ABSTRACT
In this study, the fabrication of almond gum/polyvinyl alcohol (PVA) composite nanofibers loaded with curcumin or its β-cyclodextrin inclusion complex (βCDIC) using electrospinning process was studied. The preparation of inclusion complex was done using freeze drying method. The characterization of electrospun nanofibers was studied by FESEM, XRD, TGA and FTIR analysis. FESEM results showed that the fiber diameters were in the range of 98–169 nm. XRD analysis indicated the crystalline aggregates of curcumin in the fiber matrix, whereas curcumin-βCDIC did not show crystalline aggregates. Thermal stability of nanofibers increased after incorporation of curcumin and curcumin-βCDIC into them. FTIR analysis confirmed the formation of the complex between curcumin and βCD. The solubility of curcumin and curcumin-βCDIC incorporated into nanofibers were increased up to 70 and 160 times compared with pure curcumin, respectively. The results showed that almond gum nanofibers can be used as a suitable carrier for hydrophobic compounds to enhance their solubility for application in the food and pharmaceutical industries.
Introduction
Curcumin [1,7-bis(4-hydroxy-3-methoxyphenyl)-1,6-heptadien-3,5-dione] is a hydrophobic polyphenolic pigment which is found in the rhizomes of the plant Curcuma longa. C. Curcumin has been used as a therapeutic compound in traditional medicine in China and India.[Citation1] Curcumin is an antioxidant compound[Citation2,Citation3] which possesses antimicrobial[Citation4], anti-inflammatory[Citation5], anticancer[Citation6] and wound-healing properties.[Citation7] Also, curcumin has a yellow-orange color and has been used as a natural food color.
However, there are some restrictions which limit the application of curcumin in pharmaceutics or food industry such as: low water solubility[Citation8] which decreases its dispersion in food or aqueous solution, low bioavailability which decreases its biological efficiency[Citation9], sensitivity to light, thermal treatment and neutral or alkaline pH conditions which cause to degrade rapidly when exposed to these circumstances.[Citation10] Also, high dosage and rapid metabolism limit its therapeutic effect.[Citation11] In situ delivery of curcumin can enhance its stability and therapeutic effect.[Citation12] Therefore, the incorporation of curcumin in different carriers or delivery system can prevail to these limitations.
Cyclodextrins (CDs) are cyclic oligosaccharides derived from starch. CDs have a hydrophobic cavity and a hydrophilic shell. The hydrophobic compounds can make a non-covalent bond with the hydrophobic cavity and form an inclusion complex and therefore increase their aqueous solubility and stability.[Citation13] CDs are composed of six to eight units of glucopyranose which have different diameters of the hydrophilic cavity. The three most common CDs are α, β, and γ which have 6, 7 and 8 units, respectively. It is demonstrated that curcumin can complex with βCD in 2:1 molar ratio of βCD: curcumin. Curcumin has two phenyl rings and each of them is incorporated into a separated βCD host.[Citation14]
Electrospinning is a versatile and economic technique that produce nanofibers and has been received a lot of attention in recent years. Electrospun nanofibers have many attractive properties such as large surface to volume ratio which make them suitable for use as a delivery system for other compounds.[Citation15]
Almond gum is a high molecular weight polysaccharide which is suitable for electrospinning. Almond gum is composed of two fractions: water soluble fraction (90%w/w) and water insoluble fraction (10%w/w).[Citation16] Electrospun almond gum nanofibers are appropriate carriers for delivering the sensitive compounds such as vanillin and can preserve them from unsuitable conditions.[Citation17] Also, the solubility of insoluble compounds can be increased by encapsulation into the produced nanofibers of the water soluble fraction.
The purpose of this study was encapsulation of curcumin and its βCDIC using almond gum/PVA electrospun nanofibers. The characterization of curcumin and curcumin-βCDIC incorporated into the nanofibers are investigated and the solubility of curcumin is studied as well.
Materials and methods
Materials
Almond gum exudates were collected from the trunk and branches of almond trees (Amygdalus communis L.) in Saman, Chaharmahal and Bakhtiari Province, Iran. The water soluble fraction of almond gum with Mw = 15.9 × 103 KDa, Mn = 5.02 × 103 KDa and PDI = 3.16 was used for electrospinning.[Citation16] Polyvinyl alcohol (PVA) (Mw: 145000, fully hydrolyzed) and curcumin were purchased from Merck (Merck, Germany). βCD was purchased from SD-fine corporation, India.
Preparation of inclusion complex
The inclusion complex was prepared by freeze drying method described by Mohan et al (2012) with a few modifications.[Citation18] 0.25g of curcumin was dissolved in 10 ml of ethanol (96% w/w) at 60 ºC for 10 min. 1.54 g of βCD was dissolved in 30 ml of water at 75 ºC for 5 min. Then curcumin was added dropwise to the aqueous solution of βCD under continuous stirring. The stoichiometry of curcumin and βCD was fixed at 1:2. The solution was stirred for 7 days at 37 ºC and then filtered through 0.45 µm filter. The clear solution was freeze dried at −40 ºC for 24 h to obtain solid complex (Dena Vacuum, Iran).
Preparation of the electrospinning solution
To prepare the electrospinning solutions (), the specified amount of PVA was dissolved in deionized water and stirred at 80 ºC for 2 h. Then, almond gum was added to the PVA solution and stirred for 1 h at 45 ºC and then hydrated overnight in a refrigerator. The proportion of almond gum/PVA was fixed at 80:20 (w/w). In the next step, the determined amount of curcumin or curcumin-βCDIC was added to this solution and stirred for 8 h at room temperature. Finally, the solution was left to stabilize for 1 h before electrospinning.
Table 1. The properties of electrospinning solutions and electrospun nanofibers.
Electrospinning procedure
The electrospinning solutions were filled into a 1 ml syringe (needle inner diameter = 0.8 mm) and held in a horizontal position. Production of nanofibers was done at ambient conditions (25 ⁰C and 30% relative humidity) using electrospinning (Fanavaran Nano-Meghyas, Iran). The applied voltage was 18 kV, the flow rate was 0.125 ml/h and nozzle-collector distance was 15 cm.
Measurement and characterization
The morphology of electrospun nanofibers was analyzed by Field emission scanning electron microscope (FESEM). The samples were sputtered with gold prior to imaging (HITACHI S-4160, Korea). The average diameter of nanofibers was calculated using Digimizer software to around 60 fibers in the FESEM images. The viscosity of the solutions was measured using RVDV-II Brookfield viscometer (Brookfield, USA) equipped with a small sample adapter (spindle speed = 10 rpm, time = 2 min, sample volume = 8 ml) at room temperature with spindle 21. The conductivity of the solutions was measured at room temperature by a digital conductivity meter (model no 103, Taiwan).
Fourier transform infrared spectroscopy (FTIR) analysis of curcumin powder, βCD powder, curcumin-βCDIC powder, almond gum/PVA nanofibers, almond gum/PVA/curcumin nanofibers, and almond gum/PVA/curcumin-βCDIC nanofibers were conducted with a Tensor 27 spectrometer (Bruker, Germany) over the frequency range of 4000-600cm−1 and resolution of 4cm−1 using the ATR pellet method. OPUS software was applied to analyze infrared spectra. The baseline of spectra was corrected and then smoothed by 11 points using the Savitzky-Golay algorithm. The spectra were finally normalized using the min‒max normalization method.
The X-ray diffraction (XRD) patterns of PVA powder, almond gum powder, curcumin powder, βCD powder, curcumin-βCDIC powder, almond gum/PVA nanofibers, almond gum/PVA/curcumin nanofibers, and almond gum/PVA/curcumin-βCDIC nanofibers were obtained on a Philips X’Pert diffractometer using Cu Kα radiation (λ = 1.54 °A) over the 2θ range of 10–60⁰ with step size of 0.05 ⁰. The XRD spectra were treated by X’Pert and Origin software.
Thermal properties of curcumin powder, βCD powder, curcumin-βCDIC powder, almond gum/PVA nanofibers, almond gum/PVA/curcumin nanofibers, and almond gum/PVA/curcumin-βCDIC nanofibers were analyzed by the thermogravimetric analyzer (TGA) (STA 503, Germany). The TGA data were recorded from room temperature to 600 ⁰C, at a heating rate of 10 ⁰C/min, under argon atmosphere.
Solubility of curcumin
Excess amounts of almond gum/PVA/curcumin nanofibers and almond gum/PVA/curcumin-βCDIC nanofibers samples were dispersed into the 30 ml of distilled water separately to obtain the supersaturated solutions. The samples were agitated for 24 h at room temperature until reach to equilibrium. 5 ml of the supersaturated solutions was filtered through a 0.45 μm filter and diluted with methanol to reach a suitable dilution. The absorbance of the samples was read at 425 nm.[Citation19,Citation20]
Results and discussion
FESEM results
FESEM images of almond gum/PVA/curcumin nanofibers and almond gum/PVA/curcumin-βCDIC nanofibers with different concentrations of curcumin and curcumin-βCDIC, respectively, are presented in . In the cases of almond gum/PVA/curcumin nanofibers, the nanofibers containing 1% (w/w) and 2% (w/w) curcumin showed smooth structure without any bead while by increasing the concentration of curcumin to 3% (w/w) and 4% (w/w) some beads appeared in fiber strong. This may be due to the low solubility of curcumin which caused the aggregation in spinning solution. Also, the average fiber diameter was decreased by increasing the amount of curcumin (). This may be related to the increasing solution conductivity by increasing the amount of curcumin. Suwantong et al (2007) and Sun et al (2013) also obtained a similar result, namely, by increasing the amount of curcumin the average fiber diameter was decreased.[Citation21,Citation22]
Figure 1. FESEM images of almond gum/PVA/curcumin and almond gum/PVA/curcumin-βCDIC nanofibers, a) almond gum/PVA 8% (w/w) + curcumin 1% (w/w), b) almond gum/PVA 8% (w/w) + curcumin 2% (w/w), c) almond gum/PVA 8% (w/w) + curcumin 3% (w/w), d) almond gum/PVA 7% (w/w) + curcumin 4% (w/w), e) almond gum/PVA 8% (w/w) + curcumin-βCDIC 2% (w/w), f) almond gum/PVA 8% (w/w) + curcumin-βCDIC 3% (w/w), g) almond gum/PVA 7% (w/w) + curcumin-βCDIC 4% (w/w), h) almond gum/PVA 7% (w/w) + curcumin-βCDIC 5% (w/w).
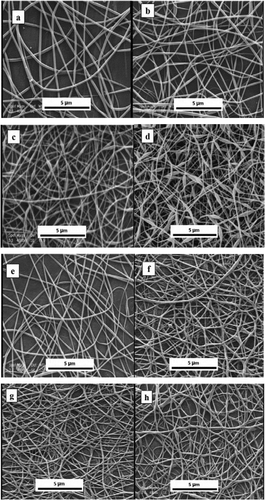
For the almond gum/PVA/curcumin-βCDIC nanofibers, the nanofibers containing 2% (w/w), 3% (w/w) and 4% (w/w) of curcumin-βCDIC were smooth and bead free. By increasing the concentration of curcumin-βCDIC to 5% (w/w) some beads appeared in the nanofibers due to the higher viscosity of the solution. The average diameter of nanofibers decreased by increasing the curcumin-βCDIC concentration that is due to the higher solution conductivity as previously mentioned. βCD can decrease the interfacial tension between almond gum/PVA solution and curcumin due to its surfactant-like properties and therefore resulted in higher solubility of curcumin.[Citation23,Citation24] The higher solubility of curcumin led to the higher stability of solution and therefore showed nanofibers with the lower diameter in comparison with the solution containing pure curcumin.
FTIR analysis
The FTIR spectra of curcumin powder, βCD powder, curcumin-βCDIC powder, almond gum/PVA nanofibers, almond gum/PVA/curcumin nanofibers, and almond gum/PVA/curcumin-βCDIC nanofibers are depicted in . For curcumin, the broad absorption band at 3426 cm−1 and the sharp peak at 3508 cm−1 are attributed to the phenolic O-H stretching vibration. The bands at 1628 cm−1and 1592 cm−1 are related to carbonyl group C = O and stretching vibrations of the benzene ring, respectively. The bands at 1432 cm−1 and 1508 cm−1 are related to olefinic bending vibration of C–H bound to the benzene ring of curcumin and C-C vibrations, respectively.[Citation25,Citation26] The bands at 1277 cm−1 and 1154 cm−1 are due to aromatic C = O stretching and C-O-C stretching modes, respectively.[Citation22]
Figure 2. FTIR spectra of curcumin (cur) powder, βCD powder, curcumin-βCDIC powder, almond gum/PVA nanofibers, almond gum/PVA/curcumin nanofibers and almond gum/PVA/curcumin-βCDIC nanofibers.
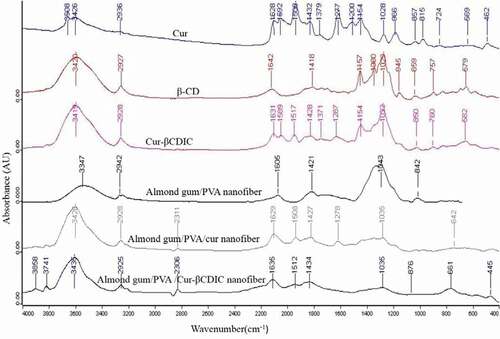
For the FTIR spectrum of βCD, vibrations observed at 3420 cm−1 corresponding to OH stretching. The Peak observed at 2927 cm−1 is related to symmetric/asymmetric C-H stretching. The peak at 1642 cm−1 is attributed to the deformation band of water H-O-H. The peak at 1157 cm−1 is associated with C-H overtone vibrations. The appearance of the sharp peak at 1029 cm−1 corresponds to the C-O-C glucose units of βCD.[Citation18]
In curcumin-βCDIC FTIR spectrum, sharpening of the peak at 3419 cm−1 is observed which is due to the increased inter molecular H bonding in inclusion complex. The inclusion complex may be formed by the interaction among C = C, C = O, -OH and aromatic ring present in βCD and curcumin. The Shift of peaks in the range of 1000-4000cm−1 supports the formation of the complex. The strong peak at 1277 cm−1 and 1508 cm−1 of curcumin shifted to 1287 cm−1 and 1517 cm−1 in the complex, respectively and their intensity decreased which explains the formation of the complex. The appearance of the peak at 1589 cm−1 in the complex is related to the peak at 1592 cm−1 of curcumin.
The FTIR spectrum of almond gum/PVA nanofibers shows a broad band at 3347 cm−1 which is related to the stretching vibration of O-H groups. The peak at 2942 cm−1 is attributed to asymmetric C-H2 functional groups. The band at 1605 cm−1 may be related to asymmetrical stretching of carboxylate groups or intramolecularly bound water. The peaks at 1421 cm−1 and 1043 cm−1 correspond to symmetrical stretching of carboxylate groups of uronic acid residues in almond gum and the stretching vibration of alcoholic groups, respectively. It is important to note that the wave number range between 1500 and 500 cm−1 represents the carbohydrate fingerprints.[Citation16]
The FTIR spectrum of almond gum/PVA/curcumin nanofibers shows that the peaks at 1277 cm−1 and 1508 cm−1 are related to curcumin. The intensity of the broad peak at 1043 cm−1 in almond gum/PVA nanofibers is decreased and shifted to 1035 cm−1 in nanofibers of almond gum/PVA/curcumin.
The FTIR spectrum of almond gum/PVA/curcumin-βCDIC nanofibers shows that the peak at 1287 cm−1 which is related to curcumin-βCDIC is disappeared. This may be due to the interaction between almond gum/PVA nanofibers and curcumin-βCDIC. The intensity of the broad peak at 1043 cm−1 in almond gum/PVA nanofibers is decreased and shifted to 1035 cm−1 in almond gum/PVA/curcumin-βCDIC nanofibers.
XRD analysis
XRD patterns of curcumin powder, βCD powder, curcumin-βCDIC powder, almond gum powder, PVA powder, almond gum/PVA nanofibers, almond gum/PVA/curcumin nanofibers, and almond gum/PVA/curcumin-βCDIC nanofibers are depicted in . XRD pattern of curcumin has a crystalline structure and shows a sharp diffraction peak centered at 2θ≈ 15º. βCD has a semi crystalline structure. The XRD pattern of curcumin-βCDIC did not show the sharp diffraction peak of curcumin. As can be seen, some diffraction peaks of curcumin and βCD are absent in the XRD pattern of curcumin-βCDIC. Curcumin is incorporated into the βCD matrix and a true inclusion complex between curcumin and βCD is formed. As previously mentioned, the hydrophobic compounds such as curcumin can make a non-covalent bond with the hydrophobic cavity of βCD and form an inclusion complex. In the inclusion complex, the guest molecules are distinct from each other and therefore cannot form crystals.[Citation27] The XRD patterns of almond gum and PVA show amorphous and semi-crystalline structures, respectively. The XRD pattern of almond gum/PVA nanofibers shows an amorphous structure and is very similar to the almond gum pattern. The crystalline peaks of curcumin do not appear in the XRD pattern of almond gum/PVA/curcumin-βCDIC nanofibers while in the XRD pattern of almond gum/PVA/curcumin the sharp peak of curcumin in 2θ≈ 15º appear. These results indicate that the true inclusion complexation between curcumin and βCD is kept even after the electrospinning process.
TGA analysis
Thermal stabilities of curcumin powder, βCD powder, curcumin-βCDIC powder, almond gum/PVA nanofibers, almond gum/PVA/curcumin nanofibers, and almond gum/PVA/curcumin-βCDIC nanofibers were evaluated by thermogravimetric analysis (TGA) and derivative thermosgravimetric (DTG) ( and ).
Figure 4. TGA thermograms of the curcumin (cur) powder, βCD powder, curcumin-βCDIC powder, almond gum/PVA nanofibers, almond gum/PVA/curcumin nanofibers and almond gum/PVA/curcumin-βCDIC nanofibers.
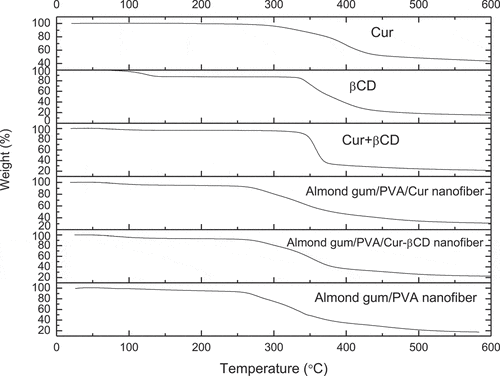
Figure 5. DTG curves of the curcumin (cur) powder, βCD powder, curcumin-βCDIC powder, almond gum/PVA nanofibers, almond gum/PVA/curcumin nanofibers and almond gum/PVA/curcumin-βCDIC nanofibers.
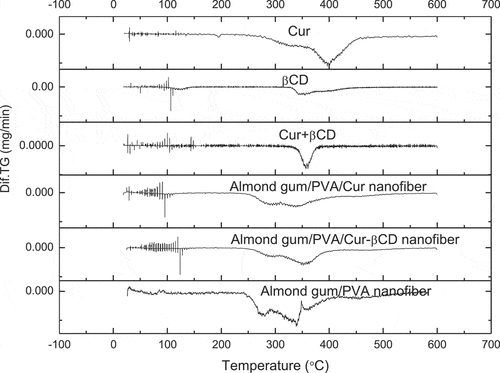
According to the , the initial weight loss below 130°C in all samples can be related to water evaporation. The TGA thermogram of curcumin shows that the main decomposition is started at around 270°C and continued up to 430°C. The TGA thermogram of βCD shows two weight loss gradients. The first weight loss (70°C to 130°C) is attributed to water evaporation and the second weight loss (340°C to 420°C) is related to the main degradation. The second weight loss of curcumin-βCDIC is started at around 330°C and continued up to 370°C which is related to its degradation. As can be seen, the thermal stability of curcumin is enhanced from 270°C to 330°C after incorporation into the matrix of βCD.
For almond gum/PVA nanofibers, the second weight loss (240°C to 345°C) is attributed to the main decomposition of almond gum and the third weight loss (366°C to 550°C) is related to the degradation of residue almond gum and PVA chains. The second weight loss of almond gum/PVA/curcumin nanofibers (256°C to 350°C) is due to the decomposition of almond gum/PVA nanofibers and some part of curcumin and third weight loss (390°C to 550°C) is related to the residue almond gum/ PVA nanofibers and the main part of curcumin. For almond gum/PVA/curcumin-βCDIC the second weight loss (260°C to 393°C) is attributed to the decomposition of almond gum/PVA nanofibers and some parts of curcumin-βCDIC and third weight loss (417°C to 550°C) is related to the residue almond gum/ PVA nanofibers and the main part of curcumin-βCDIC. As can be seen the onset of the main decomposition in almond gum/PVA/curcumin nanofibers and almond gum/PVA/curcumin-βCDIC nanofibers is somehow higher than almond gum/PVA nanofibers that is related to presence of curcumin and βCDIC in the nanofibers which have higher thermal stability than almond gum/PVA nanofibers.
DTG curves () show points at which weight losses reached their maximum rates. presents the thermogravimetric data for almond gum/PVA nanofibers, almond gum/PVA/curcumin nanofibers and almond gum/PVA/curcumin-βCDIC nanofibers during heating. As can be seen the onset temperature of thermal degradation and temperature (ºC) at max. loss rate in almond gum/PVA/curcumin-βCDIC nanofibers is higher than almond gum/PVA/curcumin nanofibers and almond gum/PVA nanofibers which is related to presence of βCD with high thermal stability.
Table 2. Thermogravimetric data of almond gum/PVA nanofibers, Almond gum/PVA/Curcumin nanofibers and Almond gum/PVA/ curcumin-βCDIC nanofibers.
Solubility test
The water solubility of curcumin, which was complexed with βCD is increased up to 50 folds compared with the pure curcumin. The solubility of curcumin and curcumin-βCDIC which are incorporated into almond gum/PVA nanofiber are enhanced to 70 and 160 folds, respectively, in comparison of pure curcumin. The soluble fraction of almond gum and also PVA can completely dissolve in water and therefore have a positive effect on curcumin solubility. Several researchers also have increased the solubility of curcumin using complexation with CD. Mangolim et al (2014) produced the curcumin-βCDIC using different methods.[Citation28] They observed that the water solubility of complexed curcumin compared with pure curcumin was increased up to 31 and 28 fold using co-precipitation and freeze drying methods, respectively. Increasing the solubility of curcumin can be resulted in enhanced circulation time in the body and finally improves its bioavailability.
Conclusion
In this study, nanofibers containing almond gum/PVA/curcumin or almond gum/PVA/curcumin-βCDIC were successfully prepared by electrospinning. FESEM results showed that the almond gum/PVA solutions containing curcumin-βCDIC produced nanofibers with the lower diameter in comparison of the almond gum/PVA solutions containing pure curcumin. The diameter of nanofibers decreased with increasing curcumin or curcumin-βCDIC content. XRD analysis suggested that curcumin was presented as the crystalline aggregate in almond gum/PVA/curcumin nanofibers whereas the crystalline peaks of curcumin did not appear in the XRD pattern of almond gum/PVA/curcumin-βCDIC nanofibers. FTIR analysis demonstrated the formation of the complex between curcumin and βCD. Thermogravimetric analysis showed that thermal stability of almond gum/PVA nanofibers was slightly increased after incorporation of curcumin and curcumin-βCDIC into it due to the presence of βCD and curcumin with higher thermal stability. Incorporation of curcumin and curcumin-βCDIC into the almond gum/PVA nanofibers successfully improved the solubility and dissolution of curcumin. Therefore, the enhanced solubility of curcumin will improve its bioavailability and therapeutic potential.
References
- Aggarwal, B.; Sunaram, C.; Malani, V.; Jchikawa, H. Curcumin: The Indian Solid Gold; Springer: New York, 2007
- Jayaprakasha, G.; Rao, L.; Sakariah, K. Antioxidant Activities of Curcumin, Demethoxycurcumin and Bisdemethoxycurcumin. Food Chemistry 2006, 98, 720–724.
- Bengmark, S.;. Curcumin an Atoxic Antioxidant and Natural NFkappaB,cyclooxygenase-2, Lipooxygenase, and Inducible Nitric Oxide Synthase Inhibitor: A Shield against Acute and Chronic Diseases. Journal of Parenteral and Enteral Nutrition 2006, 30, 45–51.
- Wang, Y.; Lu, Z.; Wu, H.; Lv, F. Study on the Antibiotic Activity of Microcapsule Curcumin against Foodborne Pathogens. International Journal of Food Microbiology 2009, 136, 71–74.
- Jurenka, J.;. Anti-Inflammatory Properties of Curcumin, a Major Constituent of Curcuma Longa: A Review of Preclinical and Clinical Research. Alternative Medicine Review 2009, 14, 141–153.
- Villegas, I.; Sanchez-Fidalgo, S.; De La Lastra, C. New Mechanisms and Therapeutic Potential of Curcumin for Colorectal Cancer. Molecular Nutrition & Food Research 2008, 52, 1040–1061.
- Biswas, T.; Mukherjee, B. Plant Medicines of Indian Origin for Wound Healing Activity: A Review. The International Journal of Lower Extremity Wounds 2003, 2, 25–39.
- Gomez-Estaca, J.; Balaguer, M.; Gavara, R.; Hernandez-Munoz, P. Formation of Zein Nanoparticles by Electrohydrodynamic Atomization: Effect of the Main Processing Variables and Suitability for Encapsulating the Food Coloring and Active Ingredient Curcumin. Food Hydrocolloids 2012, 28, 82–91.
- Shaikh, J.; Ankola, D. D.; Beniwal, V.; Singh, D.; Kumar, M. Nanoparticle Encapsulation Improves Oral Bioavailability of Curcumin by at Least 9-Fold When Compared to Curcumin Administered with Piperine as Absorption Enhancer. European Journal of Pharmaceutical Sciences 2009, 37, 223–230.
- Tonnesen, H.;. Solubility, Chemical and Photochemical Stability of Curcumin in Surfactant Solutions E Studies of Curcumin and Curcuminolds, Xxviii. Pharmazie 2002, 57, 820–824.
- Lao, C. D.; Ruffin, M. T.; Normolle, D.; Heath, D. D.; Murray, S. I.; Bailey, J. M.; Boggs, M. E.; Crowell, J.; Rock, C. L.; Brenner, D. E. Dose Escalation of a Curcuminoid Formulation. BMC Complementary and Alternative Medicine 2006, 6, 6–10.
- Hsu, C.; Cheng, A. Clinical Studies with Curcumin. Advances in Experimental Medicine and Biology 2007, 595, 471–480.
- Paramera, E.; Konteles, S.; Karathanos, V. Stability and Release Properties of Curcumin Encapsulated in Saccharomyces Cerevisiae, Beta-Cyclodextrin and Modified Starch. Food Chemistry 2011, 125, 913–922.
- Tang, B.; Ma, L.; Wang, H.; Zhang, G. Study on the Supramolecular Interaction of Curcumin and Beta-Cyclodextrin by Spectrophotometry and Its Analytical Application. Journal of Agricultural and Food Chemistry 2002, 50, 1355–1361.
- Rezaei, A.; Nasirpour, A.; Fathi, M. Application of Cellulosic Nanofibers in Food Science Using Electrospinning and Its Potential Risk. Comprehensive Reviews in Food Science and Food Safety 2015, 14, 269–284.
- Rezaei, A.; Nasirpour, A.; Tavanai, H. Fractionation and Some Physicochemical Properties of Almond Gum (Amygdalus Communis L.) Exudates. Food Hydrocolloids 2016, 60, 461–469.
- Rezaei, A.; Tavanai, H.; Nasirpour, A. Fabrication of Electrospun Almond gum/PVA Nanofibers as a Thermostable Delivery System for Vanillin. International Journal of Biological Macromolecules 2016, 2016, 91, 536–543.
- Mohan, P.; Sreelakshmi, G.; Muraleedharan, C.; Joseph, R. Water Soluble Complexes of Curcumin with Cyclodextrins: Characterization by FT-Raman Spectroscopy. Vibrational Spectroscopy 2012, 62, 77–84.
- Taylor, S.; McDowell, I. Determination of the Curcuminoid Pigments in Turmeric (Curcuma Domestica Val) by Reversed-Phase High-Performance Liquid Chromatography. Chromatographia 1992, 34, 74–77.
- Carvalho, D. M.; Takeuchi, K. P.; Geraldine, R. M.; Moura, C. J.; Torres, M. C. L. Production, Solubility and Antioxidant Activity of Curcumin Nanosuspension. Food Science and Technology (Campinas) 2015, 35, 115–119.
- Suwantong, O.; Opanasopit, P.; Ruktanonchal, U.; Supaphol, P. Electrospun Cellulose Acetate Fiber Mats Containing Curcumin and Release Characteristic of the Herbal Substance. Polymer 2007, 48, 7546–7557.
- Sun, X.; Williams, G.; Hou, X.; Zhu, L. Electrospun Curcumin-Loaded Fibers with Potential Biomedical Applications. Carbohydrate Polymer 2013, 94, 147–153.
- Lin, S.; Kao, Y. Solid Particulates of Drug β-cyclodextrin Inclusion Complexes Directly Prepared by Spray Drying Technique. International Journal of Pharmaceutics 1989, 56, 249–259.
- Guyot, M.; Fawaz, F.; Bildet, J.; Bonini, F.; Lagueny, A. M. Physicochemical Characterization and Dissolution of Norfloxacin/ Cyclodextrin Inclusion Compounds and PEG Solid Dispersions. International Journal of Pharmaceutics 1995, 123, 53–63.
- Yallapua, M.; Jaggi, M.; Chauhana, S. beta-Cyclodextrin-Curcumin Self-Assembly Enhances Curcumin Delivery in Prostate Cancer Cells. Colloids and Surfaces B: Biointerfaces 2010, 79, 113–125.
- Wang, P.; Hu, W.; Su, W. Molecularly Imprinted Poly (Methacrylamide-Co-Methacrylic Acid) Composite Membranes for Recognition of Curcumin. Analytica Chimica Acta 2008, 615, 54–62.
- Giordano, F.; Novak, C.; Moyano, J. Thermal Analysis of Cyclodextrins and Their Inclusion Compounds. Thermochimica Acta 2001, 380, 123–151.
- Mangolim, C. S.; Moriwaki, C.; Nogueira, A. C.; Sato, F.; Baesso, M. L.; Neto, A. M.; Matioli, G. Curcumin–B-Cyclodextrin Inclusion Complex: Stability, Solubility, Characterization by FT-IR, FT-Raman, X-Ray Diffraction and Photoacoustic Spectroscopy, and Food Application. Food Chemistry 2014, 153, 361–370.