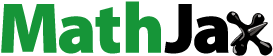
ABSTRACT
Soursop is a tropical fruit that undergoes postharvest deterioration rapidly. Conversion into powder is an ave nue to value-add the fruit as it helps to reduce postharvest losses. Although powder production is not complicated, studies have shown that caking is a common problem often associated with fruit powders. Thus, an addition of a food additive is needed to improve the storage stability of powders. In this study, soursop powder was produced by spray-drying an enzyme-liquefied soursop puree incorporated with either tricalcium phosphate (TCP) or calcium silicate (CS), at three different concentrations (0.5, 1.0, and 1.5% w/w). The control was considered powder without the addition of anticaking agent. Storage stability of the powder packed in aluminum-laminated polyethylene (ALP) pouches was examined at conventional (25 ± 1°C) and accelerated (38 ± 1°C) temperatures for 91 days until lumpiness was observed. Statistical analysis showed that the addition of anticaking agent significantly (p ≤ 0.05) improved the process yield of powder (7.2%). The moisture content, water activity, density, and water solubility index of the powder were significantly affected by storage time, storage temperature, and concentration of the anticaking agents. The critical moisture content, Xc, for control and powder incorporated with either TCP or CS was 0.07 g H2O/g ds. The total color difference (ΔE) of the powder increased throughout the storage period, followed by a zero-order kinetic reaction. Kinetics-derived Arrhenius model showed that the activation energy (Ea) of color change ranged between 6.5 and 17.3 kJ/mol. Scanning electron microscopy showed that the freshly spray-dried powder was composed of spherical particles with smooth surfaces but these particles tended to agglomerate and form liquid bridges after storage for 91 days. Overall, TCP and CS exhibited a protective effect by lowering moisture adsorption and improved the glass transition temperature of the powder.
Introduction
Annona muricata L., commonly known as soursop, is one of the members of Annonaceae family. In recent years, the soursop fruit has received considerable attention from the public because numerous studies had indicated that soursop fruit is nutritionally high in carbohydrates and contains appreciable amounts of micronutrients and minerals.[Citation1] However, a postharvest-challenging feature of this fruit is that it softens and bruises easily, releasing an unpleasant strong smell when it ripens.[Citation2] Consequently, the fruit has a very short shelf life and quickly becomes unacceptable for fresh consumption and further processing. Therefore, before the fruit loses its usefulness, the fruit should be transformed into value-added commercial products such as soursop powder. To achieve this, spray drying may be used to transform liquefied soursop fruit into powder. Application of enzymes during the preparation of soursop puree as spray drying feed can help to improve liquefaction and reduce the viscosity of the puree. This is because a spray drying feed that has low viscosity (less than 250–300 mPas) and a smooth consistency (i.e., free from solid matter) is able to improve atomization efficiency as the feed can be pumped into the drying system easily.[Citation3,Citation4]
Studies on changes in the quality of a food product as a function of time are important to ensure product compliances to food standards. There are several factors that affect a food’s shelf life, such as environmental conditions that the food is exposed to (high or low storage temperature and relative humidity, and the presence of light) and the food packaging properties (permeability of the packaging to oxygen, water vapor, and light).[Citation5,Citation6] Potential modes of degradation of quality (i.e., color changed, increased of moisture) and kinetics of the quality degradation as a function of reaction phase condition are important indicators in the food’s shelf life.[Citation5,Citation6] Kinetics modeling of the quality change during storage describes the reaction rate as a function of storage time, and hence, is able to predict changes in the particular food product during storage.[Citation7,Citation8] The kinetics of quality change in a food product may follow zero-, first-, or second-order reaction.[Citation6] Labuza[Citation6] used the Arrhenius model as an exponential model to assess the dependence of degradation rate constant on temperature and the value of activation energy (Ea) computed from the equation measures the temperature sensitivity of the reaction.
According to the Malaysian Food Law and Regulation, Section 2.11 (1985), dried fruit and vegetable products, including powders, should be dehydrated under natural or artificially induced condition and should not contain more than 8% of water.[Citation9] However, during storage, food powders are subjected to time consolidation effect, a deleterious phenomenon which affects the quality of food powder.[Citation10,Citation11] It describes the progressive compaction of food powder under its own weight over time, leading to caking and, thus, quality degradation.[Citation10] Caking is an irreversible condition due to adsorption of excessive moisture from the surrounding, especially if the powder is amorphous. Chang et al.[Citation12] reported that spray-dried soursop powder is an amorphous powder with a glass transition temperature (Tg) ranging between 46°C and 58°C, indicating the powder has a high tendency to cake over time. According to Aguilera et al.,[Citation10] powder particles may go through four stages of deterioration during storage, namely bridging, agglomeration, compaction, and liquefaction, which eventually lead to caking. Thus, to improve storage stability, anticaking agents are usually added during the production of food powders.[Citation13,Citation14] Barbosa-Cánovas et al.[Citation15] proposed that one of the four ways by which an anticaking agent increases the stability of powders is by increasing the Tg of the powder. The other three ways are (i) competing with the host powder for moisture, (ii) acting as physical barriers between particles, and (iii) acting as moisture-protective barriers. On the other hand, Aguilera et al.[Citation10] suggested that an anticaking agent alters the lattice pattern of the molecular structure of powder, thereby delaying the glassy-rubbery transition phase. Many types of food grade anticaking agents are used in the current industrial practices such as calcium silicate, tricalcium phosphate, silicon dioxide, and calcium stearate.[Citation13,Citation14] However, there is very little information on the protective effect of anticaking agents during storage.
Freshly produced spray-dried soursop powder was found to clump easily (unpublished data), thereby, requiring a further study to improve its storage stability. Thus, the aim of this study was to determine the effects of two types and concentrations of anticaking agents and storage conditions (conventional and accelerated storage) on the stability of spray-dried soursop powder that had been packed in heat-sealed aluminum-laminated polyethylene (ALP) pouches. Many investigations have reported that ALP pouch is a sufficiently good packaging material that retains the highest quality attributes and preserves the product by extending the product’s shelf life especially in dried powder.[Citation7,Citation8,Citation16] The mode of protective effect exhibited by the anticaking agents was also investigated.
Materials and methods
Materials
Soursop fruits at commercial maturity stage (1.2 ± 0.2 kg) were purchased from a soursop plantation located in Simpang Renggam, Johor, Malaysia. Fruits that have been peeled were vacuum-packed and frozen at –20°C until required. Maltodextrin DE 10 (Brand AAA) was a gift from San Soon Seng Food Industries Sdn. Bhd. (Sungai Buloh, Malaysia). Food-grade Pectinase® Ultra SP-L (pectinase) and Celluclast® 1.5 L (cellulase) were purchased from Novozymes, Denmark. Food-grade tricalcium phosphate (TCP) and calcium silicate (CS) were purchased from Spectrum Chemical Mfg Corp, New Jersey, USA. Aluminum-laminated polyethylene (ALP) pouches (110 mm × 130 mm; thickness 10 um) were manually made using ALP sheets constructed from polyethylene terephthalate and linear low-density polyethylene laminated with aluminum which were purchased from Good and Well Sdn. Bhd. (Selangor, Malaysia). Analysis showed that the water vapor permeability of the ALP pouches was 1.19 × 10−7 kg/m2dayPa, measured at room temperature (25 ± 1°C). All chemicals including the salt used to establish salt solutions were analytical grade.
Preparation of spray drying feed
Frozen soursop fruits were thawed at room temperature, and the core was removed. Pectinase at a concentration of 1.5% v/w was added to 100 g of representative samples and incubated in a water bath at 50ºC for 75 min.[Citation17] The resulting puree was filtered through a plastic juice strainer (28 cm × 14.8 cm, mesh size 0.1 cm × 0.1 cm) to remove the seeds. The pectinase-treated puree was added with 1.3% v/w of cellulase and incubated at condition described above for further liquefaction. The puree was collected and pasteurized at 79ºC for 69 s.[Citation18] Prior to spray drying, a total of 100 g of puree was added with 37% w/w maltodextrin. Tricalcium phosphate (TCP) or calcium silicate (CS) at concentrations of 0 (control), 0.5, 1.0, and 1.5 g/100g was added to the spray drying feed. The range of concentrations used was based on the recommendation of the Food and Drug Administration regulation (Part 172, Subpart E), which states that the concentration of food grade anticaking agent in powders should not exceed 2.0% of the weight of food.[Citation19] The mixture was homogenized at 12,000 rpm using a high-speed homogenizer (Heidolph DIAX 900, Schwabach, Germany) for five minutes. The viscosity of the mixture was 17.5 ± 0.1 mPas with a total soluble solid of 23 ± 1°Brix.[Citation17] The mixture was then spray-dried using a mini spray dryer (Model B-290, Büchi, Switzerland) at an inlet temperature of 156°C, drying airflow rate 40 kg/h, and feed flow rate of 2.5 ml/min.[Citation12]
Packaging and storage of soursop powder
Twenty grams of spray-dried soursop powder were placed in separate ALP pouches and heat-sealed at 150 ± 1ºC using a vertical continuous band sealer (GW-FRB-980II, Good and Well, Selangor, Malaysia). The width of the seal was 10 mm, and the sealed pouches were visually inspected to ensure there is no possibility of leakage. The pouches were then placed vertically along a sample holder (a wooden stick, 230 mm) to ensure that all the pouches did not come into contact with each other when exposed to the same environment. The sample holder with the pouches were placed in desiccators (340 mm width × 340 mm length × 270 mm height with 240 mm inner diameter) maintained at 93 ± 2% equilibrium relative humidity (ERH) using saturated potassium nitrate (KNO3) salt solution and monitored using a digital hydrometer (Pro’sKit NT-311, USA). Next, half of the desiccators were placed at room temperature (RT) at 25 ± 1ºC and the rest at elevated temperature (ET) in an incubator (Memmert BE200, Germany) maintained thermostatically at 38 ± 1ºC for 91 days as a preliminary study had shown that soursop powder without treatment (control) experienced formation of lumps after storage of 91 days. Changes in properties of the powder were monitored at a constant interval of seven days.
Assessment of spray-dried soursop powder
Yield
The process yield of spray-dried soursop powder from each treatment was determined by calculating the ratio of total solid content in the powder obtained to the total solid content in the spray drying feed mixture. The process yield was expressed in percentage (%).[Citation12,Citation20]
Moisture content
The moisture content was determined by the vacuum drying method.[Citation12,Citation20,Citation21] One gram of soursop powder was weighed on a pre-dried and pre-weighed glass petri dish and dried at 70ºC using a vacuum oven (VD 23, Binder Gmbh, Germany) until a constant weight was obtained after 24 h. The moisture content of soursop powder was expressed in percentage of dry basis (% db).
Water activity (Aw)
Two grams of soursop powder was weighed into a sample cup and inserted into the pre-calibrated water activity meter (AquaLab 3TE, USA). The water activity of the sample was read at room temperature (25 ± 1°C).[Citation11,Citation22]
Glass transition temperature (Tg)
A differential scanning calorimeter (Perkin Elmer Diamond DSC, Shelton, CT, USA) was equipped with an intracooler refrigeration unit (Perkin Elmer Intracooler 2P), pre-calibrated with indium (melting point = 156.60°C) and zinc (melting point = 419.60°C), was used to determine the glass transition temperature (Tg) of the soursop powder. About 5–10 mg of the powder was placed in an aluminum pan (Perkin Elmer, 0219-0062) and the cover was crimped in place (Perkin Elmer, 0219-0061). The sample was heated from 20ºC to 120ºC for powder stored from Day 0 until Day 42 and from –60ºC to 60ºC for powder stored from Day 49 until Day 91 with a heating rate of 10ºC/min. Nitrogen gas was flushed at a flow rate of 20 ml/min. An empty sealed aluminum pan was used as a reference, and a baseline was constructed using another empty pan under the same temperature range and scanning rate. The thermogram generated was analyzed using the Pyris thermal analysis software (Pyris Manager) and Tg was calculated as half the extrapolated change in specific heat (∆Cp).[Citation4]
Density
An AccuPyc II 1340 pycnometer (Micromeritics Instruments Inc., Norcross, Georgia) was used to determine powder density. Approximately one gram of the soursop powder was filled into a 3.5 cm3 sample module, closed with a filter cap and inserted into the sample chamber and then capped with a cell chamber cap O-ring. The true density was measured by the gas displacement method and expressed as g/cm3 in which the sample cell was filled with helium gas of 19.5 psig.[Citation23]
Color
The color of the spray-dried soursop powder was determined using a Minolta CR-300 colorimeter coupled with a Minolta DP-301 data processor (Konica Minolta, Japan). A handheld chroma meter with a measuring head of 8 mm diameter was connected to the colorimeter with an optical fiber cable. Result was expressed as L* (brightness/darkness), a* (redness/greenness), and b* (yellowness/blueness). The instrument was pre-calibrated with a white standard tile (L* = 91.0, a* = + 0.3165, b* = + 0.3326).[Citation12]
The total color difference ΔE, was calculated between the initially spray-dried powder (Day 0) and the stored soursop powder (Day n) using the following equation (Equation 1):
where ,
, and
are the color parameters of the initial spray-dried powder and
,
, and
are color parameters of the stored powder at Day n where n is the number of storage day.
Kinetic modeling of total color difference (ΔE)
The kinetic reaction of total color difference (ΔE) was evaluated using the kinetic equation (Equation 2) as follows:[Citation5]
where A is ΔE, t is time, k is the kinetic constant, and n is the reaction order. The kinetic change in color of food powder was reported to follow zero-order[Citation7,Citation8] or first-order[Citation16,Citation24] reaction using the following equations:
where C is ΔE, C0 is the initial ΔE, t is storage day (θ), and k0 and k1 are kinetic constants for zero- and first-order, respectively.
Meanwhile, the Arrhenius model (Equation 5) was applied to evaluate the dependency of the rate constant on storage temperature as below:
where k is the rate constant, A is the pre-exponential factor, Ea is the activation energy (kJ/mol), R is the universal gas constant (8.314 J/mol K), and T is the absolute temperature (°K). The Arrhenius equation (Equation 5) is rearranged to allow determination of Ea from the kinetic constants obtained from powder stored at two different temperatures.[Citation25]
Water solubility index (WSI)
The solubility of the powder in water was determined using the method described by Cano-Chauca et al.[Citation26] for mango powder and which was also adopted for acerola pomace powder,[Citation27] papaya powder[Citation28] and melon milk powder.[Citation29] A known weight of soursop powder (2.5 g) was mixed vigorously with 30 ml of distilled water in a 50 ml centrifuge tube using a vortex mixer (ZX4, Velp Scientifica, Italy). The centrifuge tube was incubated in a water bath for 30 min at 37ºC. After incubation, the mixture was centrifuged at 5100 × g for 30 min (Model 4200, Kubota, Tokyo, Japan). The supernatant was decanted into a pre-dried and pre-weight glass petri dish and then dried at 105ºC in a convection oven (ED 23, Binder Gmbh, Germany) until constant weight was obtained after 24 h. The dried residue in the petri dish was the solubilized powder, and the residue weight over the initial powder weight was expressed as water solubility index (WSI).
Surface morphology
The surface morphology of the spray-dried soursop powder was viewed using a Scanning Electron Microscope (SEM). The sample powder was attached to the mounted pin stubs and placed into a chamber of a sputter coater (Bal-Tec SCD 005, Japan). Sputtering of gold on the sample was done under high vacuum condition (0.05 mbar) for 180 s. Scanning electron micrographs were obtained using a Variable Pressure Scanning Electron Microscope (Leo 1455 VP SEM, Germany) equipped with an SE1 detector (general purpose imaging detector). All samples were placed into LEP specimen holder and loaded into the chamber. Then, N2 gas was introduced into the chamber and detector was set at an accelerating voltage EHT (Extra High Tension) of 20kV and at a working distance (WD) of 9 mm. All samples were viewed, and digital images were captured at different magnifications of 100×, 500×, 1000×, and 2000×.[Citation12]
Statistical analysis
Samples were analyzed in triplicate, and the values were averaged and expressed as mean ± SD (standard deviation). One-way analysis of variance (ANOVA) was used to identify the significant differences among the means of various sample treatments. A probability level of p ≤ 0.05 (confident level 95%) was identified as significant, analyzed using Minitab version 16.0 software (Minitab Pty Ltd, Sydney).
Results and discussion
Effect of anticaking agents and storage conditions on process yield of soursop powder
The process yields of soursop powder produced from all treatments were generally more than 68.0%. There was a significant difference (p ≤ 0.05) in process yield between soursop powder treated with an anticaking agent and the control (without the addition of anticaking agent) whereby yield may be improved by up to 7.2% when an anticaking agent is present. There was no significant difference (p > 0.05) for process yield of all the samples treated with anticaking agents, indicating the type and concentration of anticaking agents used did not influence the process yield. The highest process yield was obtained from the spray drying feed treated with 1.5% TCP (73.2 ± 1.2%). In this study, incorporation of either of the anticaking agents (TCP or CS) was within the threshold level advocated by the Food and Drug Administration regulation (Part 172, Subpart E)[Citation19] and resulted in a positive result in the process yield of soursop powder. Jaya and Das[Citation13] reported that the presence of an anticaking agent (TCP) produces a more free-flowing mango powder; thus, improving powder recovery in the cyclone collection vessel.
Effect of anticaking agents and storage conditions on properties of soursop powder
Moisture content
and (A) show the change in moisture content of spray-dried soursop powders stored at RT and ET, respectively. Generally, the moisture content for all spray-dried powders was increased at the end of storage. From the figures, the increase in moisture content was non-linear. There was a lag period, determined as the time taken for the moisture content of powder to start to increase non-linearly, of about 17–21 days before the increase in moisture content became apparent (). Uptake of moisture by the soursop powder may happen by transmission of moisture from a high RH environment through the ALP pouch. However, the degree of increase was dependent on the type and concentration of anticaking agents used ().
Table 1. Lag time, level of increase, and critical moisture content (Xc) in the moisture content of soursop powder stored at RT and ET at the end of storage.
Figure 1. (A) Moisture content, (B) water activity, (C) glass transition temperature and (D) total color difference of soursop fruit powder stored at RT over 91 days.
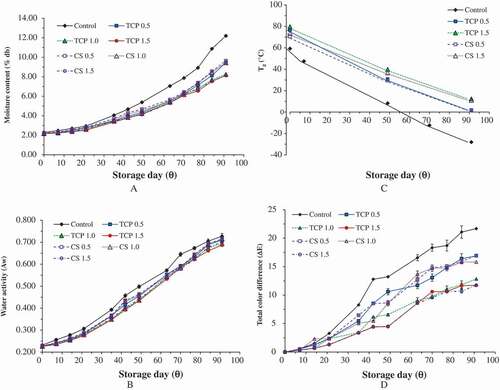
Figure 2. (A) Moisture content, (B) water activity, (C) glass transition temperature and (D) total color difference of soursop fruit powder stored at ET over 91 days.
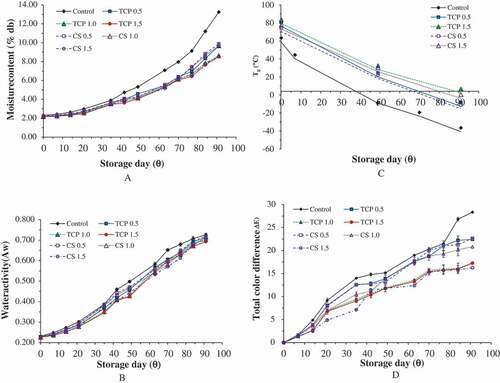
The level of moisture content for control sample increased 4.3–4.8 fold when it was stored at RT and ET, respectively, for 91 days. This value is 171% more than the level of moisture content increase exhibited by powders treated with 1.5% anticaking agents (2.7–3.0 fold). The addition of TCP suppressed the increase in moisture content better than did CS, except when the concentration of TCP was 0.5%. There was no significant difference (p > 0.05) between 1.0% TCP and 1.5% TCP, and thus, the addition of 1.0% TCP may be regarded as optimal. These results suggest that the mode of anticaking agent TCP or CS used to exhibit protective effect is by forming a moisture-protective barrier between the powder particles that prevent adsorption of moisture. Barbosa-Cánovas et al.[Citation15] proposed that anticaking agent acts as a moisture-protective barrier on the surface of the powder by reducing the strength of particles especially the electrostatic forces in order to reduce moisture adsorption.
In this study, the critical moisture content (Xc) where the powder begins to exhibit deterioration reaction such as lumpiness, stickiness, and caking was derived from the intersection point between the change in moisture content of a powder stored at RT and ET and is expressed as g H2O/g dry solid.[Citation30] From , at about 0.07 g H2O/g ds, powders stored at ET began to adsorb more moisture than powders stored at RT. Fabra et al.[Citation30] attributed this change to the rate of sugar dissolution being greater to the rate of moisture adsorption. This may be the case for soursop powder as it is a sugar-rich material. The Xc for control and powder incorporated with either TCP or CS was 0.07 g H2O/g ds ().
Water activity (Aw)
and (B) show the water activity (Aw) of soursop powder obtained from different treatments over the storage period. At both storage temperatures, the increase in Aw for control sample was significantly greater than the Aw for powders incorporated with either 1.0% or 1.5% anticaking agents. Sample stored at ET experienced a slightly higher percentage of increase in Aw than sample stored at RT, especially for the sample treated with TCP. This phenomenon indicates that the soursop powder packed in ALP pouch achieved equilibrium with the surrounding high RH environment (93 ± 2%) in an enclosed system (desiccator). At the end of the storage period, the Aw of most of the powders were not significantly different (p > 0.05) from each other and ranged between 0.69 and 0.73. This range of values signifies that the powders had become relatively unstable at the end of storage as chemical and microbiological reactions may be activated at Aw greater than 0.4.[Citation31] A similar outcome was also reported for coconut powder stored at 90% RH and 38°C as the Aw increased up to 94% over storage for 30 days.[Citation32]
Glass transition temperature
A step change in heat flow was observed in typical DSC thermogram (data not shown) of spray-dried soursop powder without treatment due to a change in heat capacity as the material undergoes a physical transition from glassy state to rubbery state.[Citation33] This indicates the presence of an amorphous structure in all the spray-dried soursop powders. ) shows the initial Tg of spray-dried soursop powders treated with anticaking agents were ranged between 70.5°C and 79.6°C while the Tg for the control sample was 59.3°C. These values indicate the powders are in the glassy state because they exhibited the Tg values (Tg > 25°C) higher than RT. The much lower initial Tg of the control sample was due to the sample possessing a greater level of amorphous properties. Higher Tg values of powder treated with anticaking agents agreed with Barbosa-Cánovas et al.[Citation15] who proposed that anticaking agent increases the stability of powders by increasing the Tg of the product. A similar result was reported by Phanindrakumar et al.[Citation34] that Tg of pineapple powder improved 15% due to the addition of 0.25% TCP. Jaya & Das[Citation35] and Phanindrakumar et al.[Citation34] attributed this phenomenon to the incorporation of high molecular weight anticaking agents such as TCP (MW = 310) and CS (MW = 172) which increased the overall molecular weight of mango and pineapple powder, respectively. In this study, the Tg of both TCP and CS were not able to be determined, probably because they exceeded the maximum temperature that the DSC used could reach. Thus, although the quantity used was only 1.5%, their presence in the powder made a big difference to the Tg value.
At the end of storage day, the recorded Tg was relatively low (Tg < 3°C), indicating these powders were not stable and had a high tendency to undergo a physical transition. Lowering of Tg value may be due to the plasticizing effect of water as water has a Tg of – 135°C[Citation36] which depressed the Tg of the amorphous material.[Citation30] This might be due to adsorption of moisture during storage especially at ET (). It is reported that the accumulation of moisture led to the mobilization of molecules and they exhibited a higher tendency to undergo physical transition.[Citation33,Citation37] Goula et al.[Citation37] and Fabra et al.[Citation30] also reported a stepwise declining trend in Tg for tomato and grapefruit powders, respectively, over storage time. The control experienced the highest degree of Tg reduction (>145%) at the end of storage day at both storage temperatures. This value is 1.4–2 times greater than the percent decreases in samples treated with anticaking agents. At the end of storage, the addition of 1.5% TCP or CS produced a sample that had 10–15°C higher Tg than sample treated with 0.5% concentration at both storage temperatures, indicating better powder stability. In general, storage at ET () led to lower Tg values. This result is expected as the heat applied promotes the physical transformation. This agrees with the finding reported in tomato powder that the lowest Tg (0°C) was recorded at the highest storage temperature (37°C) while the highest Tg (26°C) at the lowest storage temperature (0°C) after 5 months of storage.[Citation38]
Density
The average density of the initial spray-dried soursop powder was 1.44 ± 0.01 g/cm3, indicating the powder was composed of easy flowing particles that possessed a strong cohesive strength. The average density of soursop powders added with anticaking agent TCP, regardless of the concentration, was significantly higher (p ≤ 0.05) than samples treated with CS. This is probably due to the density of TCP being higher than CS, in line with Codex Alimentarius[Citation39] which states that CS (Codex number 552) has a lower bulk density and true density than TCP (Codex number 341 iii).
The density of all the soursop powder was found to increase slightly with storage by up to 6.3% and 6.7% for storage at RT and ET, respectively. This might be due to the distances among the particles which became closer to the domination of van der Waals forces, causing particles that stay in a closed space to become compact. Since density is negatively correlated with volume, when the voids are reduced and the volume occupied by powder becomes smaller, the density is higher.[Citation33] The anticaking properties of TCP and CS were relatively more effective at a concentration of 1.0% (w/w) as they better protect the soursop powder from changing the true density. The percent increase in density for samples stored at ET was slightly higher than the sample stored at RT by up to 1.0%. This may be due to more heat gained by the particles from high storage temperature lead to a closer distance and accelerated the formation of a liquid bridge between the particles due to increase in interparticle forces.[Citation10] Chauhan and Patil[Citation40] also reported that the increase in bulk density caused poor flowability in mango powder that stored higher temperature (21°C) compared to sample stored at low temperature (10°C).
Color
Color of spray-dried powder
The color parameter L*, a*, and b* for spray-dried soursop powder without an anticaking agent (control) was 85.5 ± 0.3, 2.4 ± 0.1, and 17.4 ± 0.2, respectively, indicating the powder has high whiteness. Statistical analysis showed that neither the addition nor the types and concentrations of anticaking agent influenced the color of powder as there were no significant differences (p > 0.05) in the color parameters for the initial spray-dried soursop powder. The color parameters of the soursop powder change following storage (). This is supported by the visually observable gradual change in color of all samples from the initial cream color to light yellow at the end of the storage period, with control being more yellowish () compared to sample treated with CS () and TCP (). shows the color parameter (L*, a*, and b*) of spray-dried soursop powder for the control sample and anticaking agent-incorporated samples before (Day 0) and after (Day 91) the storage at RT and RT. The color changed over the storage was explained using total color difference (ΔE).
Total color difference (ΔE)
and (D) show that after a brief lag period, ΔE increased throughout the storage period, indicating a continuous color change at RT and ET, respectively. At the end of storage day, the lowest ΔE was recorded in 1.5% TCP or CS while the highest ΔE was recorded for control. Color change during storage was observed in the powder may be due to physical and chemical reactions such as the non-enzymatic browning reaction that took place in the soursop powder especially at ET. According to Muralikrishna et al.,[Citation41] factors namely moisture, water activity, sugar content, and storage temperature caused non-enzymatic browning in the stored food product. Similar results were reported for aloe vera powder that decreased in lightness and yellowness over accelerated storage for 49 days.[Citation16]
The control sample experienced 85% more color change compared to the sample treated with 1.5% anticaking agents at the end of storage day at both storage temperatures. Statistical results showed that there was no significant difference (p > 0.05) in ΔE between sample treated with TCP and CS regardless concentrations used at both storage temperatures throughout the storage period, indicating both anticaking agents exhibited similarly protective properties in retaining the color change. This is probably because the anticaking agents protected the color of powder by preventing adsorption of moisture and reduced the chance of non-enzymatic browning. Meanwhile, all samples that were stored at ET had significantly higher (p ≤ 0.05) ΔE than samples stored at RT regardless the concentration used and storage time (). This indicates accelerated storage temperature brought more pronounced color changed in soursop powder.[Citation24] In order to understand the color changed of powder over storage and its dependency on the temperature, kinetics rate reaction and Arrhenius model were employed and the results are discussed below.
Kinetics of color change in spray-dried soursop powder during storage
Data of ΔE for spray-dried soursop powder was substituted in kinetic equation (Equations 3 and 4) and the parameters are presented in . Overall, the R2 values for zero-order kinetic reaction were considered satisfactory as R2 was more than 0.95 for both storage temperatures, indicating ΔE of soursop powder follows zero-order kinetic reaction. Zero-order kinetic reaction in color changed was also reported in mango yogurt powder,[Citation8] jack fruit powder,[Citation24] and pomegranate powder.[Citation7]
Table 2. Kinetic parameters and activation energy of soursop powder stored at RT and ET by fitting into degradation equation.
A positive sign for k0 signifies the ΔE increased positively with storage time.[Citation16] The highest k0 value being recorded for the control at both storage temperatures, signifying more color change took place in the soursop powder without treatment.[Citation7,Citation16] Besides, the kinetic constants for the sample treated with CS were higher than those for TCP when the concentrations used were 0.5% and 1.0% w/w at both storage temperatures. This trend was reversed when the concentration used was 1.5% w/w, indicating CS showed better anticaking properties than TCP when concentration used was high. Moreover, lower kinetic constants were recorded when a higher concentration of TCP and CS was used, indicating they exhibited a better protective effect. Kinetic constants of sample powder stored at ET were higher than the samples stored at RT, indicating that soursop powder experiences more color change when stored at higher temperature due to heat-induced chemical reactions such as melting of sugars.
Application of the Arrhenius equation (Equation 6) to study on the dependency of kinetic constant on temperature showed that the activation energy (Ea) ranged between 6.5 and 17.3 kJ/mol (). The lower the Ea, the greater is the tendency to change in color over time. With the lowest Ea, the control has the greatest tendency to undergo color change over storage time because the energy required to activate the molecules is low. Increasing the concentrations of TCP increased the Ea, indicating color change would not take place easily as more energy would be required for the change to occur. However, this trend was not observed in samples treated with CS as the lowest Ea was recorded for samples added with 1.0% CS. The sample treated with 1.5% CS had the greatest activation energy indicating that at this concentration, CS is the best anticaking agents to use to reduce color change. The deterioration reaction takes place more easily, and the results were consistent with the highest kinetic constant value recorded in control compared to samples treated with anticaking agents ().
Water solubility index (WSI)
The average WSI of soursop powders at the beginning of storage was relatively high (>93%), indicating the powders under study have a high dissolution ability. This may be due to the initial spray-dried powder has a large pore, high porosity with small contact angle which leads to better dissolve ability in water.[Citation42] Overall, a significant reduction (p ≤ 0.05) in WSI was observed over storage time and the percent decreased. This indicates the powder tends to become more compact due to time consolidation effect, leading to a decrease in wettability and solubility. Lump formation due to van der Waals bonding among the particles reduced the dispersibility of the powder and decreased in solubility.[Citation22] At the end of storage, control exhibited the greatest reduction in WSI (>35%) at both storage temperatures. This indicates dissolution would be difficult because the particles tend to adhere and formed scum that was made up a distinct layer that restricted penetration of water and lead to poor wettability and solubility.[Citation43]
Statistical analysis also showed that at both storage temperatures, there was a significant difference (p ≤ 0.05) between samples treated with TCP and CS. The WSI difference may be due to CS having a smaller particle size that leads to better dispersibility. Lipasek et al.[Citation44] correlated the solubility of powder with the particle size and reported that CS has an average particle size of 8.40 ± 0.88 μm which is smaller than TCP (12.83 ± 2.63 μm). Hence, the sample treated with CS was dispersed more readily when contacting with water, which resulted in higher solubility. In general, soursop powders that were treated with anticaking agents regardless the type and concentration exhibited better WSI which was more than 75% at the end of storage, indicating these powders have better rehydration ability (<20% reduction). This signifies the anticaking agents added are effective in protecting the particles from adhering together and, thus delaying the formation of lumps.
Surface morphology
shows the micrograph for soursop powder particles that exhibited the common morphology of an amorphous particle which has a rounded and spherical shape and presents a continuous wall with the absence of surface cracks (A–E).[Citation45] Visually, there was no difference in morphology between the control sample () and the sample treated with 0.5% TCP ( and ) and 0.5% CS ( and ). However, as the amount of anticaking agents used increased, many small particle size particles were found scattered on the micrograph ( and ). This may be due to the addition of anticaking agent improves the dispersibility of the powder by forming small particles surrounding the large particles that constituted a protective barrier to prevent uptake of moisture.[Citation15]
Figure 5a. Scanning electron micrographs of soursop powder obtained from different treatments (A) control, (B) treated with 0.5% TCP, (C) treated with 1.5% TCP, (D) treated with 0.5% CS, and (E) treated with 1.5% CS stored at RT and ET on (A-E) Day 0, (1) 49, and (2) 91 at magnification level of 2000×.
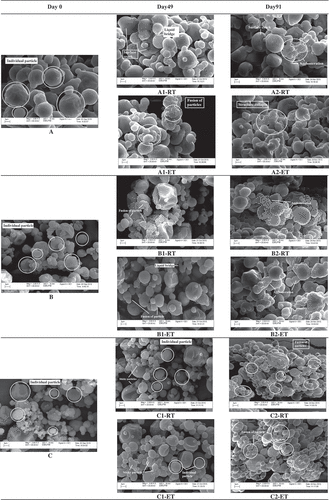
As storage progressed, the particles of control sample were found to stick on the contact point as the edges merged, possibly due to the formation of liquid bridges () when van der Waals force became a dominant attractive force.[Citation10] At the end of storage day (Day 91), liquid bridges brought the particles to become agglomerate particles (). Surface cracking was also observed due to particles indentations caused by stress ().[Citation46] This may be due to adsorption of moisture during storage and causes the particles to mobilize and polarize by the water molecules. Besides, time consolidation effect where the particles in a closed environment become compact, reduced the distances among the particles and led to the fusion of particles and eventually stick together and agglomerated.[Citation38]
The addition of anticaking agents improved the stability of soursop powder as TCP and CS delayed the onset of caking. Most of the particles treated with TCP ( and ) and CS ( and ) were remained round and separated individually on Day 91. A higher degree of particles fusion was noted in samples treated with a lower concentration of TCP (), while more individual particles remained separately in samples treated with higher concentration of TCP (). A similar observation was obtained for samples treated with CS ( and ). Also, after storage for 91 days, lower degree of fusion of sticky particles was observed in all samples treated with anticaking agents as compared to control sample. These observations indicate the anticaking agents exhibited anticaking properties probably by reducing moisture adsorption by the particles.
Besides, surface deformation has become severe for all the samples stored at ET with a higher degree of agglomeration. The particles lost their structural integrity and eventually collapsed at the end of storage day ( and ). Liquid bridges appeared to disintegrate, and particles surfaces deformation was observed because of the particle agglomeration. More formation of clusters was observed in samples added with TCP ( and ) and CS ( and ) regardless of the concentrations used and storage time in samples stored at ET. These observations indicated adsorption of moisture took place at a high rate as more molecules gain energy and were activated when exposed at high temperature. Similar findings were reported by Liu et al.[Citation38] where the caking phenomenon was observed in tomato powder that was stored at a high temperature (37°C) for 5 months.
Conclusion
In this study, soursop powder that is less inclined to caking was produced by spray-dried with the addition of anticaking agents, either tricalcium phosphate (TCP) or calcium silicate (CS). Generally, the addition of an anticaking agent significantly (p ≤ 0.05) improved the process yield of powder by up to 7.2%. Moreover, the stability of powder was improved as a significantly (p ≤ 0.05) lower moisture content, water activity and, density; and a significantly (p ≤ 0.05) higher WSI and Tg were recorded for powders with an anticaking agent. These results suggested that the anticaking agents act as a physical barrier between the powder particles, which prevented adsorption of moisture from the surrounding environment. In addition, the effect of color changed over storage was investigated and the total color difference (ΔE) of soursop powder over storage followed a zero-order kinetic reaction. SEM also showed all the soursop particles exhibited the onset of caking at the end of storage (Day 91). Overall, 1.0% TCP and 1.5% CS were selected as the optimum concentration of anticaking agent that should be used as the storage stability of soursop powder is higher. The outcome of this work shows that the use of anticaking agents to produce soursop powder is effective in preserving the quality of the powder. Studies on the moisture sorption isotherm of soursop to understand the behavior of the powder and applications of soursop powder as a functional ingredient in food industry such as bakery or beverage will be carried out.
This work offers an insight into how anticaking agents affect the stability of powder.
The protection mode showed by anticaking agents is by reducing moisture absorption.
The morphology structure showed anticaking agents delayed the onset of caking.
The critical moisture content (Xc) of soursop powder is 0.07 g H2O/g ds.
The total color difference (ΔE) followed a zero order kinetic reaction.
Additional information
Funding
References
- de Souza, V.R.; Pereira, P.A.P.; Queiroz, F.; Borges, S.V.; de Deus Souza Carneiro, J. Determination of Bioactive Compounds, Antioxidant Activity and Chemical Composition of Cerrado Brazilian Fruits. Food Chemistry 2012, 134, 381–386. DOI: 10.1016/j.foodchem.2012.02.191.
- Espinosa, I.; Ortiz, R.I.; Tovar, B.; Mata, M.; Montalvo, E. Physiological and Physicochemical Behavior of Soursop Fruits Refrigerated with 1-Methylcyclopropene. Journal of Food Qualitative 2013, 36, 10–20. DOI: 10.1111/jfq.2013.36.issue-1.
- APV Dryer Handbook. APV Limited. 2000. [cited 2016 March 8]. Retrieved from https://userpages.umbc.edu/~dfrey1/ench445/apv_dryer.pdf.
- Grabowski, J.A.; Truong, V.D.; Daubert, C.R. Spray-Drying of Amylase Hydrolyzed Sweetpotato Puree and Physicochemical Properties of Powder. Journal of Food Science 2006, 71, E209–217. DOI: 10.1111/j.1750-3841.2006.00036.x.
- Kilcast, D.; Subramaniam, P. Food and Beverage Stability and Shelf Life; Woodhead Publishing Limited: Cambridge, 2011.
- Labuza, T.P. Application of Chemical Kinetics to Deterioration of Foods. Journal of Chemical Education 1984, 61, 348. DOI: 10.1021/ed061p348.
- Dak, M.; Sagar, V.R.; Jha, S.K. Shelf-Life and Kinetics of Quality Change of Dried Pomegranate Arils in Flexible Packaging. Food Packaging and Shelf Life 2014, 2, 1–6. DOI: 10.1016/j.fpsl.2014.04.005.
- Kumar, P.; Mishra, H. Storage Stability of Mango Soy Fortified Yoghurt Powder in Two Different Packaging Materials: HDPP and ALP. Journal of Food Engineering 2004, 65, 569–576. DOI: 10.1016/j.jfoodeng.2004.02.022.
- Malaysian Food Law and Regulation, Food Act 1983 (Act 281) and Regulations 1983; International Law Book Services: Malaysia, 2009.
- Aguilera, J.M.; Delvalle, J.M.; Karel, M. Caking Phenomena in Amorphous Food Powders. Trends in Food Science & Technology 1995, 6, 149–155. DOI: 10.1016/S0924-2244(00)89023-8.
- Hartmann, M.; Palzer, S. Caking of Amorphous Powders — Material Aspects, Modelling and Applications. Powder Technology 2011, 206, 112–121. DOI: 10.1016/j.powtec.2010.04.014.
- Chang, L.S.; Karim, R.; Abdulkarim, S.M.; Ghazali, H.M. Production and Characterization of Enzyme‐Treated Spray‐Dried Soursop (Annona Muricata L.) Powder. Journal of Food Process Engineering. 2018, e12688. DOI: 10.1111/jfpe.12688.
- Jaya, S.; Das, H. Accelerated Storage, Shelf Life and Color of Mango Powder. Journal of Food Processing and Preservation 2005, 29, 45–62. DOI: 10.1111/j.1745-4549.2005.00012.x.
- Lipasek, R.A.; Ortiz, J.C.; Taylor, L.S.; Mauer, L.J. Effects of Anticaking Agents and Storage Conditions on the Moisture Sorption, Caking, and Flowability of Deliquescent Ingredients. Food Research International 2012, 45, 369–380. DOI: 10.1016/j.foodres.2011.10.037.
- Barbosa-Cánovas, G.V.; Ortega-Ricas, E.; Juliano, P.; Yan, H. Food Powders: Physical Properties, Processing, and Functionality; Kluwer Academic: Plenu, New York, 2005.
- Ramachandra, C.T.; Rao, P.S. Shelf-Life and Colour Change Kinetics of Aloe Vera Gel Powder under Accelerated Storage in Three Different Packaging Materials. Journal of Food Science and Technology 2013, 50, 747–754. DOI: 10.1007/s13197-011-0398-9.
- Chang, L.S.; Karim, R.; Mohammed, A.S.; Ghazali, H.M. Characterization of Enzyme-Liquefied Soursop (Annona Muricata L.) Puree. LWT - Food Sciences Technological 2018, 94, 40–49. DOI: 10.1016/j.lwt.2018.04.027.
- Umme, A.; Bambang, S.S.; Salmah, Y.; Jamilah, B. Effect of Pasteurisation on Sensory Quality of Natural Soursop Puree under Different Storage Conditions. Food Chemistry 2001, 75, 293–301. DOI: 10.1016/S0308-8146(01)00151-0.
- Food and Drug Administration. Food Additives Permitted for Direct Addition to Food for Human Consumption. 2016. https://www.accessdata.fda.gov/scripts/cdrh/cfdocs/cfcfr/CFRSearch.cfm?CFRPart=172&showFR=1&subpartNode=21:3.0.1.1.3.5 (accessed May 11, 2016).
- Shavakhi, F.; Boo, H.C.; Osman, A.; Ghazali, H.M. Effects of Enzymatic Liquefaction, Maltodextrin Concentration, and Spray-Dryer Air Inlet Temperature on Pumpkin Powder Characteristics. Food Bioprocess Technological 2012, 5, 2837–2847. DOI: 10.1007/s11947-011-0686-4.
- Ferrari, C.C.; Germer, S.P.M.; de Aguirre, J.M. Effects of Spray-Drying Conditions on the Physicochemical Properties of Blackberry Powder. Drying Technology 2012, 30, 154–163. DOI: 10.1080/07373937.2011.628429.
- Adhikari, B.; Howes, T.; Wood, B.J.; Bhandari, B.R. The Effect of Low Molecular Weight Surfactants and Proteins on Surface Stickiness of Sucrose during Powder Formation through Spray Drying. Journal of Food Engineering 2009, 94, 135–143. DOI: 10.1016/j.jfoodeng.2009.01.022.
- Thomas, A.; Saleh, K.; Guigon, P.; Czechowski, C. Characterisation of Electrostatic Properties of Powder Coatings in Relation with Their Industrial Application. Powder Technology 2009, 190, 230–235. DOI: 10.1016/j.powtec.2008.04.072.
- Pua, C.K.; Hamid, N.S.A.; Tan, C.P.; Mirhosseini, H.; Rahman, R.A.; Rusul, G. Storage Stability of Jackfruit (Artocarpus Heterophyllus) Powder Packaged in Aluminium Laminated Polyethylene and Metallized Co-Extruded Biaxially Oriented Polypropylene during Storage. Journal of Food Engineering 2008, 89, 419–428. DOI: 10.1016/j.jfoodeng.2008.05.023.
- House, J.E. Principles of Chemical Kinetics, 2nd ed.; Academic Press: USA, 2007.
- Cano-Chauca, M.; Stringheta, P.C.; Ramos, A.M.; Cal-Vidal, J. Effect of the Carriers on the Microstructure of Mango Powder Obtained by Spray Drying and Its Functional Characterization. Innovative Food Science and Emerging Technologies 2005, 6, 420–428. DOI: 10.1016/j.ifset.2005.05.003.
- Moreira, G.É.G.; Maia Costa, M.G.; de Souza, A.C.R.; de Brito, E.S.; de Medeiros, M.D.F.D.; de Azeredo, H.M.C. Physical Properties of Spray Dried Acerola Pomace Extract as Affected by Temperature and Drying Aids. LWT- Food Science and Technology 2009, 42, 641–645. DOI: 10.1016/j.lwt.2008.07.008.
- Wong, C.W.; Lim, W.T. Storage Stability of Spray-Dried Papaya (Carica Papaya L.) Powder Packaged in Aluminium Laminated Polyethylene (ALP) and Polyethylene Terephthalate (PET). International Food Research Journal 2016, 23, 1887–1894.
- Zungur Bastıoğlu, A.; Tomruk, D.; Koç, M.; Ertekin, F.K. Spray Dried Melon Seed Milk Powder: Physical, Rheological and Sensory Properties. Journal of Food Science and Technology 2016, 53, 2396–2404. DOI: 10.1007/s13197-016-2214-z.
- Fabra, M.J.; Talens, P.; Moraga, G.; Martínez-Navarrete, N. Sorption Isotherm and State Diagram of Grapefruit as a Tool to Improve Product Processing and Stability. Journal of Food Engineering 2009, 93, 52–58. DOI: 10.1016/j.jfoodeng.2008.12.029.
- Chen, X.D.; Mujumdar, A.S. Drying Technologies in Food Processing; Blackwell Publishing Limited: West Sussex, 2008.
- Jena, S.; Das, H. Shelf Life Prediction of Aluminum Foil Laminated Polyethylene Packed Vacuum Dried Coconut Milk Powder. Journal of Food Engineering 2012, 108, 135–142. DOI: 10.1016/j.jfoodeng.2011.06.036.
- Fitzpatrick, J.J.; Hodnett, M.; Twomey, M.; Cerqueira, P.S.M.; O’Flynn, J.; Roos, Y.H. Glass Transition and the Flowability and Caking of Powders Containing Amorphous Lactose. Powder Technology 2007, 178, 119–128. DOI: 10.1016/j.powtec.2007.04.017.
- Phanindrakumar, H.S.; Radhakrishna, K.; Mahesh, S.; Jagannath, J.H.; Bawa, A.S. Effect of Pretreatments and Additives on the Thermal Behavior and Hygroscopicity of Freeze-Dried Pineapple Juice Powder. Journal of Food Processing and Preservation 2005, 29, 307. DOI: 10.1111/j.1745-4549.2005.00030.x.
- Jaya, S.; Das, H. Glass Transition and Sticky Point Temperatures and Stability/Mobility Diagram of Fruit Powders. Food Bioprocess Technological 2009, 2, 89–95. DOI: 10.1007/s11947-007-0047-5.
- Roos, Y. Melting and Glass Transitions Weight Carbohydrates of Low Molecular. Carbohydrate Research 1993, 238, 39–48. DOI: 10.1016/0008-6215(93)87004-C.
- Goula, A.M.; Karapantsios, T.D.; Achilias, D.S.; Adamopoulos, K.G. Water Sorption Isotherms and Glass Transition Temperature of Spray Dried Tomato Pulp. Journal of Food Engineering 2008, 85, 73–83. DOI: 10.1016/j.jfoodeng.2007.07.015.
- Liu, F.; Cao, X.; Wang, H.; Liao, X. Changes of Tomato Powder Qualities during Storage. Powder Technology 2010, 204, 159–166. DOI: 10.1016/j.powtec.2010.08.002.
- Food and Agriculture Organization. Codex Alimentarius: Food Additive. 2016. http://www.fao.org/gsfaonline/additives/results.html?techFunction=2&searchBy=tf (accessed Jan 8, 2016).
- Chauhan, A.K.; Patil, V. Effect of Packaging Material on Storage Ability of Mango Milk Powder and the Quality of Reconstituted Mango Milk Drink. Powder Technology 2013, 239, 86–93. DOI: 10.1016/j.powtec.2013.01.055.
- Muralikrishna, M.; Nanjundaswamy, A.M.; Siddappa, S.G. Guava Powder Preparation, Packaging and Storage Studies. Journal of Food Science and Technology 1969, 6, 93–98.
- Hogekamp, S.; Schubert, H. Rehydration of Food Powders. Food Science and Technology International 2003, 9, 223–235. DOI: 10.1177/1082013203034938.
- Fang, Y.; Selomulya, C.; Chen, X.D. On Measurement of Food Powder Reconstitution Properties. Drying Technology 2007, 26, 3–14. DOI: 10.1080/07373930701780928.
- Lipasek, R.A.; Taylor, L.S.; Mauer, L.J. Effects of Anticaking Agents and Relative Humidity on the Physical and Chemical Stability of Powdered Vitamin C. Journal of Food Science 2011, 76, 1062–1074. DOI: 10.1111/j.1750-3841.2011.02333.x.
- Koc, B.; Yilmazer, M.S.; Balkır, P.; Ertekin, F.K. Spray Drying of Yogurt: Optimization of Process Conditions for Improving Viability and Other Quality Attributes. Drying Technology 2010, 28, 495–507. DOI: 10.1080/07373931003613809.
- Cai, Y.Z.; Corke, H. Production and Properties of Spray-Dried Amaranthus Betacyanin Pigments. Journal of Food Science 2000, 65, 1248–1252. DOI: 10.1111/j.1365-2621.2000.tb10273.x.