
During the harvesting, packaging, storage, and transportation of potatoes, peel fragmentation will occur. Fragmentation of the peel can seriously affect the appearance and quality of the potato. Therefore, the physical method of shading and water cooling can be used to protect the skin when potatoes are stored.
Considering the mechanical properties and microstructure of peel, fragmentation of potato peel is closely related to its elasticity. That’s to say, knowledge of the relationship between the macroscopic mechanical properties and the microstructure of potato peel can help to cultivate good potato by advanced breeding methods, and thereby solving the problem of broken peel. Moreover, the packaging of potatoes with a flexible cushioning material also reduces the breakage of the peel during the transport of potatoes.
Conclusion
In this paper, the biomechanical characteristics of peels from two potato cultivars were studied. Three measurement methods were successfully applied in order to test tensile strength, elastic modulus, fracture strain, tear strength, and static friction coefficient. When the peel is under tensile stress, the stress–strain curve presents an approximate S-shape, which provides some reference to build a nonlinear model of the peel material. In the friction performance test process, it was observed that the friction coefficient of potato peel against rubber materials is larger than that against metallic materials, which can be used as an index to evaluate the friction performance of potato peel. The experimental results indicated that the static friction coefficients of metallic materials against potato peel were less than those of rubber materials against potato peel. Therefore, friction–coefficient–reducing coating can be used on digging and transporting components with biomimetic techniques to provide a theoretical basis for the design of biomimetic and drag reducing digging devices. In addition, metallic materials on the separating and transporting components can be replaced by rubber materials, which can increase the static friction between potato peel and separating device as well as transporting device so as to increase the transporting ability and reduce the damage to potato peel. The tensile properties of the potato peel showed that the potato peel was a kind of heterogeneous and anisotropic materials without obvious bioyield point. ‘Yangyu 4’ was stronger in the aspect of longitudinal and transverse deformation resistance than ‘Helan 14’ and weaker in that of susceptibility to injury in the process of plucking, managing, storing and transporting so that it is necessary to clamp potatoes in longitudinal direction when designing transporting and storing machines. The average tear strength of the potato peel on the ‘Helan 14’ was greater than that of ‘Yangyu 4’, which indicated that the tear strength of ‘Helan 14’ could be used as an indicator of potato tear performance.
Combined the results of test with the scanning electron micrographs, it can be concluded that the mechanical properties are closely related to the peel microstructure. The macro mechanical mechanical properties of potato peel were explained from a microscopic point of view. We can conclude that the rough surface texture with spherical micro-convex structures is one of the reasons for its outstanding friction coefficient. The compact and regular polygonal grain structure of epidermal cells leads to the larger tensile fracture resistance of the pericarp as well as the anti-shear capacity of peel. From the results of test, we can obtain that the tear force of two kinds of peel specimens are much smaller than the tensile fracture force, which inferred that the failure mode of peel tear is mainly cell and cell peeling off, while the failure mode of tensile fracture is mainly the simultaneous fracture caused by stress between cells and cells. Moreover, the fracture morphology of fresh peels reflected a viscoelasticity behavior.
Relating the mechanical properties and microstructure of peel, fragmentation of potato peel is closely related to its elasticity. In practice, the physical method of shading and water cooling can be used to protect the peel when potatoes are stored. Another way is advanced breeding methods, which can be used to achieve superior breeding of potato varieties, thereby solving the problem of broken peel. Also, the packaging of potatoes with a flexible cushioning material also reduces the breakage of the peel during the transport of potatoes.
The results of this paper provide basic technical parameters and mechanical indexes to fruit properties for establishing the fracture prediction model of peels from the microscopic point of view. As a food species with long storage time, its excellent storage performance has a close relationship with its special microstructure of the epidermis. Therefore, the results of this study provide basic data and ideas for the follow-up potato peel research as well as some fundamental work in support of biomimetic materials prototype selection for water resistance and fresh-keeping of fruit and vegetable skin packaging.
In order to study the relationship between the microstructure of the peel and the macroscopic mechanical properties more comprehensively, the deformation and failure response of microstructures of different potatoes peel under external force will be analyzed by using micromechanical theory and this work will be ongoing.
Additional information
Funding
References
- Yongmei, W.; Wei, S.; Ping, W. G. Research on Mechanical Damage to Potato in Harvesting. Journal of Anhui Agricuture. 2014, 42, 9, 2837–2840.
- Ying, S. Y.; Dongxiang, Z.; Meimei, Z. Study on Bruising Damage Experiment of Potato and Finite Element Analysis. Journal of China Agricultural University. 2008, 13, 1, 81–84.
- Yongmei, W.; Wei, S.; Guanping, W. Experimental Research on Compression Mechanical Properties of Potato. Journal of Agricultural Mechanization Research 2015, 3, 170–174.
- Xinhui, W.; Yuming, G. Effect of High Pulsed Electrical Filed on Properties of Compressive Mechanical and Deformation Process of Potato. Transaction of the Chinese Society for Agricultural Machine. 2013, 44, S2, 181–185.
- Romano, A.; Masi, P.; Aversano, R.; Carucci, F.; Palomba, S.; Carputo, D. Microstructure and tuber properties of potato varieties with different genetic profiles. Food Chemistry 2018, 239, 789–796.
- Strehmel, N.; Praeger, U.; Konig, C.; Fehrle, I.; Erban, A.; Geyer, M.; Kopka, J.; van Dongen, JT. Time course effects on primary metabolism of potato (Solanum tuberosum) tuber tissue after mechanical impact. Postharvest Biology and Technology 2010, 56, 109–116.
- Wenbin, G.; Chunguang, W.; Jinging, G. Correlation between Starch Content and Parameters in Viscoelastic Model of Potato Tuber. Journal of Agricultural Mechanization Research 2014, 1, 190–193.
- Wang Chenglong; Li Xiaoyu; Wu Zhenzhong; Zhou Zhu; Feng Yaoze. Machine vision detecting potato mechanical damage based on manifold learning algorithm. Transactions of the Chinese Society of Agricultural Engineering (Transactions of the CSAE) 2014, 30(1), 245–252.
- Shi Linrong; Wu Jianmin; Sun Wei; Zhao Wuyun; Wang Lijuan; Zhang Fengwei. Mechanical compression of whole tuberp potato. Journal of Northwest A & F University (Natural Science Version) 2014, 42(5), 189-195, 204.
- Wenbin, G.; Chunguang, W.; Baishun, L. Potato Stress Relaxation Properties. Agricultural Machine Journal. 2008, 39, 2, 205–207.
- Juxia, W.; Qingliang, C.; HonT 1040.3-2006o, L.; Yaping, L. Mechanical Properties and Microstructure of Apple Peel during Storage. International Journal of Food Properties 2017, 20(5), 1159–1173. DOI: 10.1080/10942912.2016.1203934.
- Wang Juxia; Cui Qingliang; Li HonT 1040.3-2006o; Liu Yaping; Wang Yu. Evaluation on peels texture of different apple cultivars based on rheological properties. Transactions of the Chinese Society of Agricultural Engineering (Transactions of the CSAE) 2016, 32(21), 305–314.
- Juxia, W.; Qingliang, C.; HonT 1040.3-2006o, L.; Yaping, L. Experimental Research on Mechanical Properties of Apple Peel. Journal of Engineering and Technology Science 2015, 47(6), 688–705. DOI: 10.5614/j.eng.technol.sci.2015.47.6.8.
- Qing Yanmei; Li Changyou; Huang Handong; Cao Yuhua. Finite Element Analysis on Mechanical Properties of Longan. Transactions of the Chinese Society for Agricultural Machine 2011, 42(6), 143–147.
- Rong, W.; Qunying, J.; Deqiang, W. Macromechanic Parameters of Grapes and Tomatoes. Transactions of the Chinese Society of Agricultural Engineering. 2004, 20, 2, 54–57.
- Andrews J; Adams SR; Burton KS; Edmondson, RN. Partial purification of Tomato fruit peroxidase and its effect on the mechanical Properties of tomato fruit peel. Journal of Experimental Botany 2002, 53 (379), 2393-2399.
- Hetzronia, A.; Vana, A.; Mizrach, A. Biomechanical Characteristics of Tomato Fruit Peel. Postharvest Biology and Technology 2011, 59(1), 80–84. DOI: 10.1016/j.postharvbio.2010.08.008.
- Allende, A.; Desmet, M.; Vanstreels, E.; Verlinden, B. E.; Nicolaï, B. M. Micromechanical and Geometrical Properties of Tomato Skin Related to Differences in Puncture Injury Susceptibility. Postharvest Biology and Technology 2004, 34(2), 131–141. DOI: 10.1016/j.postharvbio.2004.05.007.
- Grimm, E.; Khanal, B. P.; Winkler, A.; Knoche, M.; Köpcke, D. Structural and Physiological Changes Associated with the Skin Spot Disorder in Apple. Postharvest Biology and Technology 2012, 64, 111–118. DOI: 10.1016/j.postharvbio.2011.10.004.
- Singh, K. K.; Reddy, B. S. Post-Harvest Physico-Mechanical Properties of Orange Peel and Fruit. Journal of Food Engineering 2006, 73(2), 112–120. DOI: 10.1016/j.jfoodeng.2005.01.010.
- Guo Shilu; Wang Weibing; Li Meng; CHEN Shaojie; LI Junhu. Research on The test of potato mechanism and finite element analysis for Crash. Machine Design and Manufacturing Engineering 2016, 45(1), 56-59.
- Wu Jie; li Fan; Ge Yun; Hu Rong. Measurement of contact pressure of Korla pear under compression and bruising predication using finite element analysis. Transactions of the Chinese Society of Agricultural Engineering (Transactions of the CSAE) 2013, 29(6), 261–266.
- Lixin, L.; Zhiwei, W. Dropping Impact Mechanical Characters Tics of Apple. Transactions of the Chinese Society of Agricultural Engineering. 2007, 23, 2, 254–258.
- Scanlon, M. G.; Long, A. E. Fracture Strengths of Potato Tissue under Compression and Tension at Two Rates of Loading. Food Reseach International 1995, 28(4), 397–402. DOI: 10.1016/0963-9969(95)00013-C.
- Armando, A.; Jaidith, J.; Guillermo, A. Caracterización De Las Propiedades Mecánicas De Dos Variedades De Batata (Ipomoea Batatas Lam). Información Tecnológica 2015, 26(4), 75–80. DOI: 10.4067/S0718-07642015000400010.
- Kristiawan, M.; Chaunier, L.; Della Valle, G.; Lourdin, D.; Guessasma, S. Linear Viscoelastic Properties of Extruded Amorphous Potato Starch as a Function of Temperature and Moisture Content. Rheol Acta 2016, 55, 597–611. DOI: 10.1007/s00397-016-0935-6.
- Liu Jianfeng; Li Guohuai; Peng Shu’ang; Cheng Yunqing. Structure and Storage Quality of Pyrusussuriensis Fruits. Acta Horticulture Sinica 2007, 34(4), 1007–1010.
- Singh, F.; Katiyar, V. K.; Singh, B. P. Analytical Study of Turgor Pressure in Apple and Potato Tissues. Postharvest Biology and Technology 2014, 89, 44–48. DOI: 10.1016/j.postharvbio.2013.11.007.
- Li, M.; Tian, S. L.; Shen, J.; Wang, X. Z.; Cheng, J. X.; Li, S. Q.; Ge, X; Tian, JC. Effects of Chlorine Dioxide on Morphology and Ultrastructure of Fusarium Sulphureum and Its Virulence to Potato Tubers. International Journal of Agricultural and Biological Engineering 2017, 10(5), 242–250.
- Agata, K.; Differences in the fruit peel structures between two apple cultivars during storage. Acta Scientiarum Polonorum-Hortorum Cultus. 2012, 11, 2, 105–116.
- Maguire, KM; Lang, A; Banks, NH; Hall, A; Hopcroft, D; Bennett, R. Relationship between Water Vapour Permeance of Apples and Micro-Cracking of the Cuticle. Postharvest Biology and Technology 1999, 17(2), 89–96.
- Han, YR; He, LM; Luo, XM; Lu, YL; Shi, KY; Chen, JH; Huang, X. A Review of the Recent Advances in Design of Corrugated Plate Packs Applied for Oil-Water Separation. Journal of Industrial and Engineering Chemistry 2017, 53, 37–50. DOI: 10.1016/j.jiec.2017.04.029.
- Wu, Tong; Yu, Suihuai; Chen, Dengkai; Wang, Yanen. Bionic Design, Materials and Performance of Bone Tissue Scaffolds. Materials 2017, 10(10), 1187.
- Yuan, YP; Yu, XP; Yang, XJ; Xiao, YM; Xiang, B; Wang, Y. Bionic Building Energy Efficiency and Bionic Green Architecture: A Review. Renewable & Sustainable Energy Reviews 2017, 74, 771–787. DOI: 10.1016/j.rser.2017.03.004.
- Zhan,XL; Jin,BY; Zhang,QH; Chen, FQ. Design and Applications of Multifunctional Super-Wetting Materials. PROGRESS in CHEMISTRY 2018, 30(1), 87–100.
- T 1040.3-2006/T 1040.3-2006. Plastics––Determination of Tensile Properties––Part 3, Film with the Test Conditions of the Flakes, Standardization Administration of China: Beijing, China (Text in Chinese). 2006.
- T 1040.3-2006/T 16578. 1-2008. Plastics–Film and Sheeting–Determination of Tear Resistance–Part 1, Trouser Tear Method; Standardization Administration of China: Beijing, China (Text in Chinese). 2008.
- T 1040.3-2006/T 3917.2-2009. Textile Fabric–Tearing Performance–Part 2,Breeches Sample (Single Slit) Determination of the Tear Strength; Standardization Administration of China: Beijing, China (Text in Chinese). 2009.
- Jackman, R. L.; Marangoni, A. G.; Stanley, D. W. The Effects of Turgor Pressure on the Puncture and Viscoelastic Properties on Tomato Tissue. Journal of Texture Studies 1992, 23, 491–505. DOI: 10.1111/j.1745-4603.1992.tb00036.x.
- Hong, Y.; Xiang Bian, J. Pear Young Fruit Skin Tissue Created Guo Dian Anatomic Observation. Journal of Fruit Trees. 2002, 12, 1, 62–63.
- Koch, K.; Bhushan, B.; Barthlott, W. Multifunctional Surface Structures of Plants. An Inspiration for Biomimetics, Progress Materials Sciences 2009, 54, 37–178.