ABSTRACT
The effect of different metal ions including K+, Na+, Mg2+, and Ca2+ at different concentrations on the self-assembly of chitosan molecules deposited on the new cleaved mica sheet was investigated using atomic force microscopy imaging techniques and other affiliated offline analysis methods. Chitosan molecules self-assembled porous film first when combined with K+, Na+, or Mg2+ ions, and then gradually fractured a granular structure with increasing concentration of metal ions. Chitosan molecules would be self-assembled to fibrous structure after adding calcium ions, and grew much thicker and more flat when the concentration increased. The investigations on the effects of metal ions on the self-assembly of chitosan molecules would be useful for food preservation, environmental protection, and pharmaceutical developments and industries.
Introduction
Chitosan is the N-deacetylated product of chitin, where the degree of deacetylation is generally more than 60%. Chitosan is less widespread in biomass, being produced mainly by thermochemical alkaline deacetylation of acetamide group at the C-2 position in the 2-acetamido-2-deoxy-d-glucopyranose (GlcNAc) unit.[Citation1,Citation2] Chemically, chitosan is a linear polysaccharide containing GlcNAc and 2-amino-2-deoxy-d-glucopyranose (GlcN) units joined by β(1→4) glycosidic bonds.[Citation3] As an important natural polymer, besides excellent physical and mechanical performance, chitosan has attractive properties like biodegradability, bioactivity, and biocompatibility. Chitosan has received more attention for its wide range of applications in food science, biology, medicine, cosmetics, environmental protection, which contain potential application value. [Citation4–Citation9] Accordingly, new patterns are urgent to equip self-assembled structures of chitosan such as nanowires, hydrogels, membranes, and emulsions which could be applied to various fields. [Citation10,Citation11]
As a hydrophilic biopolymer, chitosan is a compound with intramolecular hydrogen bond owing to the presence of plenty of free –NH2 and –OH in each unit, which allows chitosan to have a strong chelating ability with metal ions.[Citation12–Citation15] Chitosan self-assembled structures are highly desirable for various applications with controllable morphology.[Citation16] However, very little attention has been paid on the self-assembled structures of chitosan on mica sheet, particularly the effects of local environments which might be very important to control self-assembled structures. In this work, chitosan structures on a mica surface in a controllable fashion were investigated by atomic force microscopy (AFM), which was unlike the previous optical microscopic and kinetic study. A lot of information on the self-assembled structures of chitosan on mica surface was revealed by AFM studies. Through previous researches, it was found that solvents, concentration, pH, and temperature were all key factors for the formation of self-assembled structures of chitosan on mica surface.[Citation17,Citation18] In this study, the effects of chitosan concentration and metal ions on chitosan filming shape were investigated in detail using AFM, and the film of chitosan molecules had made improvements ultimately. These results are useful for the function relationship study of chitosan and helpful for research and development in products of food preservation, environmental protection, cosmetic, medicine and other aspects.
Materials and methods
Preparation of chitosan solution for AFM imaging
One percent of acetic acid (Guangdong Guanghua Chemical Factory Co., Ltd) at pH 2.8 was used as the solvent to dissolve chitosan (Sinopharm Chemical Reagent Co., Ltd) and chlorate (Chengdu Kelong Chemical Rea gent Factory). To obtain 10 mg/mL chitosan solution, 2 g of chitosan were dissolved in 200 mL 1% acetic acid solution. High concentration of chitosan solution was further diluted to prepare other different concentrations of chitosan solutions. Original 5.0 M chlorate solutions were diluted to different concentrations for further use. All these solutions were stored at 4°C. Then, 10 μl of chitosan solution with different metal ions were deposited onto the freshly cleaved mica sheets of 1.0 × 0.5 cm2, respectively. The mica sheets were placed and drought in sealed desiccators at room temperature (15–20°C). Finally, the mica sheets were fixed onto the sample mounting disk with diameter of 12 mm for AFM imaging.
AFM imaging of chitosan assembly
A Multimode-8 AFM (Bruker Co., Santa Barbara, USA) was employed in ScanAsyst mode with ScanAsyst-AIR probes for topology of chitosan assembly at 0.977 Hz. The AFM detected the topography of the sample by using a flexible microcantilever with one end fixed and a nanometer tip on the other end. When the sample was scanned under the tip, the tip–sample interaction causes microcantilever deformation. That was to say, the microcantilever deformation is a direct reflection of the sample–tip interaction. [Citation19] Typical height images were collected for the further data analysis.
Data analysis
The offline software NanoScopeAnalysis V1.10 (Bruker Co.) was used to do measurement and statistical analysis with the typical height images. Section profiles, average roughness (Ra), root mean square roughness (Rq), and surface area difference had been collected after a first-order flatten and noisy line erasing to describe the topological change. Then, the data were analyzed using analysis of variance to determine the significant differences among the groups and p < 0.05 was considered statistically significant. All data were expressed as mean ± SD. Analyses were performed using Excel 2010 (Microsoft Co., Redmond, USA) and SPSS22.0 (IBM Corp., Armonk, USA).
Results and analysis
Self-assembly of chitosan in different chitosan concentrations
Typical AFM height images and section profiles of chitosan assembly in different concentrations were shown in . Height, roughness (Ra, Rq), and surface area difference were shown in . It was shown that the morphology of self-assembled chitosan in different concentrations had a significant difference.
Table 1. Height, roughness (Ra, Rq), and surface area difference of the chitosan molecular self-assembly in different chitosan concentrations and 0.05 mg/mL chitosan self-assembly in different KCl, NaCl, MgCl2, and CaCl2 concentrations.
Figure 1. Typical AFM height images and section analysis profiles of chitosan self-assembly in different concentrations in 512 × 512 pixels. Scale bar = 1um.
(a) 0.01 mg/mL; (b) 0.02 mg/mL; (c) 0.05 mg/mL; (d) 0.1 mg/mL.
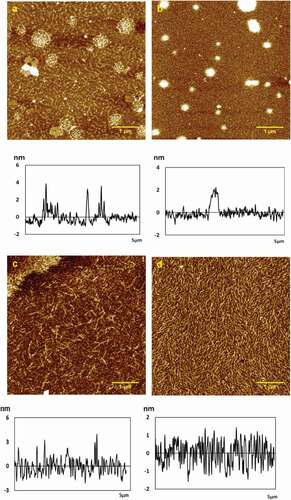
At 0.01 mg/mL, the chitosan molecules self-assembled into small particles: some arranged regularly in ripple, while others gathered into groups with the height of 3.2 ± 0.3 nm, Ra of 0.466 nm, Rq of 0.692 nm, and surface area difference of 0.105% (see ). When the concentration increased slowly to 0.02 mg/mL, chitosan particles formed fine fibers and tiled densely on the mica sheet with the height of 2.9 ± 0.2 nm, Ra of 0.323 nm, Rq of 0.544 nm, and surface area difference of 0.063%, as shown in . In addition, there are many spherical particles which gathered more closely from 0.01 to 0.02 mg/mL. When increased to 0.05 mg/mL, the molecular chains of chitosan accumulated to short and thick fibers clearly after covering the substrate at the height of 3.4 ± 0.1 nm, Ra of 0.896 nm, Rq of 1.27 nm, and surface area difference of 0.387%, which were much lower than that in other concentrations (see ). As the concentration increased to 0.1 mg/mL, the chitosan fibers covered a dense substrate with the height of 1.9 ± 0.1 nm, Ra of 0.526 nm, Rq of 0.642 nm, and surface area difference of 0.209% (see ). The height of chitosan particles and fibers in different concentrations was reflected in section analysis profiles below the corresponding height images, as shown in –1d.
The intermolecular and intramolecular hydrogen bonds played a key role in chain enwinding. The permutation of chitosan membrane molecules varied with concentration. In low concentrations, the molecules were arranged orderly. When the concentration was slightly higher, the molecules were arranged in a disorderly way, which can affect the mechanical strength of the film. If the concentration was too high, the solution of chitosan was glutinous and difficult to deaerate, and the coating was thicker and uneven. If the concentration was too low, the chitosan solution had large flow ability, the film thickness was not uniform, and it was difficult to format and remove the film. [Citation20] In addition, there were many spherical particles in , which should be impurity that resulted from chitosan sample itself, or chitosan fragments .There was large difference in height, and white particles caused by large local concentration. It was not suitable for observing the morphological details of the self-assembled chitosan. So, the 0.05 mg/mL chitosan solution was selected to study the impact of metal ions.
Effect of KCl on self-assembly of chitosan molecules
AFM images of chitosan assemblies on mica surface in the presence of various concentration of KCl (0.2, 0.5, 1.0 mg/mL) were shown in . Height, roughness (Ra, Rq), and surface area difference were shown in . In the presence of 0.2 mg/mL KCl, 0.05 mg/mL chitosan assembled porous membrane structure with the height of 3.8 ± 0.2 nm, Ra of 0.782 nm, Rq of 1.14 nm, and surface area difference of 0.672% (see ). With the KCl concentration increased to 0.5 mg/mL, chitosan film splited into large granular structure with the height of 3.5 ± 0.1 nm, Ra of 0.722 nm, Rq of 0.939 nm, and surface area difference of 0.215%, as shown in . Similar to the previous report, there were large particles of irregular polygonal configuration. At 1.0 mg/mL KCl, large particles were getting into small particles with uniform distribution, and the height was 3.01 ± 0.03 nm, and Ra was 0.175 nm, Rq was 0.307 nm, surface area difference was 0.206% (see ). It was shown that chitosan molecules assembled into the porous membrane on the mica sheet at low concentrations. With the increase of the concentration, the height, roughness (Ra, Rq), and surface area difference of the chitosan assembly became lower; the membrane splited into larger granular structure; and the larger particles were getting into small particles with uniform distribution at high concentrations. The –NH2, –OH and other groups in the chitosan molecules have coordinated with the metal ions, resulting in the change of the intermolecular interactions of the chitosan, which affects the surface shape of the membrane. K+ ions are alkaline metal ions, and their ionic radius is large, the charge is small, and the electronegativity is small. Therefore, the electrostatic attraction between the electron pairs is small when the complexation reaction occurs, and the tendency to form a complex with chitosan is also smaller. But the coordination reactions will still occur, which will affect the self-assembly structure of chitosan.
Effect of NaCl on self-assembly of chitosan molecules
AFM images of chitosan assemblies on mica surface in the presence of various concentration of NaCl (0.2, 0.5, 1.0 mg/mL) were shown in . Height, roughness (Ra, Rq), and surface area difference were shown in . When 0.2 mg/mL NaCl was added, 0.05 mg/mL chitosan assembled a porous membrane structure that was presented obviously with the height of 1.57 ± 0.07 nm, Ra of 0.324 nm, Rq of 0.400 nm, and surface area difference of 0.279% (see ). The membrane pores which evenly distributed grew in number at 0.5 mg/mL NaCl with the height of 1.4 ± 0.2nm, Ra of 0.316 nm, Rq of 0.491 nm, and surface area difference of 0.120% respectively, as shown in . When the concentration of NaCl solution increased to 1.0 mg/mL, chitosan film ruptured to form a granular structure with the height of 1.2 ± 0.1 nm, Ra of 0.425 nm, Rq of 0.598 nm, and surface area difference of 0.382%, which were higher than that in other concentrations (see ). It turned out that the amounts of the pores increased with the increase of the concentration, and the porous membrane broke into small particles with uniform distribution at higher concentrations. Na+ ions are an alkaline metal ions like K+ ions, but their ionic radius is larger than that of potassium ions, so the electrostatic attraction between electron pairs is stronger than that of potassium ions. The self-assembled film formed by sodium ion and chitosan has better roughness and surface area difference than potassium ion in general.
Effect of MgCl2 on self-assembly of chitosan molecules
Typical AFM height images of chitosan assemblies on mica surface in the presence of MgCl2 in various concentration of 0.2, 0.5, 1.0 mg/mL were shown in . Height, roughness (Ra, Rq), and surface area difference were shown in . When 0.05 mg/mL chitosan combined with MgCl2 at a low concentration, the multilayer film structure was first formed, as shown in apparently, the height variation graph showed that there were three layers films at least with the height of 9.9 ± 0.2 nm, which was much higher than that in other concentration. And Ra, Rq, surface area difference were 2.15, 3.01 nm and 0.484%, respectively. When the MgCl2 concentration increased, the multilayer film began to translate into a single-layer film with the numbers of film pore increasing, and the height was 1.9 ± 0.2 nm, Ra was 0.373 nm, Rq was 0.630 nm, and surface area difference was 0.154% (see ). The multilayer film even ruptured in the end with the height of 1.5 ± 0.1 nm, Ra of 0.771 nm, Rq of 1.48 nm, and surface area difference of 0.618% (see ). It indicated that the chitosan molecules assembled into the multilayer film at low concentration. With the increase of the concentration, the number of layers decreased. At the same time, the number of pores increased. Finally, the film ruptured at high concentration. Mg2+ belongs to alkaline-earth metal ions, which have small ionic radius and high charge. They are strong electron acceptors and can easily form complexes with chitosan, thus affecting chitosan self-assembly structure.
Effect of CaCl2 on self-assembly of chitosan molecules
AFM images of chitosan assemblies on mica surface in the presence of various concentration of CaCl2 (0.2, 0.5, 1.0 mg/mL) were shown in . Height, roughness (Ra, Rq), and surface area difference were shown in . At 0.2 mg/mL CaCl2, 0.05 mg/mL chitosan assembled a porous membrane structure with the height of 5.4 ± 0.2 nm, Ra of 0.693 nm, Rq of 1.19 nm, and surface area difference of 0.630%, as shown in . As was seen obviously, long fibers in the film intertwined with each other inseparably. As the concentration of CaCl2 increased to 1.5 mg/mL, the thickness of chitosan film increased, the numbers of the pores reduced clearly with fine fibers becoming thicker with the height of 4.4 ± 0.2 nm, Ra of 0.826 nm, Rq of 1.24 nm, and surface area difference of 0.417% (). At 1.0 mg/mL CaCl2, crude fibers disappeared; thick film appeared with pores at the height of 1.6 ± 0.1 nm, Ra of 0.461 nm, Rq of 0.538 nm, and surface area difference of 0.202% (). It concluded that with the concentration increasing, the height, roughness (Ra, Rq), and surface area difference became lower. And the fibers in the film gradually became thicker, and at high concentrations, the fiber became thick and flat membrane with numbers of holes. The calcium ion only complexes with the –NH2 on the chitosan molecule, but since the chitosan solution is acidic and the amino group exists in the form of NH3+, the coordination ability of the chitosan to the calcium ion is greatly reduced. This results in the effect of calcium ions on the self-assembly structure of chitosan showing different rules from other metal ions.
Conclusion
The studies on the morphology of chitosan self-assembly in the presence of four metal cations (K+, Na+, Mg2+, and Ca2+) by AFM can lead to the following conclusions. Compared to single chitosan self-assembly, chitosan assembled on mica surface in the presence of four metal cation tends to cross-link and gather into film more easily. However, there are some differences in the effect of different metal ions on the self-assembly of chitosan molecules. Combined with K+, Na+, or Mg2+, chitosan molecular self-assembled porous film, and with concentration increasing, the film thickness and the numbers of hole became smaller, and then membrane structure gradually fractured into pieces, and eventually became a granular structure. Chitosan molecules self-assembled porous membrane after adding calcium ions, and the numbers of hole increased with concentration increasing. The complex between metal ions and chitosan self-assembly is beneficial to chitosan film; this study might show new light on the formation of chitosan film. It will be helpful to study the characteristics of chitosan self-assembly which could provide theoretical guidance and data supports in food preservation, environmental protection, and medicine materials.
Additional information
Funding
References
- Guinesi, L. S.; Cavalheiro, E. T. G. The Use of DSC Curves to Determine the Acetylation Degree of Chitin/Chitosan Samples. Thermochimica Acta 2006, 444, 2, 128–133.
- Tsaih, M. L.; Chen, R. H. Molecular Weight Determination of 83% Degree of Decetylation Chitosan with Non‐Gaussian and Wide Range Distribution by High‐Performance Size Exclusion Chromatography and Capillary Viscometry. Journal of Applied Polymer Science 2015, 71, 11, 1905–1913.
- Signini, R.; Filho, S. P. C. On the Preparation and Characterization of Chitosan Hydrochloride. Polymer Bulletin 1999, 42, 2, 159–166.
- Tang, Z. X.; Qian, J. Q.; Shi, L. E. Preparation of Chitosan Nanoparticles as Carrier for Immobilized Enzyme. Applied Biochemistry & Biotechnology 2007, 136, 1, 77–96.
- Pan, J. R.; Huang, C.; Chen, S.; Chung, Y. C. Evaluation of a Modified Chitosan Biopolymer for Coagulation of Colloidal Particles. Colloids & Surfaces A Physicochemical & Engineering Aspects 1999, 147, 3, 359–364.
- Yuan, G.; Chen, X.; Li, D. Chitosan Films and Coatings Containing Essential Oils: The Antioxidant and Antimicrobial Activity, and Application in Food Systems. Food Research International 2016, 89, Pt 1, 117–128.
- Anchisi, C.; Meloni, M. C.; Maccioni, A. M. Chitosan Beads Loaded with Essential Oils in Cosmetic Formulations. Journal of Cosmetic Science 2007, 29, 6, 205–214.
- Rashid, S.; Shen, C.; Yang, J.; Liu, J.; Li, J. Preparation and Properties of Chitosan–Metal Complex: Some Factors Influencing the Adsorption Capacity for Dyes in Aqueous Solution. Journal of Environmental Sciences 2017, 66, 301–309.
- Choomchit, J.; Suesat, J.; Porntip, S. B. Chitosan as a Thickener for Direct Printing of Natural Dye on Cotton Fabric. Advanced Materials Research 2013, 613, 3394–3397.
- Yi, H.; Wu, L. Q.; Bentley, W. E.; Ghodssi, R.; Rubloff, G. W.; Culver, J. N.; Payne, G. F. Biofabrication with Chitosan. Biomacromolecules 2005, 6, 6, 2881–2894.
- Gong, J.; Zhou, Z.; Hu, X.; Wong, M.; Wong, K.; Du, Z. Self-Assembled Chitosan Nanotemplates for Biomineralization of Controlled Calcite Nanoarchitectures. ACS Applied Materials & Interfaces 2009, 1, 1, 26–29.
- Fang, N.; Chan, V. Chitosan-Induced Restructuration of a Mica-Supported Phospholipid Bilayer: An Atomic Force Microscopy Study. Biomacromolecules 2003, 4, 6, 1596–1604.
- Yang, X.; Tu, Y.; Li, L.; Shang, S.; Tao, X. M. Well-Dispersed Chitosan/Graphene Oxide Nanocomposites. ACS Applied Materials & Interfaces 2010, 2, 6, 1707–1713.
- Chu, E.; Sidorenko, A. Surface Reconstruction by a “Grafting Through” Approach: Polyacrylamide Grafted onto Chitosan Film. Langmuir 2013, 29, 40, 12585–12592.
- Dong, W. L.; Lim, C.; Israelachvili, J. N.; Dong, S. H. Strong Adhesion and Cohesion of Chitosan in Aqueous Solutions. Langmuir 2013, 29, 46, 14222–14229.
- Gong, J. M.; Hu, X. L.; Wong, K. W.; Zheng, Z.; Yang, L.; Lau, W. M.; Du, R. X. Chitosan Nanostructures with Controllable Morphology Produced by a Nonaqueous Electrochemical Approach. Advanced Materials 2010, 20, 11, 2111–2115.
- Wang, L.; Wu, J.; Guo, Y.; Gong, C.; Song, Y. Topographic Characterization of the Self-Assembled Nanostructures of Chitosan on Mica Surface by Atomic Force Microscopy. Applied Surface Science 2015, 353, 757–763.
- Pavinatto, F. J.; Caseli, L.; Oliveira, O. N. Chitosan in Nanostructured Thin Films. Biomacromolecules 2010, 11, 8, 1897–1908.
- Binnig, G.; Quate, C. F.; Gerber, C. Atomic Force Microscope. Physics Review Letter 1986, 56, 9, 930–933.
- Xu, Y.; Hong, H.; Qian, Y.; Liu, C. S. Preparation and Characteration of Biomedical Chitosan Films. Journal of Functional Polymers 2004, 3, 1, 55–60.