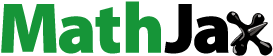
ABSTRACT
Hairtail fish samples were frozen at −3℃, −18℃, −40℃, and −80℃, respectively, followed by different numbers of freeze–thaw cycle treatment. In order to evaluate quality changes associated with microstructure and biochemical changes of hairtail samples, fractal dimension was applied to observe porous microstructure due to the formation of ice crystals in frozen hairtail meat, as well as selected quality parameters were determined. Results showed that fractal dimension presented a decreasing trend with increasing numbers of freeze–thaw cycles from the initial value of 1.9679 to 1.3867, 1.5652, 1.5955, and 1.7445 for −3℃, −18℃, −40℃, and −80℃ freezing temperatures, respectively. Increased numbers of freeze–thaw cycles were accompanied by decrease in water holding capacity, increase in microbiological counts, and a greater degree of protein and lipid oxidation, as evidenced by higher contents of total volatile basic nitrogen and the thiobarbituric acid-reactive substances value. The rates of these parameters decreased with the decrease in freezing temperature. Besides, the relationships were analysed between fractal dimension, and these traditional quality indicators and the determined correlations were within ±0.90. The results demonstrated that fractal dimension proved to be a novel and feasible method to evaluate the quality of hairtails in freezing–thawing processes.
Introduction
Hairtail (Trichiurus Haumela), also called cutlassfish or ribbonfish, is one of the most popular commercial marine fish species in the eastern Pacific Ocean. Both economically and ecologically, the hairtail plays an important role in supporting the most valuable and largest fishery in Asia with its abundant fish stocks.[Citation1,Citation2] In recent years, the production and consumption of hairtail is growing due to its delicious taste and high nutritional value. However, hairtails are vulnerable to perish even more severe than other marine fish species because of the presence of highly unsaturated fatty acids, where colour, flavour, texture, and nutritional value can be negatively affected.[Citation3,Citation4]
Freezing is one of the most preferred technique for aquatic food preservation and is widely used from domestic to industrial scale. When compared to other preservation methods, freezing is much more popular because the decrease in enzymatic and microbial activity is acquired without the application of heating or preservatives.[Citation5] Despite its ability to retain meat quality and safety, issues relating to freeze–thaw cycles remain a major concern for processors and consumers. Repeated freezing–thawing is inevitable and common in both restaurants and retail outlets, and even in domestic kitchens, and can also occur during transportation or storage.[Citation6,Citation7] Temperature fluctuations attributed to freeze–thaw cycles, being a core issue in the cold chain of frozen food, caused undesirable deteriorative changes on food quality, including lipid oxidation, water loss, and undesirable flavour and texture of frozen food.[Citation8–Citation10] An understanding of the quality changes due to freeze–thaw cycles in hairtails is essential to the fish industry, as their main objective is to produce superior products with high resale values that are both appealing and enjoyable to the customer.[Citation11]
Fractal dimension, proposed by Mandelbrot, is a parameter in the geometry characterized as the space-filling capacity of a space structure at a certain scale. Recently, fractal dimension was used to quantify the palm oil crystal networks by image analysis and rheological measurements by Omar.[Citation12] Joardder[Citation13] analysed the correlation between microstructure and porosity through the fractal dimension of dried foods. Chen[Citation14] established a fractal dimension model to analyse beef marbling standards images in China and the USA. During frozen storage of fish, ice crystallization and tissue dehydration can cause a honeycomb microstructure in the muscle tissue, which is a key factor influencing the final quality of frozen food. For fish under multiple freeze–thaw cycles, the microstructure analysis based on fractal dimension has not yet been reported earlier.
Therefore, this paper aimed to evaluate quality changes based on fractal dimension and biochemical changes for hairtail samples under multiple freeze–thaw cycles. Fractal dimension was employed to describe the porous microstructure of frozen hairtails. Its correlation with traditional quality indicators including water holding capacity, total volatile basic nitrogen (TVB-N), thiobarbituric acid-reactive substances (TBARS) value, and total plate count was also investigated.
Materials and methods
Chemicals
Optimum cutting temperature (OCT) embedding agent (ethanol) and hematoxylin and eosin (H&E) were bought from Sigma Chemical Co. (St. Louis, MO, USA). Dry ice was provided by Hangzhou Jinggong Machinery Co. Ltd. (Hangzhou, Zhejiang, China). All chemicals used in this study were of analytical reagent grade.
Sample preparation
Fresh hairtails (length 70.0 ± 2.0 cm) were purchased from a local aquatic market (Zhoushan, Zhejiang, China) and were immediately transported to the laboratory in an icebox. The hairtail fishes were de-headed, gutted, and tails cut, followed by washing with sterile ice-cold water. Then the fish were drained and cut into blocks of approximately 6.0 cm in length. Subsequently, all the fish blocks were sealed in the polyethylene bags and kept in the refrigerators at temperatures of −3 ± 0.2℃, −18 ± 1.5℃, −40 ± 2.0℃, and −80 ± 2.0℃, respectively. After 4 days of storage, all the samples were thawed by running tap water at approximately 10℃ until the core temperature reached 0–2℃, for approximately 10 min. A quarter of samples for each treatment were randomly selected for analysis. The remaining thawed samples were again frozen at the same temperature for another 4 days as described above. The freeze–thaw cycle, freezing at different temperatures for 4 days followed by thawing in aseptic water, was repeated four times.
Water holding capacity
To determine the water holding capacity of frozen hairtail samples, liquid loss was firstly estimated on minced hairtail muscle by low-speed centrifugation.[Citation15] Liquid loss was calculated as the percentage of weight loss of the mince during centrifugation of 5 g of sample for 3 min (1500 rpm). Water content in the hairtail mince was determined by drying minced hairtail sample of 3 g using a halogen water-determination meter (DHS-20A, Shanghai Jicheng analytical instruments co., Ltd., China). The analyses were run in triplicates.
Total volatile base nitrogen
TVB-N was estimated in accordance with the method of Hongbo[Citation16] The analysis included a perchloric acid extraction followed by alkalinization and steam distillation. Five grams of fish fillet was mixed with 45 mL of perchloric acid, homogenized for 2 min at 15,000 rpm. Then the solution was centrifuged for 10 min with the revolving speed of 4000 rpm. After that, the supernatant was stored at 2−6℃. Total volatile bases were finally titrated with hydrochloric acid in a boric acid solution using a Kjeldahl distillation unit (K9860, Jinan Hanon Co. Ltd., China). The TVB-N value was estimated by the following equation:
where V1 is hydrochloric acid volume consumption of sample, V2 is hydrochloric acid volume consumption of blank, C is concentration of hydrochloric acid (0.1 M), and 14 is nitrogen equivalent quality of 1.00 mL standard hydrochloric acid (1.0 M).
Thiobarbituric acid-reactive substances
The determination of TBARS was performed according to the previous method[Citation17] with slight modifications. Ten grams of hairtail fillet muscle was shredded and then mixed with 50 mL of trichloroacetic acid (0.1% EDTA) for 30 s to produce a homogenous output. After filtration, the solution was centrifuged for 4 min with the revolving speed of 4000 rpm by centrifugation. The mixture of 5 mL of supernatant and 5 mL of TBA (0.2 M) was heated in a boiling water bath (100℃) for 30 min. After the sample was cooled, 5 mL chloroform was added (1:1 ratio, v/v), vortexed, and subsequently stratified. The absorbance of the supernatant was determined at the wavelength of 532 and 600nm. The TBARS value, which is expressed as mg of malonaldehyde (MDA)/100g muscle sample, was estimated using the following equation:
Total plate count
Total plate count determination was performed following the method of Xuan[Citation18] with slight modification. A 25 g sample of hairtail meat was obtained aseptically and homogenized with 225 mL of 0.9% physiological saline. The homogenates and serial 10-fold dilution in sterile 0.9% physiological saline were mixed with agar medium and then poured into Petri dishes for microbial analyses. The total plate count, which is expressed as lgCFU/g, was determined after incubation at 37℃ for 48 h.
Microscopic tissue imaging
Frozen stored hairtail samples were used without thawing, four pieces (approximately 5 mm in length) were cut from the centre, transversally to the muscle fibre, using a blade previously cooled to −20℃. The whole operation was carried out in a walk-in freezer to ensure a perfect cold chain. Cryosection was obtained according to the previous method reported in the literature[Citation19] with some modifications. The frozen pieces were then embedded in OCT embedding compound and submerged into dry ice until completely frozen. Each embedded sample was mounted on a chuck and placed in a CRYOSTAR NX50 (Thermo Scientific, USA) previously cooled to −20°C. The surface of hairtail specimen was trimmed and subsequently covered with an adhesive film (Cryofilm type 2C(9), Section-Lab Co, Ltd., Japan) for the purpose of supporting the frozen sections.[Citation20,Citation21] A section of 14 µm thickness was carefully cut, fixed in 70% ethanol for 2 min, and then stained with H&E for later microscopic analysis. All the prepared sections were observed using a microscope (UPH203i, Chongqing UOP Optoelectronic Technology Co., Ltd, China) fitted with a digital camera (JFMV-M1200C, Nanjing Yifei Technology Co., Ltd, China) at a magnification of 200× .
Fractal dimension analysis
Fractal dimension values were obtained by the box-counting method[Citation22] through MATLAB software. The microscopic color image was firstly converted into grey scale image, and processed with binarization. The binary image was divided into square sub-boxes with a variable length denoted as ε. In the divided images, the sub-box filled by more than 50% red tissues was considered as valid. Under sections with different lengths ε, the number of the valid boxes was marked by N (ε). Plotting lg N (ε) with lg ε, the fractal dimension d of the image would be obtained through the slope of regression line.
Statistical analyses
All measurements were carried out in triplicates, and data were expressed as mean ± standard deviation. The t-test of SPSS 19 was used to test the significant differences of the means of evaluation parameters with a value of p < 0.05 being regarded as statistically significant.
Results and discussion
Assay of water holding capacity
The ability to retain water in the muscle is regarded as a critical quality parameter, and a higher water holding capacity is beneficial both to the industry and the consumers, because it has strong influence on the weight change during storage and transport, drip loss after thawing, shrinkage, and weight loss during cooking; and the tenderness and juiciness of the food.[Citation23] shows the variations in the water holding capacity of hairtails frozen at different temperatures with different freeze–thaw cycles. The initial value was 88.75%. With the increase in the number of freeze–thaw cycles, the value of water holding capacity displayed a decreasing trend. After four freeze–thaw cycles, the value decreased by 19.70%, 17.50%, 15.36%, and 12.11% in the samples that were frozen at −3℃, −18℃, −40℃, and −80℃, respectively. It was found that the decreasing rate of water holding capacity varied depending on different freezing temperatures. The higher freezing temperature, a more significant decrease was found. The data showed that the hairtail samples frozen at −3℃ had the biggest decreasing rate of water holding capacity values.
Figure 1. Effect of freeze–thaw cycles on water holding capacity in hairtail (Trichiurus haumela) samples frozen at −3℃ (◇), −18℃ (■), −40℃ (▲), and −80℃ (○). The error bars indicate the standard deviation obtained from a total of three analysis.
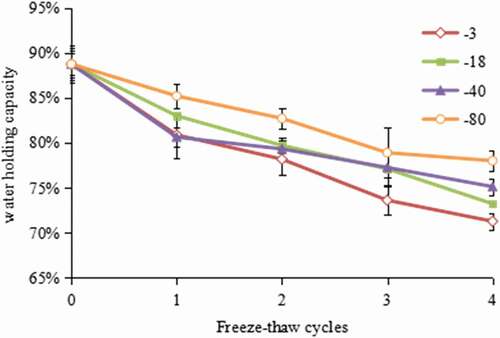
A similar phenomenon of water holding capacity variation in fish preservation was found by Ali,[Citation6] who observed that the water holding capacity of chicken breast muscle decreased from 58.8% to 50% as the number of freeze–thaw cycles increased. According to Offer,[Citation24] decreased water holding capacity of fish muscle has often been considered as an effect of structural changes, which mainly result from myosin denaturation, the shrinkage of myofilament lattice, and increased extracellular space. During freeze–thaw cycles, fluids migration and repeated ice crystallization and melting would impart mechanical damage to the muscle fibre and destroy the integrity of the muscle, which may result in an increase in a reduction of the water holding capacity and other quality indexes associated with the damage of the cell structure. Meanwhile, the changes resulted in the development of honeycomb microstructure in muscle tissues.[Citation25] Different variation rates between temperatures may be attributed to smaller ice crystals formed at a lower temperature which caused less damage on the tissue and a lower decreasing rate of water holding capacity.[Citation26]
Assay of TVBN
As an important indicator of fish spoilage, TVB-N is widely used in evaluating fish quality.[Citation27] The changes of TVB-N values in hairtail during multiple freeze–thaw cycles are illustrated in . At cycle 0, TVB-N values of all groups were 14.3 mg N/100 g, which was higher than those reported by Khalafalla.[Citation28] These differences could be attributed to the effect by species, catching season and region, age, and sex of the fish. After four cycles of freeze–thawing, the TVB-N value in hairtails steadily increased to 30.7, 25.6, 26.6, and 24.6 mg/100 g in the samples that were frozen at −3℃, −18℃, −40℃, and −80℃, respectively. According to the current hygienic standards, a level of 30 mg TVB-N/100g in the fish sample is recognized as a spoilage limit.[Citation28] When subjected to four freeze–thaw cycles, the value of samples frozen at −3℃ reached the value for decomposition. Whereas other groups remained acceptable for consumption throughout the four freeze–thaw cycles.
Figure 2. Effect of freeze–thaw cycles on TVBN value in hairtail (Trichiurus haumela) samples frozen at −3℃ (◇), −18℃ (■), −40℃ (▲). and −80℃ (○). The error bars indicate the standard deviation obtained from a total of three analysis.
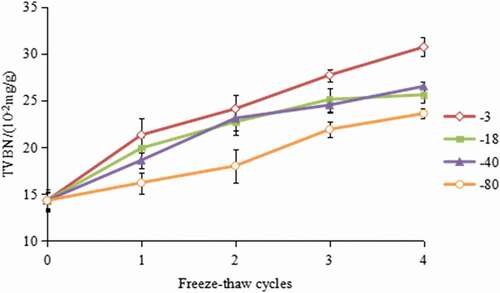
The results were in agreement with the findings of Leygonie,[Citation11] who reported that repeated freezing and thawing cause damage to the ultrastructure of the muscle cells with the ensuing release of mitochondrial and lysosomal enzymes, haem iron, and other pro-oxidants. These increase the degree and rate of protein oxidation. The reaction accumulated the dimethylamine and trimethylamine, leading to a rise in TVB-N value accordingly. The TVB-N values for hairtails frozen at −3℃ increased rapidly, while for samples frozen at −80℃, the increase rate is lower, which reflected that lower storage temperatures could inhibit the microbial and enzymatic reaction, thus inhibiting TVB-N formation.
Assay of TBARS
The hairtail meat in this study is rich in protein and fat, especially unsaturated fatty acids, which is susceptible to the pro-oxidants and oxygen attack and form hydroperoxides that easily decompose to secondary products, including MDA. Thus, TBARS value was commonly used to judge the content of secondary peroxidation product malondialdehyde and the degree of fat oxidation.[Citation29]
As shown in , the initial TBARS value of hairtail fish was 0.232 mg/100 g. After subjection to four freeze–thaw cycles, TBARS was increased to 0.505 mg/100 g in −3℃ group, 0.388 mg/100 g in −18℃ group, 0.365 mg/100 g in −40℃ group, and 0.265 mg/100 g in −80℃ group, respectively. Similar trends were found by Benjakul[Citation30] who found that freezing and thawing of muscle tissue resulted in accelerated TBARS accumulation and attributed this finding to the damage of cell membranes by ice crystals and the subsequent release of pro-oxidants, especially the haem iron. Compared with the samples frozen at −3℃, the TBARS in the −80℃ frozen samples was significantly reduced with the decrease in freezing temperature, indicating that a low temperature can significantly decrease the formation of MDA and other secondary products. This might be arising from the fact that smaller ice crystals formed under lower freezing temperature caused less damage to cell membranes, resulting in less exposure to oxygen and less lipid oxidation.
Assay of total plate count
The results of microbiological analysis are shown in . The total plate count showed similar trends as that of TVBN and TBARS value when treated with four freeze–thaw cycles. At the beginning of the storage, total plate values for fresh hairtail samples were 2.50 log CFU/g samples. For samples frozen at −3℃, total plate count increased to 6.51 log CFU/g and exhibited the highest bacterial count as the freeze–thaw cycles increased, suggesting that higher temperature freezing can significantly activate the growth of microorganisms. According to the proposed limits (7 log CFU/g) for fresh fish by ICMSF (1986), all treatments maintained relatively good quality throughout the four freeze–thaw cycles as the total plate count did not exceed the limitation.
Figure 4. Effect of freeze–thaw cycles on total plate count in hairtail (Trichiurus haumela) samples frozen at −3℃ (◇), −18℃ (■), −40℃ (▲), and −80℃ (○). The error bars indicate the standard deviation obtained from a total of three analysis.
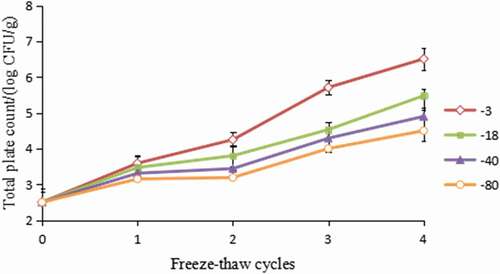
It is well known that during freezing, microbial spoilage is effectively terminated as the microbes become dormant. However, they retain their activity during thawing.[Citation11] As thawing is a much slower process than freezing and is less uniform, certain areas of the hairtail meat will be exposed to more favourable temperature conditions for microbial growth, particularly when air thawing is employed. In addition to the risk of high temperature exposure, there is an increase in moisture and nutrients available to microbe post freeze/thaw due to exudate formation. The moisture lost during thawing is rich in proteins, vitamins, and minerals derived from structural disarray caused by the freezing process, which consequently provides an excellent medium for microbial growth.
Assay of the tissue structure and the fractal dimension
shows the tissue structure of hairtails subjected to different freeze–thaw cycles. The microstructure in the images of hairtail muscle comprises two parts, the red muscle tissues stained with H&E and the white space caused by ice crystals. Frozen hairtail muscle was compared with unfrozen tissue as a control. The cross section of control sample had a uniform distribution of regularly shaped fibres. The hairtail muscle fibres showed different degrees of departure from uniformity due to the ice crystal formation as the number of freeze–thaw cycles increased. In , all the groups including −3℃, −18℃, −40℃, and −80℃ freezing show an increase of void spaces inside the frozen tissue as the number of freeze–thaw cycles increased, compared to the initial samples. According to Sriket,[Citation25] it was observed that shrinkage of fibres and gaping was formed in the microstructures of white shrimp and black tiger shrimp subjected to five freeze–thaw cycles. Protein denaturation and disruption of endomysium induced by freeze–thawing possibly resulted in a less compact structure. The disrupted structure and larger irregular pores left by ice crystals with the increase in the number of freeze–thaw cycles might be attributed to fluid migration caused by ice crystallization, and muscle fibre disarrangement caused by protein decomposition.[Citation31]
Figure 5. Effect of freeze–thaw cycles on the microstructure in hairtail (Trichiurus haumela) samples frozen at −3℃, −18℃, −40℃, and −80℃. (1, 2, 3, and 4 represent different numbers of freeze–thaw cycles).
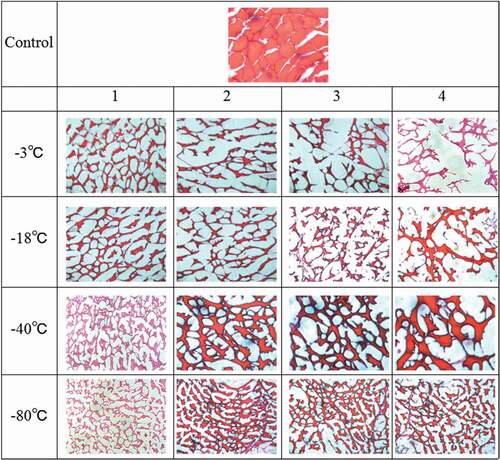
Moreover, large and irregular ice crystals were observed in micrographs of the meat from hairtails frozen at −3℃ in . Clearly, most area was occupied with the cross section of the ice crystals larger than the muscle fibres at four freeze–thaw cycles, which implies that the muscle tissue of hairtails was seriously deformed. The voids left by ice crystals in at −80℃ were still relatively small after four freeze–thaw cycles. Smaller and more regular ice crystals tended to form as the freezing temperature decreased. Therefore, low temperature could inhibit structural changes and maintain hairtail tissue with more regular, smaller voids.
Changes in the tissue structure can be quantitatively analysed by measuring the fractal dimension, which is sensitive enough to distinguish tiny structural differences, size, or area fractions.[Citation32] In this study, it appropriately describes the uneven distribution of muscle fibres in hairtail tissue. The fractal dimension values of hairtail at various freezing temperatures and multiple freeze–thaw cycles are shown in . As can be seen from the figure, the initial fractal dimension of unfrozen hairtails was 1.9679, which was similar to the fractal dimension value (1.9550) of unfrozen tilapia, determined by He.[Citation33] In general, the fractal dimension results of all freezing temperatures exhibited a decreasing trend with the increase in the number of freeze–thaw cycles. At the end of storage, its value reached 1.3867, 1.5652, 1.5955, and 1.7445 in the samples that were frozen at −3℃, −18℃, −40℃, and −80℃, respectively. The decreasing rate was lower when frozen at a lower temperature. A lower fractal dimension indicates a higher degree of irregularity of the microstructure caused by ice crystals. Large and irregular ice crystals may impart mechanical damage by physically rupturing cells, which may result in a reduction in fractal dimension, an increase in drip loss, protein denaturation, and other quality changes related to the damage of the cell structure.[Citation34]
Relationship between fractal dimension and traditional quality parameters
The Pearson correlation coefficient is widely used to determine the degree of linear dependence between two variables.[Citation35] shows the Pearson correlation coefficients between fractal dimension and various traditional quality parameters, including the water holding capacity, TVBN, TBARS, and total plate count. Water holding capacity was positively correlated with the fractal dimension, whereas TVBN, TBARS, and total plate count were negatively correlated, and high correlation coefficients (0.905< R2 < 0.991) were obtained. The results suggested that fractal dimension can be applied as a reliable quality indicator to quantitatively assess variations in quality parameters.
Table 1. Pearson correlation coefficients of fractal dimension in relation to water holding capacity, TVBN, TBARS, and total plate count.
Conclusion
Structural and biochemical changes of hairtail tissues subjected to multiple freeze–thaw cycles were analysed in the present study. The fractal dimension, which quantifies the porous structure formed in tissue samples, as well as TVBN, TBARS, and total plate count all increased when the number of freeze–thaw cycles increased, whereas water holding capacity exhibited a decreasing trend. The rate of change of these parameters decreased with a decrease in the freezing temperature. Variations of fractal dimension subjected to multiple freeze–thaw cycles were significantly correlated with the traditional quality parameters. Therefore, the fractal dimension of hairtail tissue could be used as an effective indicator to characterize changes in the quality of hairtail during freezing and thawing process. Fractal dimension is a novel quality indicator that will provide new insights in the field of food preservation.
Additional information
Funding
References
- Luan, L.; Fu, S.; Yuan, C.; Ishimura, G.; Chen, S.; Chen, J. Combined Effect of Superchilling and Tea Polyphenols on the Preservation Quality of Hairtail (Trichiurus Haumela). International Journal of Food Properties 2017. DOI: 10.1080/10942912.2017.1325903.
- Luan, L.; Wu, C.; Wang, L.; Li, Y.; Ishimura, G.; Yuan, C. Protein Denaturation and Oxidation in Chilled Hairtail (Trichiutus Haumela) as Affected by Electrolyzed Oxidizing Water and Chitosan Treatment. International Journal of Food Properties 2017, 20, S2696–S2707. DOI: 10.1080/10942912.2017.1397693.
- Maqsood, S.; Benjakul, S.; Kamal-Eldin, A. Haemoglobin-Mediated Lipid Oxidation in the Fish Muscle: A Review. Trends in Food Science & Technology 2012, 28, 33–43. DOI: 10.1016/j.tifs.2012.06.009.
- Yarnpakdee, S.; Benjakul, S.; Kristinsson, H.G. Lipid Oxidation and Fishy Odour in Protein Hydrolysate Derived from Nile Tilapia (Oreochromis niloticus) Protein Isolate as Influenced by Haemoglobin. Journal of the Science of Food & Agriculture 2014, 94, 219–226. DOI: 10.1002/jsfa.2014.94.issue-2.
- Ayati, S.V.; Hamdami, N.; Le-Bail, A. Frozen Sangak Dough and Bread Properties: Impact of Pre-Fermentation and Freezing Rate. International Journal of Food Properties 2017, 20, 782–791. DOI: 10.1080/10942912.2016.1180535.
- Ali, S.; Zhang, W.; Rajput, N.; Khan, M.A.; Li, C.B.; Zhou, G.H. Effect of Multiple Freeze-Thaw Cycles on the Quality of Chicken Breast Meat. Food Chemistry 2015, 173, 808–814. DOI: 10.1016/j.foodchem.2014.09.095.
- Rn, S.S.P., BSN; CNORa; Director, O. R.;. Effects of Freezing and Thawing Methods and Storage Time on Thermal Properties of Freshwater Prawns (Macrobrachium rosenbergii). Journal of Aquatic Food Product Technology 2015, 75, 37–44.
- Kang, E.J. Physicochemical Properties of Alaska Pollock Surimi as Affected by Salinity and Freeze-Thaw Cycles. Journal of Food Science 2010, 73, C347-C355.
- Jeong, J.Y.; Kim, G.D.; Yang, H.S.; Joo, S.T. Effect of Freeze–Thaw Cycles on Physicochemical Properties and Color Stability of Beef Semimembranosus Muscle. Food Research International 2011, 44, 3222–3228. DOI: 10.1016/j.foodres.2011.08.023.
- Çorapcı, B.;. Effects of Multiple Freezing-Thawing Processes and Different Thawing Methods on Quality Changes of Anchovy (Engraulis engrasicholus L.). Journal of Food Agriculture & Environment 2013, 11, 185–189.
- Leygonie, C.; Britz, T.J.; Hoffman, L.C. Impact of Freezing and Thawing on the Quality of Meat: Review. Meat Science 2012, 91, 93–98. DOI: 10.1016/j.meatsci.2012.01.013.
- Omar, Z.; Rashid, N.A.; Fauzi, S.H.M.; Shahrim, Z.; Marangoni, A.G. Fractal Dimension in Palm Oil Crystal Networks during Storage by Image Analysis and Rheological Measurements. LWT - Food Science and Technology 2015, 64, 483–489. DOI: 10.1016/j.lwt.2015.04.059.
- Joardder, M.U.H.; Kumar, C.; Karim, A.; Brown, R.J. Fractal Dimension of Dried Foods: A Correlation between Microstructure and Porosity. N Food Structures, Digestion and Health International Conference, Melbourne 2013; pp 14.
- Chen, J.; Liu, M.; Zong, L. The Fractal Dimension Research of Chinese and American Beef Marbling Standards Images; Springer Berlin Heidelberg, heidelberg 2012; pp 199–209.
- Hultmann, L.; Rustad, T. Textural Changes during Iced Storage of Salmon (Salmo salar) and Cod (Gadus morhua). Journal of Aquatic Food Product Technology 2002, 11, 105–123. DOI: 10.1300/J030v11n03_09.
- Hongbo, M.I.; Qian, C.; Zhao, Y.; Liu, C.; Mao, L. Comparision of Superchilling and Freezing on the Microstructure, Muscle Quality and Protein Denaturation of Grass Carp (Ctenopharyngodon idellus). Journal of Food Processing & Preservation 2013, 37, 546–554. DOI: 10.1111/j.1745-4549.2012.00678.x.
- Siu, G.M.; Draper, H.H. A Survey of the Malonaldehyde Content of Retail Meats and Fish. Journal of Food Science 1978, 43, 1147–1149. DOI: 10.1111/jfds.1978.43.issue-4.
- Xuan, W.; Li, J.R.; Li, X.P.; Zhu, J.L. Quality Changes of Yellow Grouper (Epinephelus awoara) Fillets Stored under Vacuum Packaging at 0°C. Food Chemistry 2011, 126, 896–901. DOI: 10.1016/j.foodchem.2010.11.071.
- Wada, N.; Kawamoto, T.; Sato, Y.; Mano, N. A Novel Application of A Cryosectioning Technique to Undecalcified Coral Specimens. Marine Biology 2016, 163, 1–9. DOI: 10.1007/s00227-016-2895-x.
- Kawamoto, T.;. Use of a New Adhesive Film for the Preparation of Multi-Purpose Fresh-Frozen Sections from Hard Tissues, Whole-Animals, Insects and Plants. Archives of Histology & Cytology 2003, 66, 123. DOI: 10.1679/aohc.66.123.
- Kawamoto, T.; Kawamoto, K. Preparation of Thin Frozen Sections from Nonfixed and Undecalcified Hard Tissues Using Kawamot’s Film Method; Humana Press, New Jersey 2014; pp 149.
- Narine, S.S.; Marangoni, A.G. Relating Structure of Fat Crystal Networks to Mechanical Properties: A Review. Food Research International 1999, 32, 227–248. DOI: 10.1016/S0963-9969(99)00078-2.
- Liu, Q.; Chen, Q.; Kong, B.; Han, J.; He, X. The Influence of Superchilling and Cryoprotectants on Protein Oxidation and Structural Changes in the Myofibrillar Proteins of Common Carp (Cyprinus carpio) Surimi. LWT - Food Science and Technology 2014, 57, 603–611. DOI: 10.1016/j.lwt.2014.02.023.
- Offer, G.; Cousins, T. The Mechanism of Drip Production: Formation of Two Compartments of Extracellular Space in Muscle Post Mortem. Journal of the Science of Food & Agriculture 1992, 58, 107–116. DOI: 10.1002/(ISSN)1097-0010.
- Sriket, P.; Benjakul, S.; Visessanguan, W.; Kijroongrojana, K. Comparative Studies on the Effect of the Freeze–Thawing Process on the Physicochemical Properties and Microstructures of Black Tiger Shrimp (Penaeus monodon) and White Shrimp (Penaeus vannamei) Muscle. Food Chemistry 2007, 104, 113–121. DOI: 10.1016/j.foodchem.2006.11.004.
- Kaale, L.D.; Eikevik, T.M. The Influence of Superchilling Storage Methods on the Location/Distribution of Ice Crystals during Storage of Atlantic Salmon (Salmo salar). Food Control 2015, 52, 19–26. DOI: 10.1016/j.foodcont.2014.12.022.
- Liu, D.; Liang, L.; Xia, W.; Regenstein, J.M.; Zhou, P. Biochemical and Physical Changes of Grass Carp (Ctenopharyngodon idella) Fillets Stored at −3 and 0°C. Food Chemistry 2013, 140, 105–114. DOI: 10.1016/j.foodchem.2013.02.034.
- Khalafalla, F.A.; Ali, F.H.M.; Hassan, A.R.H.A. Quality Improvement and Shelf-Life Extension of Refrigerated Nile Tilapia (Oreochromis niloticus) Fillets Using Natural Herbs. Beni-Suef University Journal of Basic and Applied Sciences 2015, 4, 33–40. DOI: 10.1016/j.bjbas.2015.02.005.
- Wang, J.; Shahidi, F. Oxidative Stability of Marine Oils as Affected by Added Wheat Germ Oil. International Journal of Food Properties 2017, 11, 20-29.
- Benjakul, S.; Bauer, F. Biochemical and Physicochemical Changes in Catfish (Silurus glanis Linne) Muscle as Influenced by Different Freeze-Thaw Cycles. Food Chemistry 2001, 72, 207–217. DOI: 10.1016/S0308-8146(00)00222-3.
- Alizadeh, E.; Chapleau, N.; Mde, L.; Le-Bail, A. Effect of Different Freezing Processes on the Microstructure of Atlantic Salmon (Salmo salar) Fillets. Innovative Food Science & Emerging Technologies 2007, 8, 493–499. DOI: 10.1016/j.ifset.2006.12.003.
- Sarkar, N.; Chaudhuri, B.B. An Efficient Approach to Estimate Fractal Dimension of Textural Images. Pattern Recognition 1992, 25, 1035–1041. DOI: 10.1016/0031-3203(92)90066-R.
- He, Q.; Yang, Z.; Gong, B.; Wang, J.; Xiao, K.; Yang, S.T. Quality Evaluation Focusing on Tissue Fractal Dimension and Chemical Changes for Frozen Tilapia with Treatment by Tangerine Peel Extract. Scientific Reports 2017, 7, 42202. DOI: 10.1038/srep42202.
- Kaale, L.D.; Eikevik, T.M. A Histological Study of the Microstructure Sizes of the Red and White Muscles of Atlantic Salmon (Salmo Salar) Fillets during Superchilling Process and Storage. Journal of Food Engineering 2013, 114, 242–248. DOI: 10.1016/j.jfoodeng.2012.08.003.
- Niu, Y.; Kong, J.; Xiao, Z.; Chen, F.; Ma, N.; Zhu, J. Characterization of Odor-Active Compounds of Various Chinese “Wuliangye” Liquors by Gas Chromatography–Olfactometry, Gas Chromatography–Mass. Spectrometry and Sensory Evaluation. International Journal of Food Properties 2017, 53, 1532-2386.