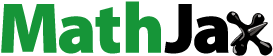
ABSTRACT
A series of starch–fatty acid samples were prepared using potato starch and four fatty acids differing in their chain length, including lauric (C12), myristic (C14), palmitic (C16), and stearic (C18) acids. The results indicated that the fatty-acid chain length played a significant role in altering the properties of potato starch–fatty acid complexes. The complexing index of potato starch–fatty acid complexes decreased from 0.38 to 0.18 with increasing carbon-chain length. V-type crystalline polymorphs were formed between starch and four fatty acids, with shorter chain fatty acids preserving more crystalline structure. X-ray diffraction studies revealed that the degree of crystallinity exhibited by the starch samples was dependent on the fatty-acid chain length. In the Fourier transformed infrared spectrum of the samples, the new spikes at 2917, 2850, 1018, and 720 cm−1 were assumed to be related to the presence of fatty long chains. The formation of amylose–fatty acid complex inhibited granule swelling of potato starch, w\ith longer chain fatty acids showing greater inhibition. Scanning electron microscopy microscopic examination indicated that amylose–fatty acid interactions taken place during starch gelatinization retarded the destruction of the granules.
Introduction
Starch is mainly composed of two components, including linear amylose and highly branched amylopectin. Lipids in starchy foods play important roles in the caloric density, texture, and flavor of foods, which alter the physical and chemical properties of starchy foods.[Citation1] Starch, especially its linear amylose fraction, can interact with endogenous or added lipids to form single-helix complexes.[Citation2] It is well known that amylose forms helical structure with a hydrophobic cavity that could include hydrophobic ligands such as iodine, lipids, or some fat-soluble bioactive substances.[Citation3,Citation4] Marinopoulou[Citation5] reported that the cavity of the amylose helix was hydrophobic and the fatty acid molecules were organized inside the helix as monomers followed head to head and tail to tail, while the carboxyl groups of the acid molecules, due to steric hindrance, remain outside the helix. Amylopectin also formed complexes with fatty acids, but small changes were observed in the functional properties of waxy wheat starch with the addition of fatty acids.[Citation6]
The starch and fatty acids complexes has been reported that influenced by many factors, such as the origins of starch[Citation6] and amylose chain length,[Citation7] complex environment.[Citation5,Citation8–Citation10] Besides, the type of fatty acid also played an important role in the complexes. The stearic acid was reported more strongly bound with amylose than oleic acid and linoleic acid.[Citation11] The chain length of fatty acid was reported played a role in retarding disruption of the granules. For example, myristic acid (C14) did not prevent the disruption of the pea starch granules, whereas stearic acid (C18) was able to prevent granules disruption effectively.[Citation12] The effects of adding monoglycerides (or monoacylglycerols) depended on the chain length and saturation of the fatty acid of the monoglyceride. Vasiliadou[Citation13] showed that at temperatures below 85°C the degree of amylose–fatty acid complexation was fatty-acid chain length dependent that is the shorter the fatty-acid chain length the more the available amylose interacted with the fatty acid when the addition of the acid was done prior to heating.
Starch granules can swell at a limited level, rupture partly and thus keep identifiable shape by controlling the condition of gelatinization, called partially gelatinization.[Citation14] The temperature at which starch and lipid complexed was usually over 80°C, where the starch completely gelatinized and the molecular chains were in a fully expanded state.[Citation15,Citation16] While the situation that the complexation happened in the case of starch granule just swelling has not been reported yet. After pasting, the starch pastes undergo aging or retrogradation when it is cooled or stored. Many starchy-based foods have poor quality in the storage process, such as the aging of bread, the viscosity of rice soup decreased and appeared precipitation. The starch complexed with lipid just started to swell at 63°C, which is the onset gelatinization temperature of potato starch, can maintain the original state of starch granules, which is conducive to the subsequent processing and to minimize the aging of starchy foods. In addition, the potato starch would have different properties in starch–fatty acid complexes because of its large particle size and containing negatively charged phosphate groups.
In this paper, the objective of this work was to study the effect of the properties of fatty acids with different carbon chains compounded with potato starch under partially gelatinization. The effects of fatty acid types on the complexing index (CI), pasting properties as well as microstructures of potato starch–lipid complex were studied.
Materials and methods
Materials
Potato starch was obtained from Xue Guan Starch Company (Ningxia, China). Lauric (C12), myristic (C14), palmitic (C16), or stearic (C18) acids were purchased from Sigma-Aldrich (St. Louis, MO). Other chemical reagents were of all analytical grades.
Starch–fatty acids complexes preparation
Starch suspension (10%, w/w) was heated at 63°C (the onset gelatinization temperature of potato starch) for 30 min in a water bath with constant stirring and then added with four fatty acids completely dissolved in ethanol. The mixture was heated at 63°C for 30 min. After complexed, the mixture was quickly cooled to room temperature. Ethanol was added to remove excess fatty acids in the system and then centrifuged at 1500g for 20 min. Washed three times to ensure that there were no free fatty acids in the systems anymore (Beckman Coulter Inc., Fullerton, CA, USA). The samples were freeze-dried and stored.
Complexing index
The CI of starch was measured by the method of Wang[Citation6] with modifications as follows. Starch–fatty acid mixtures (0.4 g) were weighted accurately into a 50-mL centrifuge tube and then distilled water was added to a total weight of 5 g. The starch suspension was heated in a boiling water-bath for 10 min to fully gelatinize starch. After cooling to room temperature, 25 mL distilled water was added to the above gelatinized starch samples and vortexed. Then, 500 µL of the samples were and added with 15 mL distilled water, and followed by the addition of 2 mL iodine solution (2.0% KI and 1.3% I2 in distilled water). The absorbance at 690 nm was measured. Starch treated in the same manner but without fatty acid addition was used as a reference. CI was calculated as follows:
where AbsRef was the absorbance of the starch solution without added fatty acids, and AbsStarch–lipid was the absorbance of the starch solution with added fatty acids.
X-ray diffraction pattern
The X-ray diffraction (XRD) patterns were measured using X-ray diffractometer (LabX XRD-6000, Shimadzu Corp, Kyoto, Japan) with a Ni-filtered CuKα (λ = 0.15418 nm, 40 kV, 40 mA) radiation. Data were collected from 2θ of 5–40° with a scanning speed of 10°/min at room temperature.[Citation17]
Fourier transformed infrared spectroscopy
The preparation of mixtures was the same as above. The Fourier transformed infrared (FTIR) spectra of samples (3 mg) in KBr (300 mg) disks were recorded in the range of 4000–400 cm−1 at the 4 cm−1 resolution; data were obtained with a FTIR meter (Nexus 470, Thermo Nicolct, USA).[Citation18]
Swelling power and solubility
Swelling power and solubility of starch mixtures were determined by the method of Wang.[Citation6] The suspension of starch mixtures (2%, w/v) was heated at 60°C for 20 min in a water bath with constant stirring, followed by rapidly cooling in ice water to room temperature. The suspension was then centrifuged at 4000 rpm for 20 min (Beckman Coulter Inc., Fullerton, CA, USA). Supernatant was collected and the residue weighed for swelling power determination. The ratio between the residue and initial dry matter was calculated for the swelling power. Amylose leaching was for solubility estimated as described by Ref. [Citation19].
Thermal properties
Thermal properties of the blend starch samples were measured using a DSC-204F1 (Netzsch, Bavarian, Germany). Starch sample (5 mg) was weighed and placed into standard aluminum differential scanning calorimeter (DSC) pan. Distilled water (10 μL) was then added to each pan with a micropipette. An empty pan was served as a reference. Samples were hermetically sealed and equilibrated at 30°C for 12 h before the analysis. The cells were heated from 20 to 150°C at a rate of 5 K min−1. The gelatinization process beginning temperature (To), the peak temperature (Tp), the conclusion temperature (Tc), the gelatinization temperature range (Tc–To), and the gelatinization enthalpy (ΔH) was obtained.[Citation20]
Particle-size distributions
Particle-size distribution was determined using a Mastersize 2000 laser diffraction instrument (Malvern Mastersizer 2000, Malvern Instruments Ltd., Malvern, UK) in wet-cell mode. The starch was added to the reservoir and sonicated for 30 s until an obscuration value of 12% was achieved. The refractive indices used for water and starch were 1.33 and 1.50, respectively. The results were presented as diameter according to volume. The surface-weighted mean diameter D [3, 2] and the volume-weighted mean diameter D [4, 3] of separated granules were measured.[Citation21]
Scanning electron microscopy of starch
Samples of amylose–fatty acid complexes were attached to double-sided adhesive tape, mounted on an aluminum specimen holder, and sputter-coated with a thin film of gold under vacuum. Samples were observed using a JEOL scanning electron microscope (JSM 5410LV, JEOL, Japan) at an accelerating voltage of 20 kV.[Citation22]
Dynamic rheological measurements
A dynamic rheometer (AR2000ex Rheometer, TA Instruments Ltd., New Castle, DE, USA) was used to determine the rheological properties of potato starch–fatty acid complexes. Starch suspensions of 6% (w/v) were stirred with mild agitation provided by a magnetic stirrer for 20 min at room temperature and were immediately conducted to the rheometer plate with a parallel plate system (40 mm in diameter) in order to carry out rheological tests. The gap size was set at 1000 μm. The sample edge was covered with a thin layer of silicone oil to minimize evaporation loss before measurement. During the temperature sweep, strain and frequency were set at 2% and 1 Hz, which were within the linear viscoelastic region. The starch samples were heated from 20 to 100°C and cooled from 100 to 20°C at a rate of 5°C min−1. Storage modulus (G′) and loss modulus (G″) were determined.[Citation23]
Statistical analysis
All experiments were conducted in triplicates. Results were reported as mean values ± standard deviation. For data analysis, the analysis of variance was performed using a SPSS package (SPSS 17.0 for Windows, SPSS Inc., Chicago, IL, USA). Differences among the mean values of various treatments were made using Duncan’s multiple range test (p < 0.05).
Results and discussion
CI
CI was the percentage complexation between fatty acids and starch. showed the CI for different samples. With the increase of the fatty-acid chain length, the CI of potato starch–fatty acid complexes which decreased significantly (p < 0.05) () indicated that the ability to compound with starch molecules decreased for fatty acids with longer chains. This may be due to the steric hindrance effect of long chain fatty acids. According to different guest compounds, amylose forms 6-, 7-, or 8-fold left-handed single helices stabilized by hydrogen bonds.[Citation24] In general, the more complicated of lipid moleculars with longer chain length fatty acids, the larger cavities of the amylose helices required to accommodate the guest molecules.[Citation12,Citation24] The longer the fatty acid chain means there was more difficulty of the fatty acid molecule entering the starch spiral structure,[Citation25] thus, the chances of forming the complex reduced and exhibited lower CI. And shorter chain fatty acids could be better dispersed in gelatinized starch, thus facilitating their interaction with amylose.[Citation6] With the increase of fatty-acid chain length, its viscosity increases, which leads to slow down the rate of movement, and starch molecular chain complex degree of decline. Similar results were also reported in previous studies that short-chain fatty acids formed complex more easily than long-chain fatty acids in starch–fatty acid complexes.[Citation2,Citation6,Citation26,Citation27] Ref. [Citation6] found that amylopectin molecules also interacted with fatty acids to form complexes, but carbon chain length of fatty acids was not the determinant for the complexation of amylopectin. Therefore, it could be thought that the fatty acids here mainly interacted with amylose molecules in potato starch and fatty-acid chain length played an important role in the properties of complexes.
Table 1. The complex index, swelling power, solubility, and granularity parameters of potato starch starch–fatty acids complexes.
XRD
The XRD patterns of potato starch with or without fatty acids were shown in . Single fatty acid exhibited a sharp peak at 21.6° with strong diffraction signal, and four other peaks around 6.8°, 20.6°, 24°, and 36°, respectively, showed relatively weak signals. Potato starch with four fatty acids exhibited the X-ray pattern with reflection intensities centered at 17.4°, 20°, 22°, and 24°(2θ). Compared with pure starch, the diffraction peaks decreased at 17.4°, while the peaks at 22° and 24° significantly increased with the introduction of fatty acids, indicating the typical V-type pattern for amylose–lipid complexes (). Besides, the diffraction peak at 5.8° for the amylose disappeared in the starch–fatty acid complex. Ref. [Citation5] pointed out that amylose–fatty acid complexes prepared at temperatures close to 60°C were in amorphous-like state (type I) whereas at temperatures higher than 90°C, the complexes were in semi-crystalline state (type II). The starch–fatty acids complexes were prepared at 63°C and combined with XRD that could be considered that the complexes were type I crystallization.
The relative crystallinity of starch–fatty acid complex decreased with the addition of fatty acids, comparing with nature starch, and the crystallinity decreased gradually with the increase of the carbon chain length of the fatty acid (). The relative crystallinity of the potato starch–lauric acid (C12) complex was much higher than starch–C18 complex. When starch granules were heated in the presence of excess water, the granules partially swelled because of the loss of the crystalline order and the absorption of water. At the same time, the amylose inside the starch granules leached out and complexed with the fatty acids.[Citation13] Crystallinity decreases with increasing fatty-acid chain length, suggesting that fatty acid interfered with the association of the starch polymers and a less-organized structure was formed, which might be due to the steric hindrance of the alkyl chains impacted the recrystallization of starch molecules and the steric hindrance increasing with the fatty chain length.[Citation25] Besides, the amylose–fatty acids complexes were probably V-type single helical amylose pattern; thus, there was no formation of double helices, junction zones, and gel network, which exhibited lower crystallinity.[Citation28]
The V-type crystalline polymorphs were formed between normal wheat starch and three fatty acids, with shorter chain fatty acids producing more crystalline structure. Refs. [Citation6,Citation29] found that the Hylon VII-complexes with stearic and palmitic acid exhibited lower degrees of crystallinity than the complexes with myristic acid, explaining that the long chain length fatty acids (stearic and palmitic acid) due to their increased stiffness were more difficult to associate themselves to form arranged semi-crystalline structures.
FTIR
showed the FTIR spectra of the fatty acids (lauric, myristic, palmitic, and stearic acid) and the FTIR spectra of the potato starch with various fatty acids. In the FTIR spectrum of the samples, potato starch had a wider peak at 2927 cm−1, which was attributed to the stretching vibration peak of C–H in starch molecular chain. As the fatty acids added into potato starch, the broad peak here became two new spikes, with peaks at 2917 and 2850 cm−1, respectively. This might be corresponding to antisymmetric and symmetric stretching of CH2 and CH3 for the presence of fatty long chains.
Figure 2. FTIR spectra of potato starch and its fatty acids complexes. A:PS; B:PS-C12; C:PS-C14; D:PS-C16; E:PS-C18.
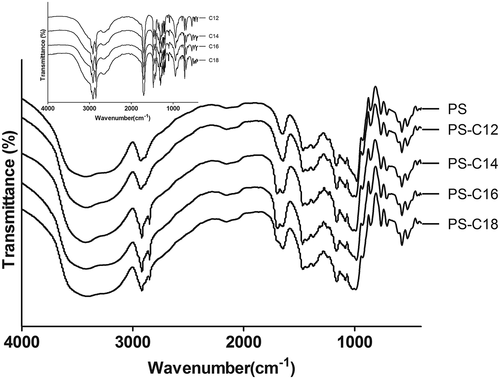
In all spectra of the fatty acids, it could be seen that the characteristic peak at 1700 cm−1 attributed to the absorbance of the carbonyl group was present. There was another new weak band at 1702 cm−1 appeared in the potato starch and fatty acids complexes. This shift of the carbonyl group peak at 1700 cm−1 for the free fatty acid was to a higher wave length value for the complexed fatty acid. FTIR spectroscopy revealed that the absorption peak attributed to carbonyl group of free fatty acid was shifted when the fatty acid was in the form of amylose complex.[Citation26]
While the strong spikes of fatty acids at 720 cm−1 in fatty acids, attributed to rocking peak of the at least four linearly successive connected methylene groups ((CH2)4), was not present in the complexes. Probably because long chain fatty acids entered into the amylose double helix and bind to starch molecules with hydrogen bonds. Besides the shift of the peak at 3300 cm−1 to the formation of hydrogen bonding between the carbonyl group of the guest molecule and the hydroxyl groups of amylose, it is noted that a small peak at 1018 cm−1 occurred for potato starch-fatty acid samples, which were not observed for pure starch, were assumed to be related to the amylose-fatty acid complex.[Citation6]
Swelling power and solubility
The swelling power of potato starch was significantly increased with the addition of four fatty acids compared with the control (p < 0.05), while with the increase of the fatty acid chain length, the swelling power decreased gradually (A). The solubility of potato starch decreased significantly with the addition of fatty acids (p < 0.05), the effect was more pronounced with the longer the fatty acid chain length (B). That means the addition of fatty acids reduced the swelling force of the starch and inhibited swelling of the starch granules.
The lower swelling power of starch–C18 sample could be attributed to the longer and more stable helices formed between amylose and C18, which was assumed to inhibit swelling of starch granules more effectively than helices formed with other shorter chain fatty acids. Longer chain length resulted in lower starch solubility of potato starch. With the addition of fatty acids, swelling of starch granules was inhibited with the limited leaching of starch polysaccharide molecules. Zhou [Citation30] reported that with the addition of fatty acids (stearic and linoleic acid) can further restrain the starch granule from hydration and swelling by covered the starch surface or formed an amylose–fatty acid complex, which deposited as an insoluble film on the granule surface and delay the transportation of water into the granules. This result confirms the substitution of the hydroxyl groups by alkyl chains leading to hydrophobic for starch. Vanmarcke and Hernández-Hernández [Citation25,Citation31] found crystallization of the amylose–LPC (lysophosphatidylcholine) inclusion complex during gelatinization, which promotes starch granule thermal stability at up to 95°C.
Thermal analysis
The gelatinization temperatures and enthalpy change derived from DSC thermograms as well as DSC curve are shown in and . The single fatty acids had only one DSC endothermic peak, and the peak temperature increased with the length of the fatty acid carbon chain. A single potato starch had an endothermic peak at 60–70°C. The potato starch–fatty acid composite displayed three separated endotherms, and there was significant difference in DSC curve () when the fatty chain length was varied ().
Table 2. The thermal parameters of potato starch–fatty acids complexes.
Figure 3. DSC curve of the potato starch with four fatty acids. A:PS; B: C12;C:PS-C12; D:PS-C14; E:PS-C16; F:PS-C18.
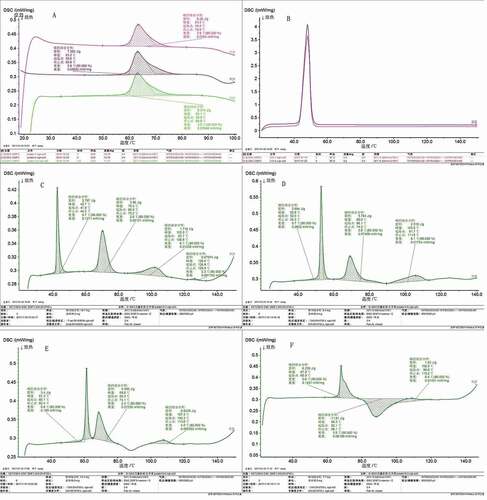
The first endotherm (Peak I) at the lower temperature was attributed to the melting of the free fatty acids, and the peak temperature increased with the length of the fatty acid carbon chain. The second endotherm (Peak II) was for the starch gelatinization endothermic peak, which was partially overlapped with peak I. Compared with pure starch, the temperature of starch gelatinization was slightly elevated added with fatty acids, which may be due to the formation of lipids and amylose complexes, reducing the water into the starch granules, directly expressed as gelatinization temperature increased. As the carbon chain increases, the thermal stability of the complex increases. Dissociation temperatures of amylose–lipid complexes increased with increasing length of hydrocarbon chains of the lipids and decreased with increasing number of olefinic bond in the hydrocarbon chains.[Citation24] Moreover, during the gelatinization process, the formation of the starch–fatty acid complex was exothermic process, while starch gelatinization was an endothermic one. Thus, the amount of heat that needs to be absorbed by starch gelatinization could be partially provided by the starch–lipid complex, and the ΔH value was reduced. At the same time, the ΔH of native starch reflects the loss of double helices or starch crystallites. The great decrease in the ΔH of potato starch upon addition of fatty acids indicated that melting of starch crystallites was inhibited. Thus, the To and Tp increased and ΔH decreased with the addition of fatty acids, indicating that their addition inhibited gelatinization of potato starch.
Peak III was the endothermic peak of potato starch and fatty acids complexes, and the cleavage temperature was more than 110°C. The carbon chain length had an effect on the cracking temperature of the complex (). With the extension of the carbon chain, the hydrophilicity of the fatty acid decreased and the hydrophobicity increased, and the complex was more stable.[Citation5] The ΔH of amylose–lipid complex was a measure of combination of the amount of complex and the degree of order (crystallinity) within the complex. The melting point and corresponding enthalpy increased with chain length, suggesting that there were formed stable compounds of starch with fatty acids. It was interesting to note that starch paste with C18 produced a DSC curve that was less regular than those generated from shorter chain length fatty acids (C12–C16). The possible reason was that there was a small amount of free C18 remaining in the system. The presence of the exothermic peaks confirmed the reorganization of the complexes and the recrystallization of the free fatty acids. The endothermic peak around C18 was assigned to the melting of crystals composed of alkyl chains, which were difficulty removed during washing with the ethanol/water mixture.[Citation5]
Marinopoulou[Citation5] found that in the case of complexes of myristic (C14), palmitic (C16), and stearic (C18) acids, two endothermic peaks were visible, whereas the complexes of decanoic (C10), oleic (C18:1), and linoleic (C18:2) acids exhibited only one endothermic peak, which might be due to its rather short chain length in the case of decanoic acid which renders it highly mobile and easily removed during washing with solvent. Ref. [Citation25] reported that the thermal behavior of starch derivatives was mainly influenced by the length of the alkyl chains, which appeared as an efficient plasticizing route for alkyl chains since the decrease in the interactions between the starch chains as the alkyl chain length increases. In the case of amylomaize (Hylon VII) starch–fatty acid (capric, myristic, palmitic, stearic, oleic) complexes, there were the same situation that the dissociation temperature of the complexes was increased proportionally to the chain length increase of the fatty acid.[Citation29]
Morphologies and size distributions
The morphologies of the potato starch–fatty acids complexes were investigating by using SEM () and its granule size distributions in terms of the surface weighted mean diameter D [3, 2] and the mean volume diameters (D [4, 3]) were illustrated in by Mastersizer. The average diameters (D [4, 3]) of the starch were 32.75, 86.12, 56.99, 42.99, and 86.21 um for the reaction without fatty acids, and with lauric (C12), myristic (C14), palmitic (C16), or stearic (C18) acids, respectively, that means the large granule size for PS-C12 and PS-C18. Fatty acids were added into starch under partial gelatinization (63°C) for complexing. In this process, the starch was pregelatinized, but the control group did not. For PS-C12, it’s caused by the pregelatinized of starch granule. While for PS-C18, stearic acid did not diffuse in starch granules but coat around it. At low temperature (63°C), few amylose molecules would exit from the granules and possibly remained attached to their granule surface like a hair.[Citation13] Thus, they were interacted with myristate to a greater degree than other fatty acids with longer chains which they need amylose molecules with longer chains to be able to complex.[Citation7] SEM microscopic examination indicated that amylose–fatty acid interactions taken place during starch gelatinization retarded the destruction of the granules. Disruption of starch granules became more apparent with the increase of the amount of fatty acids in the mixture. It was reported that stearic acid did not diffuse in teff starch granules but seemed to coat them. However, stearic acid diffused inside maize starch granules through channels.[Citation28]
Rheological properties of starch–fatty acids complexes
The rheological behavior of starch pastes reflects the properties of the swollen starch granules entrapped in a polymer amylose matrix.[Citation32] exhibited the storage modulus (G′) and loss modulus (G″) of potato starch with or without fatty acids during the heating process using the dynamic rheometer. The magnitudes of G′ and G″ for the native potato starch increased first and then decreased gradually for the collapse of the starch granules structure when the heating temperature rised from 50 to 95°C. As addition of fatty acids, G′ and G″ were significantly increased compared with the control. Except C18, the G′ and G″ of starch–fatty acids complexes decreased with fatty acids carbon chains increased from C12 to C16. At the same time, it was found that with the addition of fatty acids, the temperature of G′ and G″ began to increase and the curve gradually moved to the right (high temperature), indicating that the gelatinization of the starch mixed system was delayed ().
Figure 5. Effect of fatty acids on temperature dependences of G′ (a) and G″ (b) of potato starch suspensions during heating.
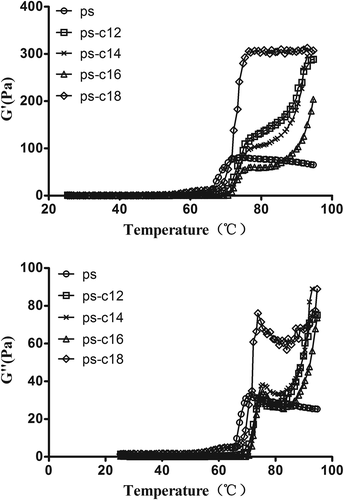
With addition of fatty acids that its carbon chains increased from C12 to C16, a decrease in the storage modulus and loss modulus was observed. There may be two reasons: first, after the addition of fatty acids covering the surface of the starch granules, hindering the water molecules into the interior of the particles; second, the interaction of fatty acids and starch to form complexes inhibits the increase in starch viscosity.
In the high amylose (70%) maize starch and fatty acids system, the myristic containing sample exhibited the higher viscosity values whereas the starch-stearate system exhibited the lower viscosity.[Citation13] Teff starch with stearic acid was more viscous and was non-gelling.[Citation28] While for the fatty acids with C18, an abnormally elevated G′ and G″ might be due to the steric hindrance effect of the C18 chain. There was only a portion of the C18 segments enter the amylose spiral structure, while the remainder was exposed to the outside, which hampered water enter into the starch granules, so that the gelatinization of starch granules was greatly inhibited. The rigidity of the expanded starch granules increased with addition of C18, thus the addition of C18 significantly increased G′ and G″. And besides, long chain fatty acids require more amylose space to complex.[Citation7] But the phosphate groups are located mostly in the long B-chains in potato amylopectin.[Citation33] The presence of phosphate groups hindered the entry of fatty acids into starch granules. Thus, considering the fatty acid carbon chain length as well as steric hindrance effect of potato starch phosphate ester, it hypothesis that the fatty acid with C16 may be most suitable for complexing with potato starch.
Conclusion
The properties of potato starch–fatty acid complexes with various fatty acid chain lengths under partially gelatinization were measured in present work. For potato starch, fatty acids chain length played an important role in the formation of amylose–fatty acid complexes. With the increase of the fatty acid chain length, the CI of potato starch–fatty acid complexes decreased significantly. Compared with pure starch, amylose–lipid complexes exhibited the typical V-type X-ray pattern, and the crystallinity decreased gradually with the increase of the carbon chain length of the fatty acid. The swelling power of potato starch was significantly decreased as fatty acids carbon chains increased, presumably due to the formation of more stable complexes with fatty acids having longer carbon chains that delayed starch swelling. Except stearic acids, the G′ and G″ of starch–fatty acids complexes decreased with fatty acids carbon chains increased from lauric acids to palmitic acids. Based on the results, its hypothesis that the fatty acid with palmitic acids may be most suitable for complexing with potato starch, which means suitable length of fatty acids for the specific starch could form more stable complexes.
Additional information
Funding
References
- Chung, C.; Degner, B.; McClements, D. J. Rheology and Microstructure of Bimodal Particulate Dispersions: Model for Foods Containing Fat Droplets and Starch Granules. Food Research International 2012, 48, 641–649. DOI: 10.1016/j.foodres.2012.06.011.
- Godet, M. C.; Tran, V.; Colonna, P.; Buleon, A.; Pezolet, M. Inclusion/Exclusion of Fatty Acids in Amylose Complexes as a Function of the Fatty Acid Chain Length. International Journal of Biological Macromolecules 1995, 17, 405–408.
- Dhillon, S.; Seetharaman, K. Rheology and Texture of Starch Gels Containing Iodine. Journal of Cereal Science 2011, 54, 374–379. DOI: 10.1016/j.jcs.2011.07.005.
- Lay Ma, U. V.; Floros, J. D.; Ziegler, G. R. Formation of Inclusion Complexes of Starch with Fatty Acid Esters of Bioactive Compounds. Carbohydrate Polymers 201183, 1869–1878.
- Marinopoulou, A.; Papastergiadis, E.; Raphaelides, S. N.; Kontominas, M. G. Structural Characterization and Thermal Properties of Amylose-Fatty Acid Complexes Prepared at Different Temperatures. Food Hydrocolloids 2016, 58, 224–234. DOI: 10.1016/j.foodhyd.2016.02.034.
- Wang, S.; Wang, J.; Yu, J.; Wang, S. Effect of Fatty Acids on Functional Properties of Normal Wheat and Waxy Wheat Starches: A Structural Basis. Food Chemistry 2016, 190, 285–292. DOI: 10.1016/j.foodchem.2015.05.086.
- Godet, M. C.; Bizot, H.; Buléon, A. Crystallization of Amylose—Fatty Acid Complexes Prepared with Different Amylose Chain Lengths. Carbohydrate Polymers 1995, 27, 47–52. DOI: 10.1016/0144-8617(95)00034-5.
- Vasiliadou, E.; Raphaelides, S. N.; Papastergiadis, E. Effect of Heating Time and Temperature on Partially Gelatinized Starch-Fatty Acid Interactions. LWT - Food Science and Technology 2014, 60, 698–707. DOI: 10.1016/j.lwt.2014.10.026.
- Seo, T.-R.; Kim, J.-Y.; Lim, S.-T. Preparation and Characterization of Crystalline Complexes between Amylose and C18 Fatty Acids. LWT - Food Science and Technology 2015, 64, 889–897. DOI: 10.1016/j.lwt.2015.06.021.
- Meng, S.; Ma, Y.; Sun, D.-W.; Wang, L.; Liu, T. Properties of Starch-Palmitic Acid Complexes Prepared by High Pressure Homogenization. Journal of Cereal Science 2014, 59, 25–32. DOI: 10.1016/j.jcs.2013.10.012.
- Liu, P.; Sun, S.; Hou, H.; Dong, H. Effects of Fatty Acids with Different Degree of Unsaturation on Properties of Sweet Potato Starch-Based Films. Food Hydrocolloids 2016, 61, 351–357. DOI: 10.1016/j.foodhyd.2016.05.033.
- Exarhopoulos, S.; Raphaelides, S. N. Morphological and Structural Studies of Thermally Treated Starch-Fatty Acid Systems. Journal of Cereal Science 2012, 55, 139–152. DOI: 10.1016/j.jcs.2011.10.011.
- Vasiliadou, E.; Raphaelides, S. N.; Papastergiadis, E. Effect of Heating Time and Temperature on Partially Gelatinized Starch-Fatty Acid Interactions. LWT - Food Science and Technology 2015, 60, 698–707. DOI: 10.1016/j.lwt.2014.10.026.
- Fu, Z.-Q.; Wang, L.-J.; Zou, H.; Li, D.; Adhikari, B. Studies on the Starch–Water Interactions between Partially Gelatinized Corn Starch and Water during Gelatinization. Carbohydrate Polymers 2014, 101, 727–732. DOI: 10.1016/j.carbpol.2013.09.098.
- Lesmes, U.; Cohen, S. H.; Shener, Y.; Shimoni, E. Effects of Long Chain Fatty Acid Unsaturation on the Structure and Controlled Release Properties of Amylose Complexes. Food Hydrocolloids 2009, 23, 667–675. DOI: 10.1016/j.foodhyd.2008.04.003.
- Raphaelides, S. N.; Georgiadis, N. Effect of Fatty Acids on the Rheological Behaviour of Amylomaize Starch Dispersions during Heating. Food Research International 2008, 41, 75–88. DOI: 10.1016/j.foodres.2007.10.004.
- Nara, S.; Komiya, T. Studies on the Relationship between Water‐Satured State and Crystallinity by the Diffraction Method for Moistened Potato Starch. Starch‐Stärke 1983, 35, 407–410. DOI: 10.1002/(ISSN)1521-379X.
- Kaewtatip, K.; Thongmee, J. Studies on the Structure and Properties of Thermoplastic Starch/Luffa Fiber Composites. Materials & Design 2012, 40, 314–318. DOI: 10.1016/j.matdes.2012.03.053.
- Hoover, R.; Ratnayake, W. S. Starch Characteristics of Black Bean, Chick Pea, Lentil, Navy Bean and Pinto Bean Cultivars Grown in Canada. Food Chemistry 2002, 78, 489–498. DOI: 10.1016/S0308-8146(02)00163-2.
- Ahmad, F. B.; Williams, P. A. Effect of Sugars on the Thermal and Rheological Properties of Sago Starch. Biopolymers 1999, 50, 401–412. DOI: 10.1002/(SICI)1097-0282(19991005)50:4<401::AID-BIP6>3.0.CO;2-V.
- Puncha-Arnon, S.; Pathipanawat, W.; Puttanlek, C.; Rungsardthong, V.; Uttapap, D. Effects of Relative Granule Size and Gelatinization Temperature on Paste and Gel Properties of Starch Blends. Food Research International 2008, 41, 552–561. DOI: 10.1016/j.foodres.2008.03.012.
- Kim, M. J.; Choi, S. J.; Shin, S. I.; Sohn, M. R.; Lee, C. J.; Kim, Y.; Cho,W.I.; Moon, T. W. Resistant glutarate starch from adlay: Preparation and properties. Carbohydrate Polymers 2008, 74(4), 787–796.
- Singh, M.; Singh, O.; Moughan, H.; Kaur, P. J.; Morphological, L. Thermal and Rheological Characterization of Starch Isolated from New Zealand Kamo Kamo (Cucurbita Pepo) Fruit – A Novel Source. Carbohydrate Polymers 2007, 67, 233–244. DOI: 10.1016/j.carbpol.2006.05.021.
- Kong, L.; Ziegler, G. R. Molecular Encapsulation of Ascorbyl Palmitate in Preformed V-Type Starch and Amylose. Carbohydrate Polymers 2014, 111, 256–263. DOI: 10.1016/j.carbpol.2014.04.033.
- Vanmarcke, A.; Leroy, L., Stoclet, G.; Duchatel-Crépy, L.; Lefebvre, J. M.; Joly, N.; Gaucher, V. Influence of fatty chain length and starch composition on structure and properties of fully substituted fatty acid starch esters. Carbohydrate polymers 2017, 164, 249–257.
- Marinopoulou, A.; Papastergiadis, E.; Raphaelides, S. N.; Kontominas, M. G. Morphological Characteristics, Oxidative Stability and Enzymic Hydrolysis of Amylose-Fatty Acid Complexes. Carbohydrate Polymers 2016, 141, 106–115. DOI: 10.1016/j.carbpol.2015.12.062.
- Lim, M. C.; Park, K. H.; Choi, J. H.; Lee, D. H.; Letona, C. A. M.; Baik, M. Y.; Park, C. S.; Kim, Y. R. Effect of short-chain fatty acids on the formation of amylose microparticles by amylosucrase. Carbohydrate polymers 2016, 151, 606–613.
- D’Silva, T. V.; Taylor, J. R. N.; Emmambux, M. N. Enhancement of the Pasting Properties of Teff and Maize Starches through Wet–Heat Processing with Added Stearic Acid. Journal of Cereal Science 2011, 53, 192–197. DOI: 10.1016/j.jcs.2010.12.002.
- Marinopoulou, A.; Papastergiadis, E.; Raphaelides, S. N. An Investigation into the Structure, Morphology and Thermal Properties of Amylomaize Starch-Fatty Acid Complexes Prepared at Different Temperatures. Food Research International 2016, 90, 111–120. DOI: 10.1016/j.foodres.2016.10.035.
- Zhou, Z.; Robards, K.; Helliwell, S.; Blanchard, C. Effect of the Addition of Fatty Acids on Rice Starch Properties. Food Research International 2007, 40, 209–214. DOI: 10.1016/j.foodres.2006.10.006.
- Hernández-Hernández, E.; Ávila-Orta, C. A.; Hsiao, B. S.; Castro-Rosas, J.; Gallegos-Infante, J. A.; Morales-Castro, J.; Ochoa-Martínez, L.; Gómez-Aldapa, C. A. Synchrotron X-ray scattering analysis of the interaction between corn starch and an exogenous lipid during hydrothermal treatment. Journal of cereal science 2011, 54(1), 69–75.
- Wang, B.; Wang, L.-J.; Li, D.; Wei, Q.; Adhikari, B. The Rheological Behavior of Native and High-Pressure Homogenized Waxy Maize Starch Pastes. Carbohydrate Polymers 2012, 88, 481–489. DOI: 10.1016/j.carbpol.2011.12.028.
- Noda, T.; Takigawa, S.; Matsuura-Endo, C. Physicochemical Properties and Amylopectin Structures of Large, Small, and Extremely Small Potato Starch Granules. Carbohydrate Polymers 2005, 60, 245–251. DOI: 10.1016/j.carbpol.2005.01.015.