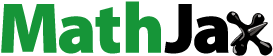
ABSTRACT
In the present study, the alcalase-generated proteolysates obtained after 8 h of proteolysis of stone fish flesh showed the most potent antioxidant activity in terms of DPPH• radical scavenging activity (77.43%, IC50 of 0.5 mg/mL), ABTS• radical scavenging activity (92.73%, IC50 of 0.33 mg/mL) and FRAP value (39.2 mmol/100 mL FeSO4). These proteolysates profiled and characterized as antioxidative peptides. The proteolysates were initially subjected to ultrafiltration using MWCO Spin-X UF. Potent fractions were further characterized based on hydrophobicity using reversed-phase high-performance liquid chromatography (RP-HPLC) and isoelectric point using an OFFGEL isoelectric focusing fractionator. Results indicated that most of the antioxidative peptides found in fractions with a molecular weight (MW) of less than 2 kDa, hydrophilic (hydrophilicity >80%) and basic (pI = 9.7). The final purified fraction with the highest antioxidant activity was selected for peptide identification and sequencing using Q-TOF mass spectrometry. A total of four peptides were identified, from which Peptide 1 (GVSGLHID) showed the highest antioxidant activity and this has potential as a novel bioingredient of nutraceuticals and functional foods to promote human health.
Introduction
Antioxidants have a critical role in minimizing oxidative damage, which causes a wide array of diseases and disorders in the human body as well as affecting the quality and nutrient content of food systems. Therefore, reducing oxidative stress using antioxidants can serve as a key step towards decreasing oxidative stress-related diseases.[Citation1] Given the current popularity of natural products for consumption, significant attention is focused on the identification of safe and natural antioxidants. Recently, the generation and characterization of bioactive peptides from natural sources has also received significant attention because of their safety, availability and nutritional value. Bioactive peptides are typically short-sequence amino acids which are encrypted and inactive within their parent protein, and may exert some biofunctionalities after their release following gastrointestinal digestion, food processing or enzymatic hydrolysis.[Citation1]
Sea cucumbers are rich and available sources of protein, consisting of valuable structurally diverse bioactives with nutraceutical, pharmaceutical and cosmeceutical potential that can be directly used as ingredients in these industries.[Citation2] Enzymatic proteolysis of sea cucumber flesh can be used to prepare bioactive peptides. The antioxidant activities of peptides derived from some species of sea cucumber have been reported in previous studies, but effective antioxidant compounds remain to be isolated and identified from other species.
Stone fish (Actinopyga lecanora) is classified among the edible species of sea cucumbers, but it is typically unpopular and discarded as by-catch. This species of sea cucumber has therefore remained underutilized in recent years. The first study to report the bioactivity potential of stone fish was carried out in 2013, and showed that the enzymatic digestion of stone fish flesh caused the generation of appreciable amounts of antioxidative proteolysates.[Citation3] Different biological activities were subsequently demonstrated for this marine organism such as antimicrobial, antihypertensive and anti-inflammatory activities.[Citation4,Citation5] These bio-functionalities position this inexpensive species of sea cucumber as a valuable potential source of bioactive compounds. Given that proteolysates of stone fish have been shown to exert potent antioxidant activity in previous studies,[Citation3] the present study focused on the purification and characterization of stone fish-derived antioxidative peptides as a potential source of nutraceutical and functional foods ingredients.
Materials and methods
Materials
The alcalase enzyme used for proteolysis was supplied by Novozymes (Denmark). Chemicals used for the assays were 2,2-diphenyl-1-picrylhydrazyl l (DPPH•) and 2, 20-azinobis-(3-ethylbenzothiazoline-6-sulfonic acid) obtained from Sigma-Aldrich, Inc. (St. Louis, MO, USA.) and Calbiochem (Billerica, MA, USA), respectively. Glutathione (GSH), sodium dodecyl sulphate (SDS) and 2,4,6-Tris(2-pyridyl)-s-triazine (TPTZ) were purchased from Acros Organics (Belgium). Coomassie brilliant blue R-250 was supplied by Bio-Rad (USA). Acetonitrile and trifluoroacetic acid (TFA) (HPLC grade) were purchased from Fisher Scientific (Pittsburgh, PA, USA). Other chemicals were all of analytical grade and supplied by Merck (Darmstadt, Germany).
Sample preparation
Freeze-dried stone fish flesh (5 g) was autoclaved at 121°C for 15 min to inactivate endogenous enzymes and microorganisms. The autoclaved sample was then dialyzed for 28 h [first against distilled water (12 h) and then reaction buffer (16 h)] at 4°C under dark conditions. The dialyzed sample was placed in a water bath until the optimum alcalase activity temperature was reached, and alcalase was then added at a ratio of 1/100 (w/w) (enzyme/substrate). Proteolysis was carried out under optimum conditions (pH 7.9 using 50 mM borate buffer and temperature 55°C) for 8 h.[Citation3] Further addition of alcalase at the same ratio took place after 5 h. For proteolysis profiling, one unhydrolyzed sample was first taken just before the addition of enzyme, and the remaining samples were taken at 1 h intervals during proteolysis. The proteolysates were immediately boiled for 10 min at 100°C to inactivate the protease. Samples were then centrifuged at 10,000 × g for 20 min and the resulting supernatant was stored at −80°C until further use.
Methods
Peptide content and degree of proteolysis determination
The o-phthaldialdehyde (OPA)-based spectroscopic technique described by Church et al.[Citation6] was applied. with slight modifications, for profiling of the stone fish proteolysates and degree of proteolysis determination (DP). A 40 µL aliquot of sample was directly added to 300 µL of OPA solution in a 96-well plate and the mixture was incubated for 2 min at room temperature. Absorbance was read at 340 nm using an ELISA plate reader (Power Wave X 340, Biotek Inc. Wilrijk, Belgium). Peptide content was calculated by interpolating values to the glutathione standard curve within a concentration range of 0–200 ppm. Samples were diluted accordingly if the absorbance was out of the linear range of the calibration curve. Distilled water was used as a negative control. The calculation of DP (%) was performed as follows:
where AS is the absorbance of the sample after hydrolysis, AC is the absorbance of the control, AP is the absorbance of the protein solution before adding protease (before proteolysis), and AT is the absorbance of protein after complete hydrolysis (total hydrolysis).
DPPH• radical scavenging assay
DPPH• radical scavenging activity was assayed following the method described by Bersuder, Hole and Smith[Citation7] and Zarei et al.[Citation1] with some modifications. Briefly, 500 µL of diluted sample was added to 1 mL of 20 mM DPPH• dissolved in 80% ethanol. The mixture was incubated for 60 min at room temperature in dark conditions. Next, the samples were centrifuged at 10,000 × g for 3 min and the supernatants were collected. The absorbance of DPPH• reduction was read at 517 nm using a UV-Vis Double Beam spectrophotometer (UVD 2950, Labomed, Inc).
To measure the antioxidant activity of the fractions, 50 μL of each fraction was added into a well of a 96-well plate and mixed with 50 μL deionized water and 100 μL DPPH• reagent to a final volume of 150 μL. The mixture was incubated for 1 h at room temperature in the dark in order to complete the reaction. Absorbance was read at 517 nm using an ELISA plate reader (Power Wave x 340, Biotek Inc. Wilrijk, Belgium). The calculation of DPPH• radical scavenging activity (%) was performed as follows:
Radical scavenging activity (%): (absorbance of negative control − absorbance of sample)/(absorbance of negative control) × 100
GSH and distilled water were used as standard (positive control) and blank (negative control), respectively.
2,2-Azino-bis(3-ethylbenzothiazoline-6-sulphonic acid) assay
The procedure described by Re et al.[Citation8] was followed with slight modifications. The 2,2-azino-bis(3-ethylbenzothiazoline-6-sulphonic acid (ABTS•) stock solution was prepared by adding 7 mM ABTS• to 2.45 mM potassium persulphate. The mixture was incubated for 16 h at room temperature in the dark. To prepare an ABTS• working solution with an absorbance range of 0.80 ± 0.02 at 734 nm, an aliquot of stock solution was diluted with distilled water. Samples (5 mg) were dissolved in 1 mL of distilled water. A 20 μL aliquot of dissolved peptide sample was added to 980 μL of ABTS• working solution. The mixture was incubated for 10 min at 37 ºC in the dark and the reduction of absorbance was measured at 734 nm. The calculation of ABTS• radical scavenging activity (%) was as follows:
Radical scavenging activity (%): (absorbance of negative control − absorbance of sample)/(absorbance of negative control) × 100
GSH and distilled water were used as standard (positive control) and blank (negative control), respectively. The ABTS• radical scavenging activity of peptide samples was expressed as % in 5 mg/mL of sample.
Ferric reducing antioxidant power assay
The ferric reducing antioxidant power (FRAP) value was assayed following the method described by Guo et al.[Citation9] First, fresh FRAP reagent was prepared by adding 2.5 mL of a 10 mM TPTZ (2,4,6-tripyridy-s-triazine) solution to 40 mM HCl plus 2.5 mL of 20 mM FeCl3 and 25 mL of 0.3 mol/L acetate buffer, with pH adjusted to 3.6. The reagent was stored at 37°C. Next, 40 μL aliquots of sample (supernatant of stone fish proteolysate) were added to 1.8 mL of FRAP reagent and the mixture was adjusted to 2 mL with distilled water and incubated at 37°C for 10 min. The reaction absorbance was spectrophotometrically determined at 593 nm using FeSO4 as the standard solution. Results were expressed as the antioxidant concentration having a ferric reduction ability equivalent to that of 1 mM FeSO4. Samples was diluted if the FRAP value was out of the linear range of the calibration curve. Distilled water was used as a negative control.
Physicochemical properties of proteolysates
Emulsifying capacity (EC)
The procedure described by Souissi et al.[Citation10] was followed with slight modifications. Briefly, 0.1 g of freeze-dried proteolysate was dissolved in 10 mL of distilled water and 10 mL of palm oil was added and mixed using a plunging homogenizer (Heidolph homogenizer DIAX 900, Sigma-Aldrich Co.) equipped with a motorized stirrer operating at 10,000 rpm for 3 minutes to form an emulsion. The mixture was centrifuged at 3000 × g for 180 s. The volume of each fraction (water, oil, emulsion) was measured separately. The emulsifying capacity was expressed as mL of emulsified oil/g of sample.
Foaming capacity (FC)
The foaming capacity was determined according to the method described by Foh et al.[Citation11] with slight modifications. A weight of 3 g freeze-dried proteolysate was dissolved in 100 mL of distilled water and 30 mL of the aqueous sample dispersion was added to a 250 mL graduated cylinder and stirred thoroughly using a homogenizer (Heidolph homogenizer DIAX 900, Sigma-Aldrich Co.) at 9000 rpm for 180 s. The total dispersion volume of the protein was instantly determined after 30 s. The foaming capacity was expressed as the foam volume/g of sample.
Oil-holding capacity (OHC)
The procedure described by Shahidi, Han & Synowiecki[Citation12] was followed. Briefly, 0.5 g of freeze-dried sample was added to a 50 mL centrifuge tube and mixed with 10 mL of palm oil. The mixture was vortexed for 30 s and then centrifuged at 3000 × g for 25 min. The free oil was removed and the oil-holding capacity was measured by calculating the weight difference.
Water-holding capacity (WHC)
The procedure described by Diniz & Martin[Citation13] was followed, with minor modifications. A 0.5 g quantity of freeze-dried sample was dissolved in 10 mL of distilled water in a 50 mL centrifuge tube. The mixture was vortexed for 30 s and then centrifuged at 3000 × g for 25 min. No. 1 Whatman filter paper was used to filter the supernatant and the retrieved volume was determined. The water-holding capacity was determined by the volume difference between the initial distilled water and the retrieved water as mL of water absorbed/g of sample.
Molecular weight fractionation by ultrafiltration (UF)
The freeze-dried proteolysate was dissolved in distilled water and ultra-filtered sequentially with different molecular weight (MW) cut-offs (MWCO) using Vivaspin 15R ultrafiltration membranes (Sartorius Stedim Biotech GmbH., Germany). First, it was ultra-filtered with a 10 kDa molecular weight membrane cut-off. This process yielded two fractions: (1) retentate (F1, represented proteolysates with MW > 10 kDa) and (2) permeate (MW < 10 kDa). The permeate was ultra-filtered using a 5 kDa membrane cut-off, and the second retentate (F2, included proteolysates with MW 5–10 kDa) and permeate were obtained. The second permeate was subsequently ultra-filtered using a 2 kDa membrane cut-off yielding the third retentate (F3, included proteolysates with MW 2–5 kDa) and permeate (F4, represented proteolysates with MW < 2 kDa). All four fractions were freeze-dried and stored at −80°C until further analysis. The yield of each fraction was evaluated based on the starting volume of total proteolysate as follows:
Sodium dodecyl sulfate polyacrylamide gel electrophoresis (SDS-PAGE)
SDS-PAGE was performed on the ultrafiltrated fractions of stone fish proteolysate as described by Laemmli,[Citation14] using 15% acrylamide for protein separation. The freeze-dried fractions were dissolved in deionized water and adjusted to a 10 mg protein/mL concentration. The sample solutions and the sample buffer (0.5 M Tris-HCl, pH 6.8, containing 4% SDS, 20% glycerol) were mixed at a 1:1 (v/v) ratio and heated for 3 min at 90°C prior to loading. The concentrations of stacking and resolving gels were 4% and 15%, respectively. Gels was run at 30 mA. Aliquots of 10 μL were loaded into individual wells and a constant current was passed through the gel for 1 h to complete peptide separation. The gel was stained using Coomassie blue (0.4 g Coomassie brilliant blue R-250 in 90 mL methanol, 15 mL acetic acid and 205 mL deionized water), destained with destaining solution (200 mL of 40% methanol and 30 mL of 6% acetic acid, adjusted to 500 mL with deionized water) and preserved in 10% acetic acid and 5% glycerol. The MWs were estimated by comparison with low-range MW standards (BIO-RAD).
Reverse phase high performance liquid chromatography (RP-HPLC)
The antioxidative ultrafiltrated fraction was further fractionated based on hydrophobicity according to the method established by Zarei et al.,[Citation15] using an RP-HPLC semi-preparative system (Agilent Technologies 1200 series, Santa Clara, CA, USA) coupled with a MWD detector and fraction collector. A 0.2 mg sample of freeze-dried ultrafilterated proteolysate was diluted in 1 mL deionized water and filtered through 0.20 μM membranes (Sartorius Stedim) prior to loading onto a semi-preparative ZORBAX 300 SB-C18 column (9.4 mm × 250 mm, 5 μm particles, Agilent Technologies, USA) at a 4 mL/min flow rate. The volume of sample injection was 500 μL with 0.2 mg/mL peptide concentration. The elution was performed in the following order: 0–5 min, 100% eluent A with deionized water containing 0.1% (v/v) TFA; 5–67.5 min, 0–45% eluent B including acetonitrile containing 0.1% (v/v) TFA, at room temperature. The peptide concentration was determined out at 205 nm. Column conditioning was performed for 60 min between two consecutive runs using eluent B for 20 min followed by eluent A for 40 min. A total of 45 fractions were collected. All fractions were immediately freeze-dried and dissolved in 200 μL deionized water to measure radical scavenging activity.
Isoelectric point focusing fractionation
Fractionation of the antioxidative bioactive peptides derived from stone fish proteolysates was carried out for 48 h using an Agilent 3100 OFFGEL fractionator and OFFGEL Kit 3–10 (Agilent Technology, Waldbronn, Baden-Wuerttemberg, Germany), in which peptide separation was based on isoelectric point (pI) in an off-gel setup. Peptide separation was performed in a two-phase system as follows. The upper phase was liquid, containing focusing buffer provided by the supplier, and the lower phase was an IPG gel strip with a linear 3–10 pH gradient. A 40 μL volume of IPG Strip Rehydration Solution was pipetted into each well and incubated for 15 min to allow the IPG gel to swell (voltage was not applied). Next, 150 μL of prepared sample (diluted with OFFGEL stock solution) was loaded into each well. A voltage of 500–4000 V was applied for 48 h. Samples were focused with a maximum current of 50 μA. Peptides migrated between the wells through the IPG gel were retrieved from the IPG region where the pH was equivalent to that of the pI.
Tandem mass spectrometry analysis
The freeze-dried potent fractions were reconstituted in 25 mL of 5% acetonitrile and Milli-Q water containing 0.1% formic acid. The mixture was intermittently vortexed for 5 min and subsequently centrifuged for 10 min at 15,000 rpm. The collected supernatant was transferred into a fresh tube and analysed using ultra-high performance liquid chromatography (1290 UHPLC, Agilent Technologies, Santa Clara, USA) coupled with a high-resolution mass hybrid Q-TOF mass spectrometer (6540 Q-TOF, Agilent Technologies, Santa Clara, USA). A 1 μL volume of peptidic sample was injected into the column (ZORBAX Eclipse-Plus C-18, 2.1 mm × 50 mm, Agilent Technologies, Santa Clara) in triplicate. Separation was carried out by eluting a gradient of 5–65% of acetonitrile containing 0.1% formic acid for 15 min. Elution was carried out by feeding the eluate into the Q-TOF mass spectrometer directly through an electrospray ion source, operating in positive mode with a 4000 V capillary voltage. Data were collected in centroid mode from 100 to 3200 m/z and automatically selected for collisionally induced dissociation (CID, MS/MS) based on the charge state preference: 2 > 1 > 3, an absolute threshold of 250 counts and a peptide isotope requirement model. CID was carried out using high-purity nitrogen and a variable voltage according to the mass-to-charge ratio of the isolated peptide.
Database identification
Peptide identification was carried out using the SpectrumMill Rev.B.04.00.127 search engine (Agilent Technologies, Santa Clara) and basic parameters of 10 ppm precursor mass tolerance and 50 ppm product mass tolerance with a ‘no-enzyme’ constraint. If the peptides had a score >6 and SPI% (percentage of scored peak intensity) >50 they were considered a match.
Peptide synthesis
Peptide synthesis was carried out according to Nokihara et al.,[Citation16] using a simultaneous multiple peptide synthesizer (model PSSM-8; Shimadzu) and the fluorenyl methoxycarbonyl chemistry (Fmoc). The purification of synthesized peptides was subsequently performed using HPLC on an ODS column PEGASIL-300 (20mm × 250mm; Senshu Scientific) with a linear gradient of 0–50% CH3CN containing 0.1% TFA in 100 min (flow rate: 5.0 mL/min; monitoring: 220 nm). The molecular mass of peptides was determined using mass spectrometry. The final purity of peptides was >90%.
Statistical analysis
All statistical tests were carried out in triplicate and data were presented as the mean ± standard deviation. One-way ANOVA was used for statistical analysis and the comparison between the groups, followed by Tukey’s test for differences identification between treatments at a significance level of 5%, using Minitab version 14 software (Minitab Inc., State College, PA, USA).
Results and discussion
Profiling, DP and antioxidant activity of stone fish proteolysates
A previous study has shown that the proteolysis of stone fish (A. lecanora) by alcalase could generate potential antioxidative proteolysates.[Citation6] Park et al.,[Citation17] Yan et al.[Citation18] and Liceaga-Gesualdo and Li Chan[Citation19] have also reported the advantages of using alcalase for generation of proteolysates, as it results in shorter peptides and reduced bitterness in addition to high antioxidant activity. Hence, in the current study, alcalase was used for digesting stone fish flesh for 8 h under optimum conditions. The proteolysis profile, peptide content and DP of stone fish proteolysates, along with their antioxidant activity within 8 h of digestion, is shown in .
Table 1. Peptide content, degree of proteolysis (DP) and antioxidant activity of alcalase digested proteolysates of stone fish flesh during 8 h of proteolysis.
As shown, after an initial rapid phase of proteolysis, the proteolysis rate tended to decrease after 4 h. However by re-adding enzyme after 5 h, it again increased and maintained a slight and constant increment by 8 h. Under proteolysis conditions, the highest amount of peptide content obtained was 7.88 ± 0.32 mmol GSH/g dried sample with DP of 89%, meaning that alcalase had efficiently hydrolyzed the stone fish proteins within 8 h of proteolysis. A similar proteolysis profile has been reported for a number of other marine products such as smooth hound,[Citation20] sea urchin[Citation21] and sea cucumber S. japonicus.[Citation22] In general, the contingent differences in the pattern of proteolysis rate observed in the different protein sources could be attributed to the diverse properties of enzyme-cutting sites and the accessibility of peptide bonds to each protease. Previous studies also revealed that the DP of seafood proteolysates varied and was mainly dependent on substrate nature, enzyme activity and stability, ratio of enzyme/substrate and operating conditions.[Citation23,Citation24]
Regarding the antioxidant activity, the proteolysate exhibited significantly (P ≤ 0.05) higher FRAP value, DPPH• and ABTS• radical scavenging activities compared with the non-hydrolyzed sample (at time 0). The radical scavenging trends of both DPPH• and ABTS• showed higher rates within the first hour of proteolysis and continued to increase slightly to 8 h, although the reducing power activity increased constantly throughout the proteolysis process. It has been suggested that peptide length and solubility, composition of amino acids and their sequences, as well as the number of free amino acids within the proteolysates, play an important role in antioxidant activity. Assay conditions such as pH, as well as type of enzyme, may also contribute to the antioxidant activity.[Citation25] It has been shown that the antioxidant activity of alcalase-digested stone fish proteolysates is greater than that of other marine-derived proteolysates such as marine rotrifer (46–50%),[Citation26] sand eel (77%),[Citation27] red tilapia (IC50 of 4.45 mg/mL)[Citation28] and giant kingfish (23.6–37.0%).[Citation29]
The IC50 of the stone fish proteolysates radical scavenging activity was also determined. The IC50 value of ABTS• (0.33 mg/mL) was significantly (P ≤ 0.05) lower than DPPH• (0.5 mg/mL). Both assays showed significantly (P ≤ 0.05) higher IC50 values compared with the GSH (0.006 mg/mL). It is evident that the observed free radical scavenging activity directly resulted from the antioxidant peptides generated by proteolysis.
Degree of proteolysis versus antioxidant activity
As shown in , strong correlations were observed between FRAP value (R2 = 0.95), DPPH• and ABTS• radical scavenging activities (R2 = 0.99 and 0.98, respectively) and the degree of proteolysis. These results are in line with those of previous studies reporting a marked increase in antioxidant activity by extending the time of proteolysis.[Citation30,Citation31] In contrary, some literature has reported a decrease in antioxidant activity with prolonged proteolysis.[Citation21,Citation32–Citation34] It has been suggested that increasing the proteolysis time to 8 h leads to a decrease in peptides size and a subsequent increase in antioxidant capacity. In addition, prolonging proteolysis time may directly enhance the solvent exposure of the side-chain group residues, which likely facilitates the reactions between antioxidative peptides and free radicals, ROS and transitional metal ions resulting in the enhancement of the antioxidant capacity.[Citation24] These factors may explain the positive correlation between antioxidant activity and degree of proteolysis of the stone fish flesh. The present study also concurs with the findings by Saiga, Tanabe and Nishimura,[Citation35] who observed that extensive hydrolysis of proteins produced a higher amount of free amino acids, thus reducing the antioxidant capacity.
Physicochemical properties of stone fish proteolysates
The functional properties of alcalase-generated proteolysate after 8 h of proteolysis was evaluated for emulsifying capacity (EC), foaming capacity (FC), oil-holding capacity (OHC) and water-holding capacity (WHC), as these parameters can influence the applicability in food systems (). The emulsifying activity of a protein is its ability to forming and stabilize an emulsion system by giving units of interface area which are stabilized per unit weight of protein.[Citation19] Our results showed that the EC value (8.5 ± 0.5 mL/g) was substantially lower than in previous reports of other marine proteolysates. The low value of EC might be attributed to the inability of the peptides in the proteolysates to lower the interfacial tension at the interface of oil and water due to low hydrophobicity and MW. According to Li-Chan and Nakai,[Citation40] the ability of a protein to interact with oil and/or other molecules of protein, thus forming an interfacial layer, is particularly related to the hydrophobic interactions. Consequently, low MW peptides are insufficiently amphiphilic to exhibit good emulsifying properties. Although small peptides are able to diffuse faster and absorb at the interface, they cannot unfold and reorient themselves at the surface.[Citation41]
Table 2. Physicochemical properties of stone fish proteolysates in comparison to other marine-based proteolysates.
Proteins in dispersion systems that are capable of lowering the surface tension at the water–air interface have flexible structures and are thus capable of creating foam.[Citation42] Such characteristics are required in products for baking, traditional food preparation and dessert formulations.[Citation39] The FC of stone fish proteolysate was found to be 2.33 ± 0.58 mL/g. The relatively low foaming capacity of the stone fish proteolysates could be the result of extensive proteolysis, hence yielding predominantly small peptides. This could lead to a stable film being formed around the gas bubbles due to the development of the hydrophilic peptides. In addition, low-MW peptides might not be capable of preserving the well-ordered alignment of molecules at the interface.[Citation10] This is supported by previous studies reporting that films with good stability are typically obtained by peptides or proteolysates with high molecular mass.[Citation10,Citation43] Researchers have suggested that the type of enzyme used also influences foaming properties.[Citation44]
The oil-holding capacity (OHC) of proteolysates is an important property that not only affects the taste of food products, but is also essential for use as substitutes or extenders in some products such as cakes, sausages, mayonnaises, and other salad dressings, where oil absorbance is required. High oil-holding capacity prevents phase separation and improves taste retention and palatability of the products.[Citation45] The OHC of the proteolysate was found to be 2.17 ± 0.29 mL/g, which is comparable with the grass carp skin hydrolysate (2.4 mL/g)[Citation36] and the Nile tilapia proteolysates (2.23 mL/g),[Citation11] but higher than shark muscle (0.3–0.5 mL/g),[Citation13] which might be due to the presence of higher amount of hydrophobic peptides in stone fish proteolysate compared with the shark muscle hydrolysate. Nevertheless, the OHC value remains far from the optimum point required in food manufacturing. The low value of OHC could be attributed to the decrease of the hydrophobic interactions as the result of extensive proteolysis, which led to the hydrolytic degradation of protein structures. Our results were in line with those of Wasswa et al. (2007),[Citation36] who reported that the OHC of grass carp skin hydrolysate decreased as DH increased. Kristinsson and Rasco (2000)[Citation46] also showed a decrease in OHC of salmon hydrolysates due to increasing DH. In contrast, Diniz and Martin (1997)[Citation13] reported that DH had no effect on the OHC of shark muscle hydrolysate.
The water-holding capacity of proteins and proteolysates is of significance in the food industry, for example in the meat product manufacturing industry, as the water retaining in food systems typically improves texture. The WHC of alcalase-digested stone fish antioxidative proteolysate was found to be 4.5 ± 0.5 mL/g. Peptides of lower MW appear to be more effective in holding water, since smaller fragments of peptides are more hydrophilic. However, extensive hydrolysis leads to the production of extra-small peptides and decreases the WHC due to the enhancement of solubility. Furthermore, peptide composition in each of the fractions may play a key role in WHC.[Citation47]
Fractionation of stone fish alcalase-generated proteolysate based on molecular weight using ultrafiltration
The UF technique is typically used in industrial and laboratory profiles to fractionate, purify and concentrate proteins and peptides. In the present study, stone fish proteolysate was fractionated using MWCO membrane ultrafiltration into four molecular sizes of < 2, 2–5, 5–10, and <10 kDa. The yield values of each molecular size fraction prepared from UF are presented in . The results clearly reveal that the <2 kDa fractions were the most abundant after membrane ultrafiltration, indicating that the protease was efficient at reducing stone fish proteins into low molecular weight peptides. These results were in agreement with the findings of He et al.[Citation48] and Fan et al.,[Citation37] which indicated that the <1 kDa fraction from rapeseed[Citation48] and tilapia[Citation38] protein hydrolysates had the highest yields after membrane ultrafiltration. Zhou et al.[Citation24] also reported the highest contents of UF fractions from abalone protein hydrolysates belonging to fractions with MWs below 3 kDa.
Table 3. The yield and DPPH• radical scavenging activity of stone fish proteolysate ultrafiltration fractions.
The UF fractions were further assayed for DPPH• by radical scavenging assay. As indicated in , the <2 kDa and 2–5 kDa peptides exhibited higher DPPH• radical scavenging activities compared with fractions of higher MW peptides. However, the radical scavenging activities of fractions <2 kDa and 2–5 kDa showed no significant difference (P > 0.05). The value of IC50 for the <2 kDa fraction was 0.33 mg/mL, which is significantly higher (P ≤ 0.05) than the GSH (IC50 0.027 mg/mL), but lower than those reported for alfalfa leaf,[Citation49] rapeseed[Citation50] and wheat gluten proteolysates.[Citation51]
The DPPH• radical scavenging activity of the UF fractions showed an inverse relation with molecular size; the lower the molecular size of peptides, the higher the DPPH• radical scavenging activity. This again reaffirms that short-chain peptides have higher antioxidant capacity than long-chain peptides. In contrast, the antioxidant activity of the protamex-digested hydrolysate and supernatant fractions of catfish was reported to decrease with an increase in degree of hydrolysis and decreasing peptide size and molecular weight.[Citation32] Our findings were in agreement with those reported previously by a number of researchers,[Citation24,Citation48,Citation52] who showed that low MW peptide fractions had higher DPPH• scavenging activities.
Molecular weight distribution profiling
The molecular size distribution profiles of stone fish alcalase-digested unfractionated proteolysates, along with the ultrafiltrated fractions, were determined using SDS gel electrophoresis. Standard MW markers were applied to indicate the presence of peptides of various MWs. As shown in , alcalase had high efficiency in degrading stone fish proteins, cleaving almost all proteins with high MW and generating polypeptides with MW of less than 45 kDa.
Figure 2. SDS-PAGE patterns of unfractionated stone fish selected proteolysate and its ultrafiltrated fractions. MW: molecular weight, N.F: unfractionated stone fish proteolysate. >10: fraction with MW > 10 KDa, 5–10: fraction with MW between 5 and 10 KDa, 2–5: fraction with MW between 2 and 5 KDa <2: fraction with MW < 2 KDa.
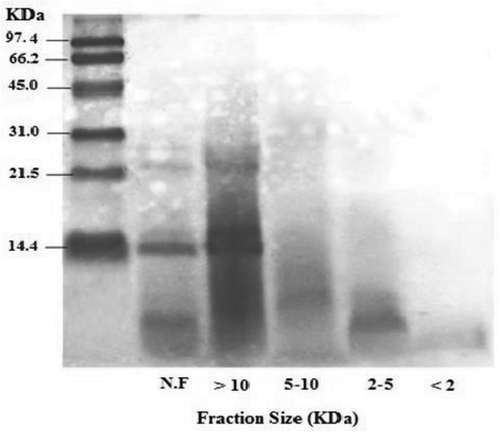
This finding supports previous research on alcalase proteolysate of S. horrens flesh, which was reported to generate polypeptides >20 kDa.[Citation53] However, this is in contrast with studies on other marine substrates with different nature of proteins like fish muscle, showing two major bands with MW of 212 and 166 kDa.[Citation28] A decrease in MW of a peptide occur due to the digestion of flesh proteins, leading to the breaking of myofibrillar proteins and long chain polypeptides into small-chain peptides. As reported previously,[Citation53,Citation54] collagen is the main protein component of sea cucumber flesh, consisting of an α long chain of amino acids with MW of 100 kDa. The absence of high MW bands above 45 kDa indicates that alcalase is capable of degrading collagen to smaller molecules with lower MW. The generation of lower MW peptides by alcalase indicates that stone fish protein could possess the majority of cutting sites compatible with alcalase. Our results are again in line with the findings of Forghani et al.,[Citation53] who showed high efficiency of alcalase for S. horrens protein cleavage in producing small peptides. Stone fish unfractionated proteolysates, as well as the >10 kDa fractions, showed two thick bands with MWs of 29 and 21 kDa. For the fractions <10 kDa, however, no significant band was observed and the peptide bands appeared as smears. As the smears of 10–5 kDa fractions were below 21 kDa, and fractions 5–2 and <2 kDa were all <6.9 kDa, it was concluded that the UF method had been successfully implemented.
Fractionation and characterization based on hydrophobicity using RP-HPLC
RP-HPLC is established as a powerful technique for the analysis of peptides and proteins. This method separates molecules based on hydrophobicity. A typical RP-HPLC system used for protein and peptide analysis consists of a sorbent with an n-alkylsilica base, with the elution of solutes carried out by incremental concentrations of organic solvent containing an ionic modifier (for example, acetonitrile containing trifluoroacetic acid) gradient. Intricate combination of peptides can be regularly separated, and quantities of low picomolar-femtomolar materials can be obtained for further characterization.[Citation55]
In the present study, the ultra-filtrated fraction <2 kDa, which showed the most potent antioxidant activity, was lyophilized and subjected to RP-HPLC fractionation with a linear acetonitrile gradient of 0–45% containing 0.1% TFA. A total of 45 fractions were collected from each run of RP-HPLC fractionation. All fractions were freeze-dried individually and dissolved in 250 μL deionized water prior to the measurement of antioxidant activity.
Fractions 2 and 3 (>99% hydrophilic), 19 (8% hydrophobic), 20 (9% hydrophobic) and 31 (17% hydrophobic) showed the highest DPPH• radical scavenging activity (68.55, 70.84, 62.60, 61.68, and 61.83%, respectively), and had been diluted up to 100-fold from the initial proteolysates. Our results showed higher DPPH• radical scavenging activity compared with walnut proteolysate RP-HPLC fractions with 40% DPPH• radical scavenging activity at a hydrophobicity of 21%[Citation34] and alfalfa leaf peptide fractions with DPPH• radical scavenging activity of 79.7% at a 1.6 mg/mL concentration.[Citation49] However, RP-HPLC peptide fractions of ostrich egg showed higher DPPH• radical scavenging activity (81%) at 200 μg/mL.[Citation31] The results for the RP-HPLC fractions 2 and 3 with <5% hydrophobicity were in agreement with those obtained previously from PRA2 fractions (with 6% hydrophobicity) of hydrolysate derived from tuna dark muscle by-product, which showed the highest DPPH• radical scavenging capacity (62.5%) at 100 μg/mL.[Citation33]
The correlation between the hydrophobicity and antioxidant activity of fractions was also determined (). A meaningful relation between hydrophobicity of fractions and their antioxidant activity was observed, where most fractions with strong antioxidant capacity were more hydrophilic. This could be attributable to the presence of hydrophilic amino acids within the antioxidative bioactive peptide sequences. The findings of the present study demonstrated that the antioxidant property of stone fish proteolysate was not only related to the size, MW and degree of proteolysis, but also to the hydrophobicity of generated bioactive peptides.
Fractionation based on isoelectric point (PI) using an OFFGEL fractionator
A total of five peptide fractions with the highest antioxidant activities obtained from RP-HPLC [fractions number 2 (F2), 3 (F3), 19 (F19), 20 (F20), and 31 (F31)] were selected for characterization based on pI using an Agilent 3100 OFFGEL fractionator coupled with a 12-well setup and an IPG strip at pH 3–10. Peptide separation was carried out in a two-phase system, including a liquid upper phase which was distributed into the wells and a conventional rehydrated IPG gel strip as the lower phase. Following the IEF process, peptides were collected from the liquid phase and readily retrieved. A total of 60 fractions were obtained, each of which was further evaluated for antioxidant activity using a DPPH• free radical scavenging assay ().
Figure 4. DPPH• radical scavenging activity of OFFGEL fractions obtained from alcalase proteolysate. The subscriptions a-h indicated the significant differences at P ≤ 0.05, (mean ± SD, n = 3). A: al-2, B: al-3, C: al-19, D: al-20 and E: al-31. F.N: Fraction Number, AA%: antioxidant activity %.
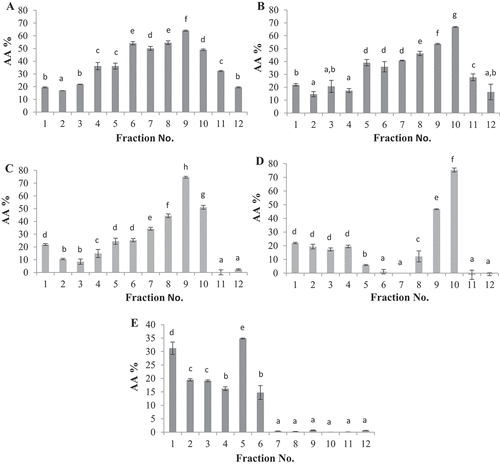
The highest DPPH• radical scavenging activities were obtained from fraction 9 of al-19 (74.7 ± 1,4) with pI = 9 and fraction 10 of al-20 (75.3 ± 2.9%) with pI = 9.7. This was in contrast with previous reports by Zarei et al.[Citation15] and Park et al.,[Citation56] which showed that the highest antioxidant activity was obtained from fractions with pI = 5–7[Citation15] and pI < 6. However, fraction 7 of F31 with pI 6.7 also showed strong antioxidant activity (74.1 ± 3.4%), comparable with fractions F18 and F 24, and in the optimum pI range reported by Zarei et al.[Citation15] for palm kernel cake OFFGEL fractions.
Purification of selected fraction (al-20–10) using RP-HPLC
Fraction 10 of al-20, which showed the highest antioxidant activity, was selected for purification with RP-HPLC. The peptide fraction chromatogram is shown in . Given that the OFFGEL buffer was a fully hydrophilic solution, it separated within the first 5 min of the RP-HPLC separation process. As shown, fractions displayed the peptide area at approximately the same time as the previous RP-HPLC fractionation. This indicates that peptides are separated at approximately the same time as they were collected in the previous step of RP-HPLC, indicating a lower possibility of error.
The final purified fractions were collected, lyophilized, and assayed for DPPH• radical scavenging activity. The final peptide fractions showed 67% DPPH• radical scavenging activity. This reduction in activity may have been caused by less reduction in peptide concentration within the fractionation processes. However, our results remain comparable with those reported by Hsu[Citation33] and Chen et al.[Citation34] Our findings indicate that bioactive peptides derived from stone fish flesh proteolysates may represent an appreciable source of natural antioxidants, and therefore required further identification and characterization by MS/MS.
Peptide identification
To identify the most potent peptides, the collected fraction was subsequently subjected to LC–MS/MS for peptide sequence identification and characterization. The mass spectra for fractions are displayed in , where the identified peptides are numbered. Four peptides were identified including Peptide 1 (GVSGLHID), Peptide 2 (PTPSRPQQGPA), Peptide 3 (PGRRAA), and Peptide 4 (LAQVAVSNPNN). All four peptide fractions were further synthesized and tested for antioxidant activity using ABTS• and DPPH• radical scavenging assays.
Figure 6. MS/MS chromatogram, a: peptide 1 (GVSGLHID), score: 76.1, ion intensity: 4.19E+ 05, RT (min): 3.42, precursor m/z: 399.2115, matched m/z: 797.416. b: peptide 2 (PTPSRPQQGPA), score: 58.6, ion intensity: 1.75E+ 06, RT (min): 2.8, precursor m/z: 568.2972, matched m/z: 1135.586. c: peptide 3 (PGRRAA), score: 64.2, ion intensity: 2.59E+ 05, RT (min): 2.35, precursor m/z: 314.1826, matched m/z: 627.369. d: peptide 4 (LAQVAVSNPNN), score: 56.4, ion intensity: 2.03E+ 05, RT (mins): 2.37, precursor m/z: 376.1959, matched m/z: 1126.586.
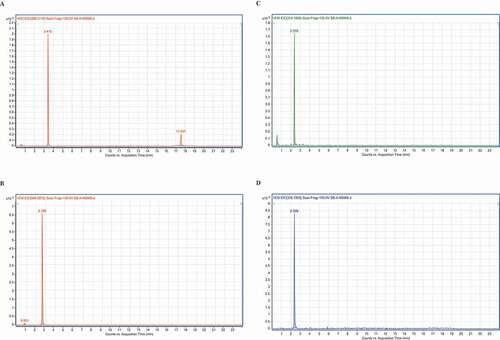
As shown in , Peptide 1 (GVSGLHID) presented the strongest antioxidant activity with an ABTS• value of 85% at 5 mg/mL with IC50 of 3.28 ± 0.15 mg/mL and DPPH• radical scavenging activity of 65% at 5 mg/mL with IC50 of 4.14 ± 0.21 mg/mL. Peptide 1 showed higher antioxidant activity than peptide H3 derived from Arca subcrenata with 56.8% DPPH• scavenging activity at 8 mg/mL.[Citation34] The next most potent antioxidative peptide was Peptide 3 (PGRRAA), followed by Peptide 2 (PTPSRPQQGPA), and the results were similar to those in previous reports on antioxidant peptides isolated from fish by-products with EC50 values from 90 to 670 mM,[Citation57,Citation58] peptide derived from horse mackerel viscera proteolysate with DPPH• radical scavenging activity of 59.1%,[Citation30] and the antioxidative peptides derived from sardinelle with the DPPH• radical scavenging activity from 38 ± 1.3% to 63 ± 1.5%,[Citation20] but were lower than Apostichopus japonicas egg proteolysates with hydroxyl radical scavenging activity of 89.82 U/mL.[Citation59]
Table 4. Stone fish-derived peptides characteristics and antioxidant activities.
Peptide 4 (LAQVAVSNPNN) showed negative ABTS• (−19% at 5 mg/mL) and DPPH• (−34% at 5 mg/mL) radical scavenging values, suggesting that the peptide has pro-oxidant activity. A similar argument was proposed by Shen et al. (2010)[Citation60] for two peptides (LSKAQSDFG) and (LVEKGDVAFI) originating from ovotransferrin, which were shown to have negative antioxidant activity. Some peptide fractions have also been reported as pro-oxidative in previous studies.[Citation60,Citation61] A number of amino acids are known antioxidants. The amino acids Tyr, Trp and Met are reported to show the greatest antioxidant activity, followed by His, Cys, and Phe.[Citation27] However, these amino acids can occasionally exert pro-oxidative effects as well, potentially due to their order within the peptide sequence.
The high potential of Peptide 1 in exerting antioxidant activity could be attributable to the presence of Asp at the C-terminal. Asp is an acidic amino acid which plays a key role in metal ion chelation and antioxidant activity.[Citation17,Citation50] The presence of Val, Leu and Ile in the sequence of Peptide 1 could be another reason for its antioxidant activity.[Citation31,Citation51] Moreover, the presence of His, which is a direct proton-donor, and its ability to quench unpaired electrons or radicals, could be critical to the radical-scavenging activity of Peptide 1.[Citation27] Our results also verified the established belief that short-chain peptides with fewer than 10 amino acids exhibit higher antioxidant capacity than long-chain peptides.
The properties of bioactive peptides are attributed to their structure, amino acid composition and sequence.[Citation27] In terms of antioxidant activity, it has been reported that the placement of amino acids such as Val, Ile, Leu, Ala, Phe, and Lys at the C-terminal results in higher efficacy than when they are located at the N-terminal region.[Citation31,Citation51] Furthermore, an abundance of hydrophobic amino acids (Met, Val, Leu, and Pro) within the peptide sequence has been shown to contribute to the enhancement of the antioxidant activity of hydrophobic peptides by increasing the solubility of the peptides in lipids as well as their interactions with free radicals.[Citation27] His is a polar amino acid whose presence in the peptide sequence is reported to cause strong antioxidant activity due to its attribution in the proton-donating ability of its imidazole group,[Citation20] along with the chelating and radical-trapping effects of its imidazole ring in lipid.[Citation17] However, a higher concentration of hydrophobic amino acids at the C-terminal has been reported to contribute to the increment of peptides antioxidant capacity – at the C-terminal of Peptide 1, the total number of hydrophilic amino acids, including His, Ile and Asp, is noticeably higher. Therefore, this line of reasoning could not be explained by the mechanism responsible for the higher antioxidant capacity of Peptide 1.
Conclusions
The present study revealed that alcalase digestion of stone fish flesh proteins for 8 h can produce appreciable quantities of antioxidative proteolysates. However, the excessive proteolysis time (8 h) leading to the enhancement of antioxidant activity also caused a decrease in the physicochemical properties of proteolysates due to a reduction in peptide size. Several fractionation steps were used to separate, purify and characterize the antioxidative peptides from stone fish alcalase proteolysate by UF (based on MW), RP-HPLC (based on hydrophobicity) and isoelectric focusing fractionation (based on isoelectric point). A negative correlation was observed between antioxidant activity and MW as well as hydrophobicity. In contrast, the net charge and isoelectric point of peptides showed a positive correlation with antioxidant activity. The final purified fraction with the highest antioxidant activity was selected for peptide sequencing using MS/MS. A total of four peptides were identified, including Peptide 1 with the sequence of GVSGLHID, which showed the highest antioxidant activity with an ABTS• value of 85% at 5 mg/mL (IC50 of 3.28 ± 0.15 mg/mL) and DPPH• radical scavenging activity of 65% at 5 mg/mL (IC50 of 4.14 ± 0.21 mg/mL). These results demonstrate that stone fish flesh represents a promising economical source of antioxidative proteolysates and peptides, with significant potential to enhance the nutritional value and health benefits, as well as the shelf-life, of nutraceutical and functional food products.
Additional information
Funding
References
- Zarei, M.; Ebrahimpour, A.; Abdul Hamid, A.; Anwar, F.; Bakar, F.; Philip, R.; Saari, N. Identification and Characterization of Papain-Generated Antioxidant Peptides from Palm Kernel Cake Proteins. Food Research International 2014, 62, 726–734. DOI: 10.1016/j.foodres.2014.04.041.
- Bordbar, S.; Anwar, F.; Saari, N. High-Value Components and Bioactives from Sea Cucumbers for Functional Foods, A Review. Marine Drugs 2011, 9, 1761–1805. DOI: 10.3390/md9101761.
- Bordbar, S.; Ebrahimpour, A.; Abdul Hamid, A.; Abdul Manap, M.-Y.; Anwar, F.; Saari, N. The Improvement of the Endogenous Antioxidant Property of Stone Fish (Actinopyga Lecanora) Tissue Using Enzymatic Proteolysis. BioMed Research International 2013, 2013, 1–9. DOI: 10.1155/2013/849529.
- Ghanbari, R.; Ebrahimpour, A.; Zarei, M.; Ismail, A.; Abdul-Hamid, A.; Saari, N. Purification and Characterization of Nitric Oxide Inhibitory Peptides from Actinopyga Lecanora through Enzymatic Hydrolysis. Food Biotechnology 2016, 30(4), 263–277. DOI: 10.1080/08905436.2016.1234391.
- Ghanbari, R.; Ebrahimpour, A.; Abdul-Hamid, A.; Ismail, A.; Saari, N. Actinopyga Lecanora Hydrolysates as Natural Antibacterial Agents. International Journal of Molecular Sciences 2012, 13(12), 16796–16811. DOI: 10.3390/ijms131216796.
- Church, F.-C.; Swaisgood, H.-E.; Porter, D.-H.; Catignani, G.-L. Spectrophotometric Assay Using o-Phthaldialdehyde for Determination of Proteolysis in Milk and Isolated Milk Proteins. Journal of Dairy Science 1983, 66, 1219–1227. DOI: 10.3168/jds.S0022-0302(83)81926-2.
- Bersuder, P.; Hole, M.; Smith, G. Antioxidants from a Heated Histidine-Glucose Model System. I: Investigation of the Antioxidant Role of Histidine and Isolation of Antioxidants by High-Performance Liquid Chromatography. Journal of the American Oil Chemists’ Society 1998, 75, 181–187. DOI: 10.1007/s11746-998-0030-y.
- Re, R.; Pellegrini, N.; Proteggente, A.; Pannala, A.; Yang, M.; Rice-Evans, C. Antioxidant Activity Applying an Improved ABTS Radical Cation Decolorization Assay. Free Radical Biology and Medicine 1999, 26, 1231–1237. DOI: 10.1016/S0891-5849(98)00315-3.
- Guo, C.; Yang, J.; Wei, J.; Li, Y.; Xu, J.; Jiang, Y. Antioxidant Activities of Peel, Pulp and Seed Fractions of Common Fruits as Determined by FRAP Assay. Nutrition Research 2003, 23, 1719–1726. DOI: 10.1016/j.nutres.2003.08.005.
- Souissi, N.; Bougatef, A.; Triki-Ellouz, Y.; Nasri, M. Biochemical and Functional Properties of Sardinella (Sardinella Aurita) By-Product Hydrolysates. Food technology and biotechnology 2007, 45, 187.
- Foh, M.-B.-K.; Amadou, I.; Kamara, M.-T.; Foh, B.-M.; Xia, W. Effect of Enzymatic Hydrolysis on the Nutritional and Functional Properties of Nile Tilapia (Oreochromis Niloticus) Proteins. American Journal of Biochemistry and Molecular Biology 2011, 1, 54–67. DOI: 10.3923/ajbmb.2011.54.67.
- Shahidi, F.; Han, X.-Q.; Synowiecki, J. Production and Characteristics of Protein Hydrolysates from Capelin (Mallotus Villosus). Food Chemistry 1995, 53, 285–293. DOI: 10.1016/0308-8146(95)93934-J.
- Diniz, F.; Martin, A. Effects of the Extent of Enzymatic Hydrolysis on Functional Properties of Shark Protein Hydrolysate. LWT - Food Science and Technology 1997, 30, 266–272. DOI: 10.1006/fstl.1996.0184.
- Laemmli, U.-K.;. Cleavage of Structural Proteins during the Assembly of the Head of Bacteriophage T4. Nature 1970, 227, 680–685.
- Zarei, M.; Ebrahimpour, A.; Abdul Hamid, A.; Anwar, F.; Saari, N. Production of Defatted Palm Kernel Cake Protein Hydrolysate as a Valuable Source of Natural Antioxidants. International Journal Molecular Sciences 2012, 13, 8097–8111. DOI: 10.3390/ijms13078097.
- Nokihara, K.; Yamamoto, R.; Hazama, M.; Wakizawa, O.; Nakamura, S. Design and Applications of a Novel Simultaneous Multi Solid-Phase Peptide Synthesizer. In Innovation and Perspectives in Solid-Phase Synthesis. Innovation and Perspectives in Solid-Phase Synthesis; Epton, R.; ed., Andover, UK: Intercept Limited, 1992; 445.
- Park, P.-J.; Jung, W.-K.; Nam, K.-S.; Shahidi, F.; Kim, S.-K. Purification and Characterization of Antioxidative Peptides from Protein Hydrolysate of Lecithin-Free Egg Yolk. Journal of the American Oil Chemists’ Society 2001, 78, 651–656. DOI: 10.1007/s11746-001-0321-0.
- Yan, M.; Tao, H.; Qin, S. Effect of Enzyme Type on the Antioxidant Activities and Functional Properties of Enzymatic Hydrolysates from Sea Cucumber (Cucumaria Frondosa) Viscera. Journal of Aquatic Food Product Technology 2016, 25, 940–952. DOI: 10.1080/10498850.2014.994083.
- Liceaga-Gesualdo, A.; Li-Chan, E. Functional Properties of Fish Protein Hydrolysate from Herring (Clupea Harengus). Journal of Food Science 1999, 64, 1000–1004. DOI: 10.1111/jfds.1999.64.issue-6.
- Bougatef, A.; Hajji, M.; Balti, R.; Lassoued, I.; Triki-Ellouz, Y.; Nasri, M. Antioxidant and Free Radical-Scavenging Activities of Smooth Hound (Mustelus Mustelus) Muscle Protein Hydrolysates Obtained by Gastrointestinal Proteases. Food Chemistry 2009, 114, 1198–1205. DOI: 10.1016/j.foodchem.2008.10.075.
- Qin, L.; Zhu, B.-W.; Zhou, D.-Y.; Wu, H.-T.; Tan, H.; Yang, J.-F.; Murata, Y. Preparation and Antioxidant Activity of Enzymatic Hydrolysates from Purple Sea Urchin (Strongylocentrotus Nudus) Gonad. LWT - Food Science and Technology 2011, 44, 1113–1118. DOI: 10.1016/j.lwt.2010.10.013.
- Pan, S.-K.; Wu, S. Hydroxyl Radical Scavenging Activity of Peptide from Sea Cucumber Using Enzyme Complex Isolated from the Digestive Tract of Sea Cucumber. African Journal of biotechnology 2012, 11, 1214–1219.
- Harnedy, P.-A.; FitzGerald, R.-J. Bioactive Peptides from Marine Processing Waste and Shellfish: A Review. Journal of Functional Foods 2012, 4, 6–24. DOI: 10.1016/j.jff.2011.09.001.
- Zhou, D.-Y.; Tang, Y.; Zhu, B.-W.; Qin, L.; Li, D.-M.; Yang, J.-F.; Murata, Y. Antioxidant Activity of Hydrolysates Obtained from Scallop (Patinopecten Yessoensis) and Abalone (Haliotis Discus Hannai Ino) Muscle. Food Chemistry 2012, 132, 815–822. DOI: 10.1016/j.foodchem.2011.11.041.
- Ryan, J.-T.; Ross, R.-P.; Bolton, D.; Fitzgerald, G.-F.; Stanton, C. Bioactive Peptides from Muscle Sources: Meat and Fish. Nutrients 2011, 3, 765–791. DOI: 10.3390/nu3090765.
- Byun, H.-G.; Lee, J.-K.; Park, H.-G.; Jeon, J.-K.; Kim, S.-K. Antioxidant Peptides Isolated from the Marine Rotifer, Brachionus Rotundiformis. Process Biochemistry 2009, 44, 842–846. DOI: 10.1016/j.procbio.2009.04.003.
- Lee, W.-S.; Jeon, J.-K.; Byun, H.-G. Characterization of a Novel Antioxidative Peptide from the Sand Eel Hypoptychus Dybowskii. Process Biochemistry 2011, 46, 1207–1211. DOI: 10.1016/j.procbio.2011.02.001.
- Shamloo, M.; Bakar, J.; Mat-Hashim, D.; Khatib, A. Biochemical Properties of Red Tilapia (Oreochromis Niloticus) Protein Hydrolysates. International food research journal 2012, 19, 183–188.
- Nazeer, R.; Kulandai, K.-A. Evaluation of Antioxidant Activity of Muscle and Skin Protein Hydrolysates from Giant Kingfish, Caranx Ignobilis (Forsskål, 1775). International Journal of Food Science & Technology 2012, 47, 274–281. DOI: 10.1111/ifs.2012.47.issue-2.
- Kumar, N.-S.; Nazeer, R.; Jaiganesh, R. Purification and Identification of Antioxidant Peptides from the Skin Protein Hydrolysate of Two Marine Fishes, Horse Mackerel (Magalaspis Cordyla) and Croaker (Otolithes Ruber). Amino Acids 2012, 42, 1641–1649. DOI: 10.1007/s00726-011-0858-6.
- Tanzadehpanah, H.; Asoodeh, A.; Chamani, J. An Antioxidant Peptide Derived from Ostrich (Struthio Camelus) Egg White Protein Hydrolysates. Food Research International 2012, 49, 105–111. DOI: 10.1016/j.foodres.2012.08.022.
- Theodore, A.-E.; Raghavan, S.; Kristinsson, H.-G. Antioxidative Activity of Protein Hydrolysates Prepared from Alkaline-Aided Channel Catfish Protein Isolates. Journal of Agricultural and Food Chemistry 2008, 56, 7459–7466. DOI: 10.1021/jf800185f.
- Hsu, K.-C.;. Purification of Antioxidative Peptides Prepared from Enzymatic Hydrolysates of Tuna Dark Muscle By-Product. Food Chemistry 2010, 122, 42–48. DOI: 10.1016/j.foodchem.2010.02.013.
- Chen, N.; Yang, H.; Sun, Y.; Niu, J.; Liu, S. Purification and Identification of Antioxidant Peptides from Walnut (Juglans Regia L.). Protein Hydrolysates. Peptides 2012, 38, 344–349. DOI: 10.1016/j.peptides.2012.09.017.
- Saiga, A.; Tanabe, S.; Nishimura, T. Antioxidant Activity of Peptides Obtained from Porcine Myofibrillar Proteins by Protease Treatment. Journal of Agricultural and Food Chemistry 2003, 51, 3661–3667. DOI: 10.1021/jf021156g.
- Wasswa, J.; Tang, J.; Gu, X.-H.; Yuan, X.-Q. Influence of the Extent of Enzymatic Hydrolysis on the Functional Properties of Protein Hydrolysate from Grass Carp (Ctenopharyngodon Idella) Skin. Food Chemistry 2007, 104, 698–704. DOI: 10.1016/j.foodchem.2007.03.044.
- Dos Santos, S.-D.-A.; Martins, V. G.; Salas-Mellado, M.; Prentice, C. Evaluation of Functional Properties in Protein Hydrolysates from Bluewing Searobin (Prionotus Punctatus) Obtained with Different Microbial Enzymes. Food and Bioprocess Technology 2011, 4, 1399–1406. DOI: 10.1007/s11947-009-0301-0.
- Fan, J.; He, J.; Zhuang, Y.; Sun, L. Purification and Identification of Antioxidant Peptides from Enzymatic Hydrolysates of Tilapia (Oreochromis Niloticus) Frame Protein. Molecules 2012, 17, 12836–12850. DOI: 10.3390/molecules171112836.
- Galla, N.-R.; Pamidighantam, P.-R.; Akula, S.; Karakala, B. Functional Properties and in Vitroantioxidant Activity of Roe Protein Hydrolysates of Channa Striatus and Labeo Rohita. Food Chemistry 2012, 132, 1171–1176. DOI: 10.1016/j.foodchem.2011.11.055.
- Li-Chan, E.; Nakai, S. Importance of Hydrophobicity of Proteins in Food Emulsions. American Chemical Social 1991, 15, 193–212.
- Chobert, J.-M.; Bertrand-Harb, C.; Nicolas, M.-G. Solubility and Emulsifying Properties of Caseins and Whey Proteins Modified Enzymatically by Trypsin. Journal of Agricultural and Food Chemistry 1988, 36, 883–892. DOI: 10.1021/jf00083a002.
- Hmidet, N.; Balti, R.; Nasri, R.; Sila, A.; Bougatef, A.; Nasri, M. Improvement of Functional Properties and Antioxidant Activities of Cuttlefish (Sepia Officinalis) Muscle Proteins Hydrolyzed by Bacillus Mojavensis A21 Proteases. Food Research International 2011, 44, 2703–2711. DOI: 10.1016/j.foodres.2011.05.023.
- Nalinanon, S.; Benjakul, S.; Visessanguan, W.; Kishimura, H. Improvement of Gelatine Extraction from Bigeye Snapper Skin Using Pepsin-Aided Process in Combination with Protease Inhibitor. Food Hydrocolloids 2008, 22, 615–622. DOI: 10.1016/j.foodhyd.2007.01.012.
- Elavarasan, K.; Kumar, N.-V.; Shamasundar, B. Antioxidant and Functional Properties of Fish Protein Hydrolysates from Fresh Water Carp (Catla Catla) as Influenced by the Nature of Enzyme. Journal of Food Processing and Preservation 2013, 38, 1207–1214. DOI: 10.1111/jfpp.12081.
- Rodríguez-Ambriz, S.-L.; Martinez-Ayala, A.-L.; Millan, F.; Davila-Ortiz, G. Composition and Functional Properties of Lupinus Campestris Protein Isolates. Plant Foods for Human Nutrition. 2005, 60, 99–107. DOI: 10.1007/s11130-005-6835-z.
- Kristinsson, H.-G.; Rasco, B.-A. Biochemical and Functional Properties of Atlantic Salmon (Salmo Salar) Muscle Proteins Hydrolyzed with Various Alkaline Proteases. Journal of Agricultural and Food Chemistry 2000, 48, 657–666.
- Cumby, N.; Zhong, Y.; Naczk, M.; Shahidi, F. Antioxidant Activity and Water-Holding Capacity of Canola Protein Hydrolysates. Food Chemistry 2008, 109, 144–148. DOI: 10.1016/j.foodchem.2007.12.039.
- He, R.; Girgih, A.-T.; Malomo, S.-A.; Ju, X.; Aluko, R.-E. Antioxidant Activities of Enzymatic Rapeseed Protein Hydrolysates and the Membrane Ultrafiltration Fractions. Journal of Functional Foods 2012, 5, 219–227. DOI: 10.1016/j.jff.2012.10.008.
- Xie, Z.; Huang, J.; Xu, X.; Jin, Z. Antioxidant Activity of Peptides Isolated from Alfalfa Leaf Protein Hydrolysate. Food Chemistry 2008, 111, 370–376. DOI: 10.1016/j.foodchem.2008.03.078.
- Pan, M.; Jiang, T.-S.; Pan, J.-L. Antioxidant Activities of Rapeseed Protein Hydrolysates. Food and Bioprocess Technology 2011, 4, 1144–1152. DOI: 10.1007/s11947-009-0206-y.
- Kong, X.; Zhou, H.; Hua, Y. Preparation and Antioxidant Activity of Wheat Gluten Hydrolysates (Wghs) Using Ultrafiltration Membranes. Journal of the Science of Food and Agriculture 2008, 88, 920–926. DOI: 10.1002/(ISSN)1097-0010.
- Park, S.-Y.; Je, J.-Y.; Ahn, C.-B. Protein Hydrolysates and Ultrafiltration Fractions Obtained from Krill (Euphausia Superba): Nutritional, Functional, Antioxidant, and ACE-Inhibitory Characterization. Journal of Aquatic Food Product Technology 2016, 25, 1266–1277. DOI: 10.1080/10498850.2015.1054539.
- Forghani, B.; Ebrahimpour, A.; Bakar, J.; Abdul Hamid, A.; Hassan, Z.; Saari, N. Enzyme Hydrolysates from Stichopus Horrens as a New Source for Angiotensin-Converting Enzyme Inhibitory Peptides. Evidence-Based Complementary and Alternative Medicine 2012, 2012, 1–9. DOI: 10.1155/2012/236384.
- Saito, K.; Jin, D.-H.; Ogawa, T.; Muramoto, K.; Hatakeyama, E.; Yasuhara, T.; Nokihara, K. Antioxidative Properties of Tripeptide Libraries Prepared by the Combinatorial Chemistry. Journal of food science 2002, 67, 1319–1322. DOI: 10.1111/j.1365-2621.2002.tb10281.x.
- Aguilar, M.;. HPLC of Peptides and Proteins: Methods and Protocols. Humana Press: Totowa 2004, 251, 3–8.
- Park, E.-Y.; Morimae, M.; Matsumura, Y.; Nakamura, Y.; Sato, K. Antioxidant Activity of Some Protein Hydrolysates and Their Fractions with Different Isoelectric Points. Journal of Agricultural and Food Chemistry 2008, 56, 9246–9251. DOI: 10.1021/jf801836u.
- Rajapakse, N.; Mendis, E.; Jung, W.-K.; Je, J.-Y.; Kim, S.-K. Purification of a Radical Scavenging Peptide from Fermented Mussel Sauce and Its Antioxidant Properties. Life Sciences 2005, 76, 2607–2619. DOI: 10.1016/j.lfs.2004.12.010.
- Mendis, E.; Rajapakse, N.; Byun, H.-G.; Kim, S.-K. Investigation of Jumbo Squid (Dosidicus Gigas) Skin Gelatin Peptides for Their in Vitro Antioxidant Effects. Life Sciences 2005, 77, 2166–2178. DOI: 10.1016/j.lfs.2005.03.016.
- Zhang, J.; Liu, S.; Zhang, Y.; Lu, Y.; Wang, M.; Wang, G.; Liu, X. Purification and Antioxidant Ability of Peptide from Egg in Sea Cucumber Apostichopus Japonicus. International Journal of Food Properties 2016, 20, 306–317. DOI: 10.1080/10942912.2016.1160409.
- Shen, S.-W.; Chahal, B.; Majumder, K.; You, S.-J.; Wu, J.-P. Identification of Novel Antioxidative Peptides Derived from a Thermolytic Hydrolysate of Ovotransferrin by LC-MS/MS. Journal of Agricultural and Food Chemistry 2010, 58, 7664–7672. DOI: 10.1021/jf101323y.
- Megías, C.; Pedroche, J.; Yust, -M.-M.; Girón-Calle, J.; Alaiz, M.; Millán, F.; Vioque, J. Production of Copper-Chelating Peptides after Hydrolysis of Sunflower Proteins with Pepsin and Pancreatin. LWT - Food Science and Technology 2008, 41, 1973–1977. DOI: 10.1016/j.lwt.2007.11.010.