ABSTRACT
It is difficult to maintain the high quality of sea cucumber Stichopus japonicus (S. japonicus) during regular thermal processing due to the special physiochemical makeup of S. japonicus body wall (SJBW) that affects its textural properties. Hence, it is very necessary to understand the parameters controlled textural alteration and the potential mechanisms during thermal processing of SJBW. In the present study, morphology, hardness and chewiness of SJBW gradually changed starting from 3 h during thermal processing. SDS-PAGE analysis indicated that notable proteolysis occurred in actin and other proteins with molecular weight of 200 kDa in a time-dependent manner. DNA fragmentation, cathepsin L and caspase-3-like activities also increased in SJBW. Within 1 h of incubation, both phosphorylated p38 and c-jun N-terminal kinase (JNK) and reactive oxygen species (ROS) production in SJBW reached the highest levels, and then returned back to the original levels after 3 h. A plausible signaling pathway in SJBW during low-temperature treatment is as follows: ROS produced, Mitogen-activated protein kinases (MAPK) phosphorylation, endogenous enzyme activation, DNA damage, protein degradation, and textural tenderization. Understanding the molecular mechanism underlying physiochemical changes during low-temperature treatment would be essential to achieve better control of the texture and quality of SJBW.
Introduction
Sea cucumber is an echinoderm and one of the important cultured aquatic species in China and other Asian countries due to its high commercial value.[Citation1] The total world production of sea cucumber in 2013 was about 232,000 tons (FAO). Stichopus japonicus (S. japonicus) is the most economically important species of more than 20 edible sea cucumbers in China.[Citation2] It is difficult to maintain the high quality of S. japonicus during regular thermal processing due to the special physiochemical makeup of S. japonicus body wall (SJBW) that affects its textural properties, which causes heavy economic losses in the seafood industry.[Citation3,Citation4] Therefore, it is very important to understand parameters that control textural alteration of SJBW during thermal processing, and how to improve its quality during processing.
Low-temperature heating has been developed to improve the eating quality of beef and pork.[Citation5] Tenderness is generally considered to be the most important eating quality characteristic of meat by consumers. The improved tenderness of meat heated at low temperature may be attributed partially to the action of proteolytic enzymes, causing weakening of fibrils, and solubilization of collagen.[Citation6] Likewise, in order to obtain the most desirable products, a low-temperature treatment may be applied to sea cucumber processing. Cathepsin, a type of lysosomal cysteine proteinase, may well be the most active proteinases in the human body. Cathepsin L (CL), a representative cathepsin, was thought to be mainly responsible for the modori phenomenon during surimi production.[Citation7] Caspases, a family of cysteine proteinases, play a central role in signal transduction and final execution in cell apoptotic processes. Currently, 14 members of the caspase family have been discovered in mammalian organisms mainly involved in interacting with the upstream adaptor molecules and down-stream caspases.[Citation8] In sea cucumber, cysteine proteases could affect the proteolysis of noncollagenous proteins[Citation9] and muscle tissues,[Citation10] which also suggested the cysteine proteases should be brought attention during tenderization. Mitogen-activated protein kinases (MAPK), including extracellular signal-regulated kinase (ERK), c-jun N-terminal kinase (JNK) and p38 MAPK, are serine-threonine kinases that play important roles in a variety of cellular activities.[Citation11] Caspase-3 activation and MAPK phosphorylation in sea cucumber have been reported by our research group during UVA radiation.[Citation4] In sea cucumber cells, chromatin condensation and marginalization as well as cytoplasmic condensation have been observed with apoptosis or apoptosis-like cell death.[Citation12] Oxidative stress results from the accumulation of reactive oxygen species (ROS) beyond the capacity of the cells to quench them. At low concentrations, ROS play an important role in cell signaling processes, whereas, at excessive amounts, they damage cellular macromolecules, such as proteins, lipids, and nucleic acids.[Citation13]
To the best of our knowledge, for the heating process in SJBW, most studies forced on the quality evaluation by various techniques,[Citation2,Citation14] but little is known about why the texture changed and whether oxidative stress induced the phenomenon. Therefore, the objective of this study was to further investigate the potential effects of ROS and their downstream signaling proteins and enzymes on the texture of SJBW, and to reveal the mechanism of textural changes in SJBW during low-temperature treatment, which will shine light on how to further improve thermal processes required to maintain the high quality of SJBW.
Materials and methods
Materials and chemicals
Living S. japonicus (150 ± 20 g) was purchased from a local aquatic market in Dalian (China). Bovine serum albumin (BSA), N,N,N,N-tetramethylethylenediamine (TEMED), Sodium dodecyl sulphate (SDS), Dithiothreitol (DTT) and Coomassie brilliant blue R-250 were obtained from Sangon Biotech Co., Ltd (Shanghai, China). Phenylmethanesulfonyl fluoride (PMSF) and Ethylenediaminetetraacetate (EDTA) were obtained from Sigma Chemical (St. Louis, MO). Caspase-3 activity kit was obtained from Beyotime Institute of Biotechnology (Haimen, China). Cathepsin L substrate was purchased from Santa Cruz Biotechnology (Shanghai, China). All of the other chemicals used in this study were of analytical grade.
Low-temperature treatment
The viscera and heads were removed from fresh sea cucumbers. Body walls were transferred to Petri dishes with 3–5 individuals in each, and were kept in HH3 Electro-Thermostatic Water Bath (Changzhou Zhongbei Instrument co. LTD, Jiangsu, China) at 37°C for 1/6, 1/2, 1, 3, 6, 12, 24, and 36 h, respectively. The samples were analyzed periodically to record their morphological changes. The sea cucumbers were frozen in liquid nitrogen as the control samples, and the body walls with no heating were as the 0 h samples. All the body walls and control samples were then stored at −80°C for further experiments.
Determination of soluble collagen content
Ten grams of SJBW were suspended in 10 mL of ultrapure water, and then placed into a water bath at 80°C for 2 h while keep shaking every 30 min. After 2 h, the samples were centrifuged at 1500 × g for 30 min. Determination of soluble collagen content was conducted following the AOAC method 990.26 described by Starkey et al.[Citation15] The results were expressed as mg/g of SJBW.
Instrumental texture analysis
Hardness and chewiness values were determined by using a TA-XT2i texture analyzer (Stable Micro Systems, Surrey, UK) fitted with a P/0.5 probe. Samples were cut into 1.5 × 1.5 × 1.5 cm cubes for analysis. Each sample underwent two cycles of compression analysis with a compression level of 70% (relative to sample width, 1 mm/s crosshead speed), with 5 s relaxation time between the two cycles according to Bourne and the SMS manual (Stable Micro Systems, Surrey, UK).[Citation16]
Evaluation of protein degradation
Protein degradation was analyzed using sodium dodecyl sulfate–polyacrylamide gel electrophoresis (SDS–PAGE) with a 5% stacking gel and a 10% running gel. The samples were ground with protein extraction solution (20 mM Tris-HCl (pH 7.5), 150 mM NaCl, 2 mM EDTA, 10 mM DTT, 1% Trition X-100, 0.1% SDS) by 1:3 (w/v) on ice. The extracts were then centrifuged at 12,000 × g for 15 min, at 4°C, and the supernatants were mixed with SDS-PAGE sample buffer (0.25 M Tris-HCl (pH 7.5), 8 M Urea, 5% SDS, 5% mercaptoethanol) at a ratio of 4:1, and boiled for 5 min. The boiled samples (10 μg) and markers were loaded into sample wells in the stacking gel and analyzed by using a Mini Protein system (Bio-Rad Laboratories, Berkeley, California, USA). After separation and staining with 0.05% Coomassie Brilliant Blue R-250, followed by destaining with 30% methanol and 10% acetic acid.
Measurement of CL activity
Samples were homogenized with protein extraction solution (50 mM phosphate buffer (pH 7.0), 0.1% Triton X-100, 1 mM EDTA) at 4°C by 1:3 (w/v) used glass grinders . The homogenates were then centrifuged at 9,600 × g for 10 min at 4°C by Legend Micro 17R centrifuge (Thermo Fisher Scientific, New York, USA), supernatants were collected for analysis of CL activity. The activity of CL was measured according to Qi et al.[Citation4] Z-Phe-Arg-Mec was used as the substrate for the determination of CL activity. The activity of CL was expressed as U/mg of protein.
Evaluation of DNA fragmentation
Treated SJBW samples for various times were collected, and DNA was procured using the method described by Miyoshi et al.[Citation17] The extracted DNA samples were examined by electrophoresis in 2.0% agarose gels; then the gels were then stained with gel red and imaged using an UV system (Bio-Rad Laboratories, Berkeley, California, USA).
Measurement of caspase-3 activity
The SJBW samples were homogenized with lysis buffer (25 mM HEPES (pH 7.4), 5 mM EDTA, 5 mM EGTA, 1 mM MgCl2, 5 mM DTT, 1 μM Pepstatin, 1 mM PMSF) and the lysates were centrifuged at 9,600 × g for 10 min at 4°C. The supernatants were then incubated with caspase-3 substrate acetyl-Asp-Glu-Val-Asp p-nitroanilide (Ac-DEVD-pNA) for 30 min at 37°C and the absorbance was read at 405 nm. Protein concentration of supernatants used in the activity assays were determined using a Bradford assay.[Citation18] Caspase-3 activity determined in treated samples was expressed by a relative increase to the control group.
Western blotting
The samples of SJBW were lysed in a lysis buffer (20 mM Tris-HCl (pH 7.5), 150 mM NaCl, 2 mM EGTA, 2 mM EDTA, 10 mM DTT, 10 mM NaF, 1 mM Na3VO4, 0.1% SDS, 1% Trion X-100, 10 μg/mL leupeptin, 10 μg/mL aprotinin, 1 mM PMSF) on ice. Lysates were centrifuged at 10,000 × g for 15 min, at 4°C, and supernatants were collected. The total proteins were quantified using the Bradford method. The supernatants were mixed with SDS-PAGE sample buffer at a ratio of 4:1 and boiled for 5 min. Then, 20 μg of protein were subjected to 10% SDS-PAGE and the total proteins were transferred into polyvinylidene difluoride (PVDF) membranes (Millipore, Billerica, Massachusetts, USA). The membranes were blocked and then incubated with anti-p-p38 (1:1000 dilution), anti-p-ERK1/2 (1:2000 dilution), anti-p-JNK (1:1000 dilution) and anti-β-actin antibody (1:1000 dilution) overnight at 4°C followed by the secondary antibody. Proteins were detected by enhanced chemiluminescence (ECL) reagent. Films were scanned and analyzed by the Chemi Doc system (Bio-Rad Laboratories, Berkeley, California, USA).
ROS measurement
The SJBW samples were directly lyophilized, subjected to electron spin resonance (ESR) spin trapping measurement. Briefly, 20 mg lyophilized powders were lodged into a glass capillary tube and placed in the ESR cavity. The ESR spectra were recorded using ESR spectrometer (Bruker A200, Karisruhe, Germany) at room temperature with the following settings: microwave power of 6.10 mw, sweep width 200.00 Gauss, center field 3460.00 Gauss, modulation frequency 100.00 kHz, modulation amplitude 1.00 gauss, time constant 5242.88 ms, and conversion time 480.00 ms.
Statistical analysis
All data were analyzed by the SPSS 16.0 (SPSS Inc., 2001, Chicago, IL, USA) and one-factor analysis of variance (ANOVA). For all assays, three separate experiments were performed. Means ± SE were calculated. Differences between means were considered to be significant at p < 0.05.
Results
Morphological and quality changes in SJBW during low-temperature treatment
The morphological changes of SJBW incubated at 37°C for different time periods are shown in ). With time progression, the morphology of the SJBW went through significant changes. The SJBW gradually became enlarged and stretched. The SJBW maintained its morphological integrity from 1/6 h to 3 h, and then started to collapse due to dermolysis from 3 to 36 h, with severe damage being observed from 12 to 36 h, until completely lost its shape (), 36 h). The effect of low-temperature treatment on soluble collagen content in the SJBW is shown in ). Compared with the control sample, no significant change was observed during the early period of tendering (before 1 h). From 3 to 36 h, soluble collagen content was increased gradually, with the most significant changes occurring from 12 to 36 h (p < 0.05). The maximum value of 16.6 ± 1.3 mg/g of sample (p < 0.05) was obtained at 36 h.
Figure 1. Changes of morphology and texture in SJBW during low-temperature treatment at 37°C. (a) The morphological observation; (b) soluble collagen of SJBW at 37°C for different time analyzed. Control was the fresh sample. The result is representative of three independent experiments; (c) changes in the hardness of SJBW at 37°C for different time; (d) changes in the chewiness of SJBW at 37°C for different time periods. Different letters indicate significant differences (p < 0.05).
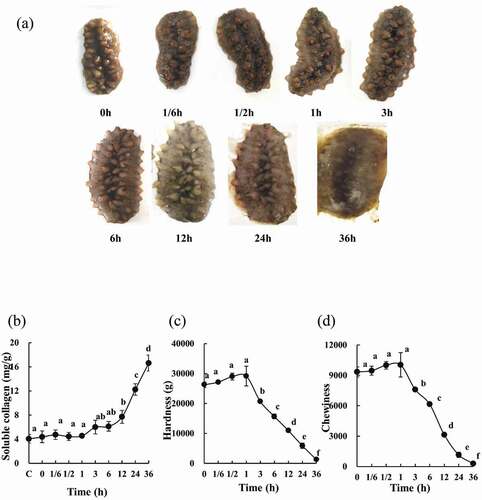
In order to determine the effect of low-temperature treatment on the quality of the product, textural analysis was conducted. The changes in hardness and chewiness of SJBW are shown in , respectively. Textural analysis results showed a slow increase in hardness until 1 h reaching a maximum value of 29137.6 ± 3272.7 g (p < 0.05), followed by a gradual decrease to 36 h with a minimum value of 1276.6 ± 113.1 g (p < 0.05). Similarly, the chewiness changes in the SJBW were observed at 37°C with the maximum and the minimum values of 10038.8 ± 1195.3 and 285.9 ± 120.3 (p < 0.05), respectively.
Changes of protein degradation and CL activity in the SJBW during low-temperature treatment
In order to identify the changes of proteins in SJBW, the proteins were extracted and analyzed by SDS-PAGE. The changes of the proteins are shown in ). Clearly, two protein bands with molecular weights of 200 kDa (High Molecular Weight, HMW) and 44.3 kDa (actin) are observed in ). The actin band remained visible until 12 h, and the HMW appeared to degrade starting from 6 h and then almost completely disappeared after 24 h during low-temperature treatment at 37°C. Apparently, long-time treatment caused serious degradation of structural proteins such as actin.
Figure 2. Protein degradation and change in CL activity in SJBW during low-temperature treatment at 37°C. (a) SDS–PAGE photographs of protein degradation. Ten micrograms of each sample were loaded in 10% (w/v) SDS–PAGE gel. HM, high MARK; LM, low MARK; (b) CL activity measured by a fluorospectrophotometer. Data are reported as mean ± SD based on three replicates. Different letters indicate significant differences (p < 0.05). (c) Control was the fresh sample.
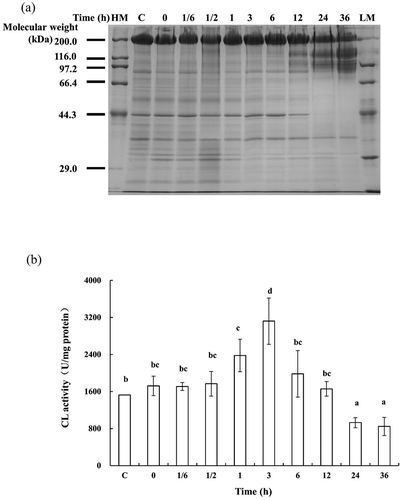
It has been reported that CL was an important factor in proteolysis of sea cucumber.[Citation19] ) shows the activity of CL which increased gradually and achieved a maximum value of 156.1 ± 15.0 U/mg protein (p < 0.05) after a 3 h incubation at 37°C and then decreased significantly (p < 0.05). A peak in CL activity existed at 3 h incubation. It seems that CL was released from the lysosome in cells, and then went through activation/deactivation cycle when SJBW was undergoing low-temperature treatment at 37°C. CL might affect protein degradation significantly starting from 6 h ()), since the increased CL activity modulated changes in protein content.
DNA fragmentation and caspase-3 activity changes in SJBW during low-temperature treatment
To investigate whether apoptosis was happening in SJBW during low-temperature treatment at 37°C, DNA fragmentation and caspase-3 activity were determined. ) shows typical results of DNA fragmentation analysis of the SJBW incubated at 37°C. Degradation of DNA on agarose gel was observed in the treated samples from 1 to 6 h. Severe DNA damage was observed in samples from 12 to 36 h.
Figure 3. DNA fragmentation and caspase-3 activation in SJBW during low-temperature treatment at 37°C. (a) DNA fragmentation analyzed by agarose electrophoresis. The result is representative of three independent experiments; (b) caspase-3 activity was measured by using a spectrophotometer. The values represent means ± SD of three separate experiments. Different letters indicate significant differences (p < 0.05). Control was the fresh sample.
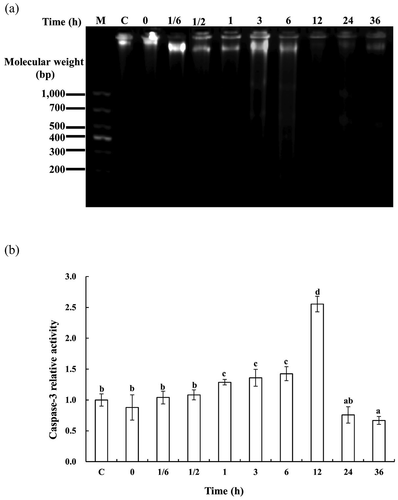
Caspase-3 activity was measured by conversion of the substrate Ac-DEVD-pNA into free pNA. The incubated samples from 1 to 6 h showed an increase in caspases-3 activity at a higher level than the samples in the earlier stages (p < 0.05) in ). The caspase-3 activity was significantly higher at 12 h and reached the maximum value of 2.6 ± 0.1 (p < 0.05) times of the control, and then declined at the later stages in SJBW (p < 0.05). The activation of caspase-3 strongly suggests that apoptosis actually occurred in SJBW during low-temperature treatment at 37°C ()).
MAPK phosphorylation in the SJBW during low-temperature treatment
The MAPK signaling pathways are activated under stress in many organisms, they can be triggered by ionizing radiation, oxidative stress, and heat shock.[Citation20] The regulation of MAPK by incubation at 37°C was investigated using Western blot. As shown in , the phosphorylated p38 MAPK and JNK increased instantaneously from 10 min to 1 h, and then disappeared after 3 h. In contrast, the activation of ERK was not induced. These results suggest that both the p38 and JNK pathways, but not the ERK, might be involved in SJBW during low-temperature treatment at 37°C. The western blot results also showed that β-actin degraded after 12 h treatment at 37°C, consistent with the data shown in ).
Figure 4. MAPK phosphorylation in SJBW during low-temperature treatment at 37°C. Twenty micrograms of protein was loaded per lane. Control was the fresh sample. Samples were analyzed with western blotting to determine the phosphorylation of p38, JNK, and ERK. β-actin was used as a loading control. Results are representative of three independent experiments.
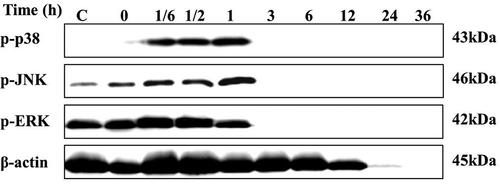
Free radical formation in SJBW during low-temperature treatment
The formation of free radicals was evaluated by subjecting lyophilized powder of SJBW to low-temperature treatment using ESR spectroscopy. ) shows typical ESR spectra of the samples. The observed ESR signal resembled the free radical produced in most biological systems with g = 2.03, which was identical to ROS-derived radical. The relative radical signal intensity is shown in ). As for samples incubated at 37°C, the relative radical intensity increased significantly (p < 0.05) as time progressed and reached a maximum value of 8.8 ± 0.2 (×105) (p < 0.05) incubated after 1 h, followed by a decrease.
Scheme of signal cascade in SJBW during low-temperature treatment
As illustrated in , we propose a signal pathway that dictates the changes in SJBW when it was treated at 37°C. The production of ROS induces phosphorylation of p38 and JNK, which activates downstream proteolytic enzymes (CL and caspase-3). The CL activation promotes degradation of structural proteins and collagen dissolution, whereas caspase-3 leads to DNA fragmentation. Consequently, protein degradation, collagen dissolution, and DNA damage lead to significant textural changes in SJBW.
Discussion
SJBW was composed of an epidermis, a dermis, and an inner circular muscle layer.[Citation21] The reduction in quality of SJBW was more visible in epidermis than in dermis during low-temperature treatment at 37°C. The significant increase in soluble collagen content over time was observed in the process of low-temperature treatment at 37°C in SJBW ()). Similarly, the results of soluble collagen content increased were found in thermal treated abalone muscle and lamb longissimus within aging periods.[Citation12,Citation15] These studies indicated that the collagen was denatured and degraded during different temperature treatment. In the present study, the textural analysis results could be explained by the dissolution of collagen, because the change in the content of soluble collagen coincides with the decline of hardness and chewiness. Similarly, Lu et al.[Citation22] reported the hardness and springiness of bighead carp fillets at 4°C increased until 8 h, followed by a slow decrease to the end of storage.
The HMW and actin were the main protein components in SJBW, and their degradations would have a significant influence on the muscle structure.[Citation23] Degradation of actin and HMW was observed in low-temperature treatment at 37°C in SJBW ()), similar to that observed in herring, catfish, and tilapia.[Citation23,Citation24] A negative correlation existed between protein degradation and CL activity after 3 h in SJBW; the degradation of protein might be attributed to the accumulation of CL from 10 min to 3 h ()). Ladratet et al.[Citation25] also reported that protein degradation during storage for sea bass might be attributed to the action of CL.
The DNA damage in SJBW during low-temperature treatment was observed ()). Heat stress could induce DNA break and inhibit DNA replication.[Citation26] Caspase-3 might be involved in the increased DNA damage observed in animal skeletal muscles.[Citation27] The caspase-3 activity in SJBW first increased and then decreased over time in low-temperature treatment ()). Caspase-3 activation was associated with the decline of shear force and the mitochondrial pathway played a part in bovine skeletal muscles during the postmortem.[Citation28,Citation29] Previous studies on the correlation between cathepsins and caspases were inconclusive, thus it was suggested that cathepsins might be at the downstream, or upstream of the caspases activation, and they may substitute for the caspases, or are completely independent as the executioner proteases.[Citation30] Nevertheless, this study indicated that caspase-3 was at the downstream of CL in SJBW.
It has been reported that MAPK regulates DNA damage as well as the caspase-3 activity in nucleus pulposus cells and human breast cancer cells MCF-7.[Citation31,Citation32] The significant activation of p38 and JNK in SJBW () was consistent with a report on Sarcophaga crassipalpis.[Citation33] However, ERK was not significantly phosphorylated in SJBW during low-temperature treatment. ERK phosphorylation has been implicated in cell proliferation and cell cycle progression, while p38 and JNK are more commonly activated in response to stress and cellular damage.[Citation34] Therefore, the ERK phosphorylation might play a role of protective signal against apoptosis in SJBW. Nonetheless, these results indicated that p38 and JNK contribute to the change of SJBW quality.
Contribution of ROS over-production to injury in cells have previously been reported.[Citation35] Although the detailed mechanisms involved in the degradation of texture in SJBW remain unclear, the evidence so far suggests the involvement of ROS in cell injury. For example, UVB-induced ROS promotes apoptosis accompanied with MAPK activation in sea urchin embryos and heat stress induced ROS production in celomocytes of Eisenia hortensis.[Citation36,Citation37] Similarly, our results demonstrate that low-temperature treatment led to free radical formation as observed in ESR spectra (). A number of studies have reported that overproduction of ROS is harmful to the normal metabolism and induces lipid peroxidation, protein degradation, and DNA damage.[Citation38] Heat stress stimulates ROS production and protein degradation in skeletal muscles at 37°C.[Citation39] The ROS-induced DNA damage has been observed during heat stress.[Citation40] We found that low-temperature heating generates a large amount of ROS at 1 h, when a series of important signaling proteins such as p38 were activated. The detail signal cascade of ROS induced-endoenzyme regulated texture remains to be clarified.
Conclusion
Low-temperature treatment of SJBW at 37°C induced ROS formation, MAPK phosphorylation, protein degradation regulated by CL activation, as well as DNA damage and caspase-3 activation. The ROS production is the earliest event. Although it needs to be further investigated how ROS and the downstream signaling affect the texture of SJBW during low-temperature treatment. Potentially these findings can lead to improved processing of sea cucumber to yield better products with more consistent quality.
Additional information
Funding
References
- Wu, Z.; Chen, H.; Wang, W.; Jia, B.; Yang, T.; Zhao, Z. Differentiation of Dried Sea Cucumber Products from Different Geographical Areas by Surface Desorption Atmospheric Pressure Chemical Ionization Mass Spectrometry. J. Agric. Food Chem. 2009, 57, 9356–9364. DOI: 10.1021/jf9018504.
- Bi, J. R.; Li, Y.; Cheng, S. S.; Dong, X. P.; Kamal, T.; Zhou, D. Y.; Li, D. M.; Jiang, P. F.; Zhu, B-W..Tan, M. Q. Changes in Body Wall Of Sea Cucumber (Stichopus Japonicus) during a Two-step Heating Process Assessed by Rheology, Lf-nmr, and Texture Profile Analysis. Food Biophysics. 2016, 11, 257-265. DOI: 10.1007/s11483-016-9437-4.
- Qi, H.; Dong, X. F.; Zhao, Y. P.; Li, N.; Fu, H.; Feng, D. D. ROS Production in Homogenate from the Body Wall of Sea Cucumber Stichopus Japonicus, under UVA Irradiation: ESR Spin-Trapping Study. Food Chem. 2016a, 192, 358–362. DOI: 10.1016/j.foodchem.2015.07.030.
- Qi, H.; Fu, H.; Dong, X.; Feng, D.; Li, N.; Wen, C. Apoptosis Induction Is Involved in UVA-induced Autolysis in Sea Cucumber Stichopus Japonicus. J. Photochem. Photobiol. B 2016b, 158, 130–135. DOI: 10.1016/j.jphotobiol.2016.02.034.
- Christensen, L.; Gunvig, A.; Tørngren, M. A.; Aaslyng, M. D.; Knøchel, S.; Christensen, M. Sensory Characteristics of Meat Cooked for Prolonged Times at Low Temperature. Meat Sci. 2012, 90, 485–489. DOI: 10.1016/j.meatsci.2011.09.012.
- Huang, M.; Huang, F.; Xue, M.; Xu, X.; Zhou, G. The Effect of Active Caspase-3 on Degradation of Chicken Myofibrillar Proteins and Structure of Myofibrils. Food Chem. 2011, 128, 22–27. DOI: 10.1016/j.foodchem.2011.02.062.
- Zhong, C.; Cai, Q. F.; Liu, G. M.; Sun, L. C.; Hara, K.; Su, W. J.; Cao, M. J. Purification and Characterisation of Cathepsin L from the Skeletal Muscle of Blue Scad (Decapterusmaruadsi) and Comparison of Its Role with Myofibril-Bound Serine Proteinase in the Degradation of Myofibrillar Proteins. Food Chem. 2012, 133, 1560–1568. DOI: 10.1016/j.foodchem.2012.02.050.
- Wang, K. K. Calpain and Caspase: Can You Tell the Difference? Trends Neurosci. 2000, 23, 20–26. DOI: 10.1016/S0166-2236(99)01479-4.
- Wu, H. T.; Li, D. M.; Zhu, B. W.; Sun, J. J.; Zheng, J.; Wang, F. L.; Konno, K.; Jiang, X. Proteolysis of Noncollagenous Proteins in Sea Cucumber, Stichopus Japonicus, Body Wall: Characterisation and the Effects of Cysteine Protease Inhibitors. Food Chem. 2013, 141, 1287–1294. DOI: 10.1016/j.foodchem.2013.03.088.
- Zhao, C. C.; Yang, Y.; Wu, H. T.; Zhu, Z. M.; Tang, Y.; Yu, C. P.; Sun, N.; Lv, Q.; Han, J. R.; Li, A. T.; et al. Characterization of Proteolysis in Muscle Tissues of Sea Cucumber Stichopus Japonicus. Food Sci. Biotechnol. 2016 25, 1529–1535. DOI: 10.1007/s10068-016-0237-x.
- Chen, J.; Guo, R.; Yan, H. Naringin Inhibits ROS-activated MAPK Pathway in High Glucose-Induced Injuries in H9c2 Cardiac Cells. Basic Clin. Pharmacol. Toxicol. 2014, 114, 293–304. DOI: 10.1111/bcpt.12209.
- Zhu, B. W.; Dong, X. P.; Sun, L. M.; Xiao, G. H.; Chen, X. J.; Murata, Y.; Yu, C. X. Effect of Thermal Treatment on the Texture and Microstructure of Abalone Muscle (Haliotis Discus). Food Sci. Biotechnol. 2011, 20, 1467–1473. DOI: 10.1007/s10068-011-0203-6.
- Stadtman, E. R. Oxidation of Free Amino Acids and Amino Acid Residues in Proteins by Radiolysis and by Metal-Catalyzed Reactions. Annu. Rev. Biochem. 2003, 62, 797–821. DOI: 10.1146/annurev.bi.62.070193.004053.
- Dong, X. P.; Li, Y.; Li, Y.; Song, L.; Cheng, S. S.; Li, D. M.; Zhu, B. W.; Zhou, D. Y.; Tan, M. Q. Combination of NMR and MRI Techniques for Non-Invasive Assessment of Sea Cucumber (Stichopus Japonicas) Tenderization during Low-Temperature Heating Process. Food Anal. Methods. 2017. DOI: 10.1007/s12161-016-0770-5.
- Starkey, C. P.; Geesink, G. H.; Oddy, V. H.; Hopkins, D. L. Explaining the Variation in Lamb Longissimus Shear Force across and within Ageing Periods Using Protein Degradation, Sarcomere Length and Collagen Characteristics. Meat Sci. 2015, 105, 32–37. DOI: 10.1016/j.meatsci.2015.02.011.
- Bourne, M. C. Texture Profile Analysis. Food Technol. 1978, 32, 62–66.
- Miyoshi, N.; Watanabe, E.; Osawa, T.; Okuhira, M.; Murata, Y.; Ohshima, H. ATP Depletion Alters the Mode of Cell Death Induced by Benzyl Isothiocyanate. Biochim. Biophys. Acta 2008, 1782, 566–573. DOI: 10.1016/j.bbadis.2008.07.002.
- Bradford, M. M.;. A Rapid and Sensitive Method for the Quantitation of Microgram Quantities of Protein Utilizing the Principle of Protein-Dye Binding. Anal. Biochem. 1976, 72, 248–254. DOI: 10.1016/0003-2697(76)90527-3.
- Zhou, D. Y.;Chang, X. N.; Bao, S. S.; Song, L.; Zhu, B. W.; Dong, X. P.; Zong, Y.; Li, D. M.; Zhang, M. M. Liu, Y. X.; et al. Purification and Partial Characterisation of a Cathepsin L-Like Proteinase from Sea Cucumber (Stichopus Japonicus) and Its Tissue Distribution in Body Wall. Food Chem. 2014, 158, 192-199. DOI:10.1016/j.foodchem.2014.02.105.
- Brancho, D.; Tanaka, N.; Jaeschke, A.; Ventura, J. J.; Kelkar, N.; Tanaka, Y. Mechanism of P38 Map Kinase Activation in Vivo. Genes Dev. 2003, 17, 1969–1978. DOI: 10.1101/gad.1107303.
- Menton, D. N.; Eisen, A. Z. The Structure of the Integument of the Sea Cucumber, Thyonebriareus. J. Morphol. 2010, 131, 17–35. DOI: 10.1002/jmor.1051310103.
- Lu, H.; Liu, X.; Zhang, Y.; Wang, H.; Luo, Y. Effects of Chilling and Partial Freezing on Rigor Mortis Changes of Bighead Carp (Aristichthysnobilis) Fillets: Cathepsin Activity, Protein Degradation and Microstructure of Myofibrils. J. Food Sci. 2015, 80, C2725–C2731. DOI: 10.1111/1750-3841.13134.
- Timson, D. J. Fine Tuning the Myosin Motor: The Role of the Essential Light Chain in Striated Muscle Myosin. Biochimie. 2003, 85, 639–645.
- Thavaroj, W.; Pansawat, N.; Konno, K. Thermal Denaturation Profiles of Catfish and Tilapia Myofibrils as Affected by pH for Heating. Fish. Sci. 2012, 78, 431–439. DOI: 10.1007/s12562-011-0444-6.
- Ladrat, C.; Verrezbagnis, V.; Noel, J.; Fleurence, J. In Vitro Proteolysis of Myofibrillar and Sarcoplasmic Proteins of White Muscle of Sea Bass (Dicentrarchus Labrax L.): Effects of Cathepsins B, D and L. Food Chem. 2003, 81, 517–525. DOI: 10.1016/S0308-8146(02)00481-8.
- Kantidze, O. L.; Velichko, A. K.; Luzhin, A. V.; Razin, S. V. Heat Stress-Induced DNA Damage. Acta Nat. 2016, 8, 75–78.
- Quadrilatero, J.; Rush, J. W. Increased DNA Fragmentation and Altered Apoptotic Protein Levels in Skeletal Muscle of Spontaneously Hypertensive Rats. J. Appl. Physiol. 2006, 101, 1149–1161. DOI: 10.1152/japplphysiol.00194.2006.
- Cao, J. X.; Ou, C. R.; Zou, Y. F.; Ye, K. P.; Zhang, Q. Q.; Khan, M. A.; Pan, D. D.Zhou, G. Activation Of Caspase-3 and Its Correlation with Shear Force in Bovine Skeletal Muscles during Postmortem Conditioning. J. Anim. Sci. 2013, 91, 4547-4552. DOI: 10.2527/jas.2013-6469.
- Huang, F.; Huang, M.; Zhang, H.; Zhang, C.; Zhang, D.; Zhou, G. Changes in Apoptotic Factors and Caspase Activation Pathways during the Postmortem Aging of Beef Muscle. Food Chem. 2016, 190, 110–114. DOI: 10.1016/j.foodchem.2015.05.056.
- Jäättelä, M.; Tschopp, J. Caspase-Independent Cell Death in T Lymphocytes. Nat. Immunol. 2003, 4, 416–423. DOI: 10.1038/ni0503-416.
- Ravindran, J.; Gupta, N.; Agrawal, M.; Bhaskar, A. S. B.; Rao, P. V. L. Modulation of ROS/MAPK Signaling Pathways by Okadaic Acid Leads to Cell Death Via, Mitochondrial Mediated Caspase-Dependent Mechanism. Apoptosis. 2011, 16, 145–161. DOI: 10.1007/s10495-010-0554-0.
- Zhai, J. W.; Gao, C.; Ma, W. D.; Wang, W.; Yao, L. P.; Xia, X. X.; Luo, M.; Zu, Y. G.Fu, Y. J. Geraniin Induces Apoptosis of Human Breast Cancer Cells MCF-7 via ROS-mediated Stimulation of P38 MAPK. Toxicol. Mech. 365 Methods. 2016, 26, 311-318. DOI: 10.3109/15376516.2016.1139025.
- Fujiwara, Y.; Denlinger, D. L. P38 MAPK Is a Likely Component of the Signal Transduction Pathway Triggering Rapid Cold Hardening in the Flesh Fly Sarcophaga Crassipalpis. J. Exp. Biol. 2007, 210, 3295–3300. DOI: 10.1242/jeb.006536.
- Xia, Z.; Dickens, M.; Raingeaud, J.; Davis, R. J.; Greenberg, M. E. Opposing Effects of ERK and JNK-p38 Map Kinases on Apoptosis. Science. 1995, 270, 1326–1331.
- Chen, S. L.; Yang, C. T.; Yang, Z. L.; Guo, R. X.; Meng, J. L.; Cui, Y.; Lan, A. P.; Chen, P. X.Feng, J. Q. Hydrogen Sulphide Protects H9c2 Cells against Chemical Hypoxia-induced Injury. Clin. Exp. Pharmacol. Physiol. 2010, 37, 316-321. DOI: 10.1111/j.1440-1681.2009.05289.x.
- Bonaventura, R.; Poma, V.; Costa, C.; Matranga, V. UVB Radiation Prevents Skeleton Growth and Stimulates the Expression of Stress Markers in Sea Urchin Embryos. Biochem. Biophys. Res. Commun. 2005, 328, 150–157. DOI: 10.1016/j.bbrc.2004.12.161.
- Tumminello, R. A.; Fuller-Espie, S. L. Heat Stress Induces ROS Production and Histone Phosphorylation in Celomocytes of Eisenia Hortensis. Invertebrate Surv. J. 2013, 10(1), 50–57.
- Jena, K. B.; Jagtap, T. G.; Verlecar, X. N. Antioxidative Potential of Pernaviridis and Its Protective Role against ROS Induced Lipidperoxidation and Protein Carbonyl. Curr. Trends Biotechnol. Pharm. 2010, 4, 862–870.
- Furukawa, K.; Kikusato, M.; Kamizono, T.; Yoshida, H.; Toyomizu, M. Possible Involvement of Mitochondrial Reactive Oxygen Species Production in Protein Degradation Induced by Heat Stress in Avian Muscle Cells. J. Poultr. Sci. 2015, 52, 129–135. DOI: 10.2141/jpsa.0150028.
- Bruskov, V. I.; Malakhova, L. V.; Masalimov, Z. K.; Chernikov, A. V. Heat-Induced Formation of Reactive Oxygen Species and 8-Oxoguanine, a Biomarker of Damage to DNA. Nucleic Acids Res. 2002, 30, 1354–1363.