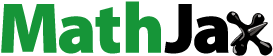
ABSTRACT
The physicochemical and digestive properties of Pleurotus eryngiiprotein and the effects of heat treatment and ultrasound treatment on the properties were investigated. The physicochemical property results showed that the shear stress and viscosity increased as the protein concentration increased from 3 to 9 g/100 g raw materials and decreased as the temperature increased from 25°C to 45°C. The pH value from 2.0 to 7.0 significantly affected the state of protein because of the change of the disulfide bond and sulfhydryl bond content. The protein might be held closer with higher sulfhydryl bond at pH 7.0 and be less coacervation with higher disulfide bonds at pH 3.5. The addition of calcium from 0.01 to 0.05 mol/L into the protein system could increase the rheology property of the protein. Based on the results of Fourier-transform infrared spectroscopy, the content of α-helix increased after heat treatment and ultrasound treatment, while the content of β-sheet decreased, indicating the change of spatial conformation of protein. The result of differential scanning calorimetry of protein manifested that ultrasound treatment significantly enhanced the protein thermal stability (P < 0.05). The digestive property results indicated that heat treatment and ultrasound treatment increased the available amino groups (DH) and vitro digestibility of protein digestive juice and reduced the content of peptides. There were more kinds of amino acids of protein digestion with ultrasound treatment than non-treatment and heat treatment. In addition, the protein after ultrasound treatment exhibited excellent antioxidant properties in in vitro digestion.
Introduction
Pleurotus eryngii is an edible and medicinal mushroom, which is a tetra-polar basidiomycete belonging to the Pleurotus sp.[Citation1] It has been widely used as an admired functional and medicinal food in China, particularly owing to its features such as unique stipe, pleasant aroma, and good culinary qualities. It also contains countless bio-active substances like protein, polysaccharides (e.g., α- and β-glucan), peptide, and dietary fiber, among others,[Citation2] and it may possess potential bio-active functional and nutritional properties such as anti-proliferate, antioxidant, and anti-tumor activities,[Citation3] thus leading to higher production of P. eryngii quickly and majorly in Asia, Europe, and America.[Citation4] In recent years, the domestic and foreign investigators of the P. eryngii have focused primarily on the biological properties and bio-active substances,[Citation5] such as polysaccharides (e.g., β-glucan), with its potential immune regulation[Citation6] and anti-tumor[Citation7] and antioxidant activities,[Citation8] and sterols (e.g., estrogen-like) with its protection against the bone loss, among others.[Citation9] Protein serves as one of the significant food components and provides more functional and nutritional value. According to the previous researches, different kinds of protein in the stomach and intestinal digestion might present different digestive properties.[Citation10] However, there are only a few reports about the physicochemical and digestive properties of P. eryngii protein and its digestive products and antioxidant properties.
Generally speaking, the physicochemical properties include rheological properties, surface hydrophobicity (H0), secondary structure, and thermal stability. The rheological property plays an important role in processing and maintaining the texture stability of food, which is reflected by the apparent viscosity in general. And it affects the sensory quality and functionality of resultant products. Testing for rheological property can provide countless valuable information for food composition, internal structure, and molecular morphology, such as elastic or storage modulus (G’), viscous or loss modulus (G’’), and phase angle (tanα). H0 and secondary structure testing can offer some important information about water-holding capacity and breaking force of protein. The previous studies reported that the fish protein, extracted by using alkaline method, exhibits strong breaking force and low deformation.[Citation11] pH value is a key factor in the processing of protein as it has a significant effect on rheological properties.[Citation12] Salts dominant in manufacturing gels and its concentration can enhance the polymerization process by promoting the cross-linkage or electrostatic interactions in the protein system.[Citation13] Protein aftrer stimulating in stomach and intestinal manifests different antioxidant activity. However, there are few reports about the effect of heat treatment and ultrasound treatment on physicochemical and digestive properties of P. eryngii protein.
Heat treatment has extensive application in the food processing industry to promote food functional properties.[Citation14] It also reported that food through pre-heat treatment could decrease enzyme activity and enhance food sensory characterization like flavor, texture, and color properties.[Citation15] Protein treated by heat treatment before enzymatic hydrolysis can rearrange the inter-linkage between protein molecules, particularly hydrophobic bonds, thus resulting in changes of protein structure and digestibility properties.[Citation16] The ultrasound technology serves as an important non-thermal physical treating technology, which features many applications in food processing.[Citation17] More importantly, the ultrasound technology enables to bring about acoustic cavitation, which results from the mechanical interaction with strong shear force by bubbles burst instantly in liquid.[Citation18] The ultrasound technology was accepted to promote the protein functional properties at large and to generate bio-active peptides. It is also reported that the ultrasound treatment can increase the rate of protein hydrolysis with enzyme and the bio-activity of hydrolysis products dramatically.[Citation19] The ultrasound treatment can change protein secondary structure as well as protein physical and chemical properties, thus bringing more protease restriction sites.[Citation20] It is reported that whey protein treated by heat and ultrasound could improve its functional properties.[Citation21] But few systematic researches have concentrated on the effects of heat treatment and ultrasound treatment on physicochemical properties like surface hydrophobicity, sulfhydryl content, and secondary structure.
The relationship between physicochemical and digestive properties of P. eryngii protein is not fully explored. Therefore, the present project aimed to investigate the physicochemical and digestive properties of P. eryngii protein and antioxidant activity of digestive products. The mechanism was researched in terms of the changes in protein physicochemical properties, digestive products antioxidant of P. eryngii protein.
Materials and methods
Materials
P. eryngii was kindly provided by the Edible Fungus Engineering Technology Center (Taigu, China) and processed into 150 particles for later use. Sigma-BSA (A3912-10 g), Sigma-lipase (5 g), Sigma-mucin (100 g), and Sigma-bile (25 g) were purchased from Shanghai North Connaught Biological Technology Co., Ltd.
Preparation of P. eryngii protein
Protein was extracted by combining cellulose enzymatic hydrolysis and acid precipitation after alkaline extraction from P. eryngii and concentrated P. eryngii powder and distilled water according to a certain liquid to material ratio of 1:55, and 1.5% cellulase was added (based on the raw material dry powder), and then the solution was placed in water bath at a constant temperature of 40°C for 100 min, and then the solution was inactivated at 80°C for 10 min. Next, the pH was adjusted to 12, and the reaction was carried out at 55°C for 2.5 h after cooling. After centrifugation at 4,000 r/min for 15 min, the supernatant was collected. The pH of the supernatant was adjusted to the isoelectric point of P. eryngii(3.6)with 0.1 mol/L HCl. After centrifugation at 4,000 r/min for 10 min, the protein was precipitated and dissolved with distilled water. The NaOH of mol/L was adjusted to pH 7.0 to obtain the P. eryngii protein solution. Then, the protein solution was dealt with liquid nitrogen and vacuum freeze-dried. to obtain P. eryngii protein power.[Citation22]
Physicochemical properties of P. eryngii protein and the effect of treatments on protein
Rheological property: Rheological property of the protein was measured by using a DHR-3 rheometer (TA Instruments, New Castle, DE). A total of 25 mL prepared protein solution was poured into the cylinder tube, and paraffin oil was added to the sample solution surface to prevent volatilization of water. Each sample solution was subjected to a four-part test: (1) Shear rate-viscosity test. Parameter settings: the share rate range was 0.1–200 s−1, and under the share rate of 200 s−1 for 1 min, it was restarted from 200 to 0.1 s−1. Subsequently, the temperature increased from 25°C to 45°C at 5°C/min. (2) Angular frequency-viscosity test. Parameter settings: the angular frequency range was 0.5–100 s−1, and the controlled strain was changed to 0.5%. The automatic stress was selected, and the initial stress was set at 0.8 (Pa s). (3) Temperature scanning test. Parameter settings: the angular frequency was controlled at 10 rad/s, and the controlled strain was changed to 0.5%. The temperature program was set at the temperature range from 25°C to 65°C at 1°C/min, under 65°C for 1 min, and the temperature was restarted from 65°C to 25°C at 1°C/min. G’, G’’, and tanα were measured. The flow behavior of protein was manifested with the Power Law model:
where τ is the shear stress (Pa), K is consistency coefficient (Pa sn), r is the shear rate (s−1), and n is the flow behavior index.
Surface hydrophobicity (H0): According to the method of Wang et al. with minor changes, the surface hydrophobicity (H0) of protein was measured adopting ANS (8-Aniline-1-Naphthalenesulfonic acid) with the hydrophobic fluorescence probe.[Citation23] The different protein samples were dissolved in 0.02 M phosphate butter (PBS, pH 7.0) and centrifuged at 8,000 g for 15 min at 4°C and collected the supernatant. The protein solution was prepared with PBS to obtain the final concentrations of 0.1, 0.2, and 0.3 M. Each protein sample (2.5 mL) added was mixed well with 8 mM ANS 12.5 μL at 25 ± 0.5°C. Absorption intensity was measured at excitation of 390 nm and emission of 470 nm using a multifunctional enzyme-labeled instrument (Spectra MX I3X). The protein sample intrinsic emission fluorescence spectrum was determined by using a multifunctional enzyme-labeled instrument (Spectra MX I3X) according to Liu et al. with some changes.[Citation24] Protein sample solutions with concentration of 0.02 mg/mL were prepared in 0.02 mol/L PBS (pH 7.0). Subsequently, the protein sample solutions were analyzed at excitation spectra of 280 nm and emission spectra from 300 to 650 nm at a constant slit of 5 nm for all samples analyzed. The initial slope of the relative fluorescence intensity versus protein concentration (mg/mL) plot was analyzed by linear regression analysis and used as an index of H0.
Sulfhydryl content and the disulfide bond contents: The R-SH of the protein solution was determined as described in Omama et al.[Citation25] and Zhou et al.[Citation26] Protein solution (2.5 mL) and EDTA adding (7 mM) in 25 mL of tris-glycine buffer (0.1 mol/L pH 8.0); then, the solution was mixed well using the Kinematica (FT-2500E). Sample solution (1mL) adding 4 mL tris-glycine buffer and 100 μL Ellman’s reagent (10 mM DTNB) mixing well at a room temperature (25°C ± 1°C) for 20 min to measure the R-SH content at 412 nm adopting a multifunctional enzyme-labeled instrument (Spectra MX I3X). The solutions without Ellman’s reagent were set as a reference, and the R-SH content was calculated using the following equation. In total, the sample solution (1 mL), 8 M urea (4 mL) and Ellman’s reagent (100 μL) were added. Afterward, the resulting solution was mixed well at 4°C for 2 h to measure the T-SH content. The solutions were measured at 412 nm by using a multifunctional enzyme-labeled instrument.
where A412 is the absorbance value, D is the dilution factor, C is the protein concentration of the sample, V is the assimilating protein solution volume, and ε is the extinction coefficient (13,600/M/cm).
Secondary structure: Fourier-transform infrared (FT-IR) spectroscopy absorption spectrum of the protein sample from 4,000 to 400 cm−1 was obtained using an FT-IR technology. The protein sample was produced by pressing in KBr windows (2 mg protein to 200 mg KBr) on a Carver press 4T pressure. The amide І bond in the region of 1,600–1,700 cm−1 was connected with stretching vibration of C=O bond and C-N of peptide bond. Secondary structural components of the protein were analyzed by the second-derivative analysis of the IR-SD after dealing with the linear baseline correction, the Fourier self-deconvolution, and the deconvoluted spectrum.
Thermal property: The thermal property of P. eryngii protein was measured adopting differential scanning calorimetry (DSC) (Lab Sys evo) according to the method of Zheng et al. with minor modifications.[Citation27] The protein sample was weighed in Al2O3 crucible and heated from 20°C to 500°C at 10°C/min, and an empty Al2O3 crucible was viewed as a reference. The peak temperature and total calorimetric enthalpy were calculated adopting the DSC data analysis software.
Effect of heat treatment and ultrasound treatment on physicochemical properties of protein: The protein was treated by heat at 75°C for 30 min and by ultrasound at 200 W for 30 min and then research the effect of heat treatment and ultrasound treatment on surface hydrophobicity, sulfhydryl content and the disulfide bond content, secondary structure, and thermal property.
Digestive properties of P. eryngii protein and the effect of treatments on protein
In vitro digestive procedure: The in vitro digestive model presents a three-step processing including the mouth, stomach, and small intestine according to Versantvoort et al.[Citation28] Digestion juices were prepared defiantly following and the in vitro digestive processing is described in . Before each experiment, the digestive juice is incubated at 37°C for 15 min. The vitro digestive is stimulated after adding saliva to the protein solution. Subsequently, gastric juices and intestinal fluids are mixed well in order to initiate the in vitro digestive processing in the stomach and intestine. Admittedly, the supernatant was collected after centrifugation so as to analyze digestive properties.
Table 1. Composition of digestive juices (500 mL) used for in vitro incubation of protein sample.
In vitro digestibility of the protein: The VDP was recorded using the method of the trichloroacetic acid (TCA-precipitation).[Citation29] The protein content was measured by using Coomassie brilliant blue method. The in vitro digestibility of protein was defined as follows: VDP = (C0-C1)/C0 × 100%, where C0 is referred to the TCA-precipitation protein content before the digestion (mg) and C1 is referred to the TCA-precipitation protein content after the digestion (mg).
Peptide contents of digestive juices: The peptides of protein after digesting in stomach and intestine were measured using the method of Lowery. The digestive solution 4 mL was collected and added to an equal volume of 10 g/L TCA, and then, the solution was centrifuged at 6,000 r/min for 15 min. The content of peptides in the supernatant was determined using the Lowry method.
Digestive juices DH: According to the method of ortho-phthaldialdehyde (OPA) by Mulcahy et al. with some changes,[Citation30] DH were determined by using the OPA method. The protein digestive products were diluted to 100 times with ultrapure water. Subsequently, the reaction solutions were measured at 340 nm using a multifunctional enzyme-labeled instrument (Spectra MX I3X). In order to obtain the content of AAG of protein according to the reference to 1-leucine standard curve (concentration range from 0.2 to 1.2 mM).
The digestive solution (2 mL) was transferred into a 10-mL volumetric flask and then re-added distilled water. The above solution was leached via 0.45 μm syringe filter and then transferred into a preparing injection bottle. At the same time, the standard sample was prepared as 5 mg/mL. The amino acid analysis operating condition: mobile phase – sodium citrate buffer solution, flow rate – 0.4 mL/min, injection volume – 20 μL, separation temperature – 57°C, reaction column temperature – 115°C, detection wavelength-valine detection wavelength is 440 nm, and other amino acid detection wavelength is 570 nm. The peak area was used as the test result, and the amino acid concentration was calculated using an external standard method.
Antioxidant activities of digestive juices: The DPPH radical scavenging activity of protein digestive products was determined as described in Ebru Pelvan Pelitli et al. with modifications.[Citation31] The test tube A0 (control group), 3.0 mL distilled water and 2 mL DPPH·solution were added. To test tube A (sample group), 3.0 mL sample solution and 2.0 mL DPPH·solution were added. To test tube A (sample control group), 3.0 mL sample solution and 2.0 mL absolute ethanol were added. Followed by adding the reaction mixture and mixing well, it reacted in dark condition at room temperature for 30 min, and subsequently, the reaction solutions were determined at 517 nm for A0, A, and B by using a multifunctional enzyme-labeled instrument (Spectra MX I3X). The DPPH radical scavenging activity was calculated using the following equation.
Reducing power activity assay: According to the method of Pelitli et al.,[Citation31] the reducing power activity of protein digestive products was measured. An protein digestive product solution 2 mL was mixed with 2 mL PBS (pH 6.6) and 2 mL of 1 g/L potassium ferricyanide. The mixture was reacted at 50°C for 20 min and then added 2 mL of 10 g/L trichloroacetic acid. Then, 2.0 mL of supernatant was chosen and mixed with 2.0 mL of distilled water and 0.4 mL of 0.1 g/L ferric chloride after centrifugation at 2,000 g for 10 min. Finally, the mixture was set at room temperature for 10 min and measured at 700 nm using a multifunctional enzyme-labeled instrument (Spectra MX I3X). The control group was the equivalent volume of distilled water rather than of the protein digestive product sample.
Superoxide anion radical activity: Superoxide anion radical was measured according to the method of Smirnoff N with some changes.[Citation32] All of the superoxide anion radical of protein digestive products were reacted. To test tube A0 (control group), 1.0 mL distilled water, 0.1 mL Pyrogallol of 60 mM, and 3.0 mL Tris-HCl of pH 8.2 were added. To test tube A (sample group), 1.0 mL sample solution, 0.1 mL Pyrogallol of 60 mM, and 3.0 mL Tris-HCl of pH 8.2 were added. To test tube B (sample control group), 3.1 mL sample solution and 1.0 mL sample solution were added. Followed by adding the reaction mixture and mixing well, it reacted at room temperature for 5 min, and subsequently, the reaction solutions were determined at 320 nm for A0, A, and B by using a multifunctional enzyme-labeled instrument (Spectra MX I3X). The superoxide anion radical activity was calculated using the following equation.
Hydroxyl radical-scavenging activity: The hydroxyl radical-scavenging activity was measured according to the method of Dai et al with minor modification.[Citation33] The hydroxyl radical was initiated by a Fenton reaction in a condition including FeSO4 and H2O2. All of the hydroxyl radical-scavenging activity of protein digestive products were reacted. To test tube A0 (control group), 1.0 mL distilled water, 0.3 mL salicylic acid of 20 mM, and 1.0 mL FeSO4 of 1.5 mM were added. To test tube A (sample group), 1.0 mL sample solution, 0.3 mL salicylic acid of 20 mM, and 1.0 mL FeSO4 of 1.5 mM were added. To test tube B (sample control group), 1.3 mL distilled water and 1.0 mL sample solution were added. Followed by adding the reaction mixture and mixing well, it reacted at 37°C for 30 min, and the absorbance was measured at 510 nm. The hydroxyl radical-scavenging activity was calculated using the following equation.
Effect of heat treatment and ultrasound treatment on digestive properties of the protein
The proteins were treated by heat at 75°C for 30 min and by ultrasound at 200 W for 30 min and then research the effect of heat treatment and ultrasound treatment on surface hydrophobicity after stimulating in stomach and intestinal of the protein, peptide contents and available amino groups, and antioxidant activities of digestive juices.
Statistical data analysis
All experimental data in this study were analyzed using Prism 5.0 and one-way analysis of F-test. Differences between variables with a P value of <0.05 were viewed as significant. All data were shown as mean ± standard deviation. In this study, all measurements were performed in triplicate.
Results and discussion
Physicochemical properties of Pleurotus eryngii protein
Rheological properties: Rheological properties of the protein solutions substantially affected the mechanical and film properties. We studied the rheological behavior of protein solutions to investigate the machine-processed effects of protein concentration on rheology. illustrates the viscosity (η) of different protein concentrations (3 g/100 g (raw materials/H2O)–9 g/100 g (raw materials/H2O)) at 25°C, and the viscosity of protein solutions formed at different concentrations was dependent on frequency. Higher viscosity was obtained at lower frequencies compared with those obtained at higher frequencies because, at longer experimental time scales, more interaction between proteins allows them to become stress-free during periodic deformation.[Citation34] As shown in , five distinct areas were found in the rheological curves: an apparent increase, two moderate increases, and two small increases between 3 g/100 g raw materials and 5 g/100 g raw materials, 5 g/100 g raw materials and 9 g/100 g raw materials, and 9 g/100 g raw materials and 11 g/100 g raw materials, which eventually tend to balance the state.
Figure 2. Viscosity (η) (a) and shear stress (b) of different protein concentrations (3 g/100 g (raw materials/H2O)–9 g/100 g (raw materials/H2O)) at 25°C.
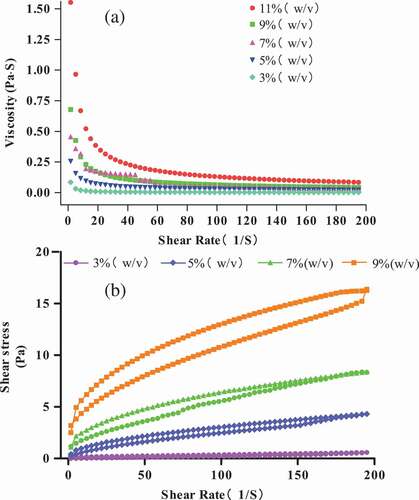
The hysteresis loop in the shear stress curves to shear rates is shown in , the hysteresis loop is in a clockwise direction, and the shear stress increases with the increase in protein solution concentrations. In the study of Liang, Zhang, and Xu,[Citation35] when the hysteresis loop was in a clockwise direction, the solution would form a new structure by shearing or breaking the structure. In addition, increasing protein concentrations can increase the closed loop area because the more closed the loop area, the more energy is needed to break down the structure at higher protein concentration.
Effects of temperatures on rheological property: shows the viscosity (η) of the 3 g/100 g raw material protein solution at different temperatures (25°C, 35°C, and 45°C). The previous researches showed that the SPI dispersion viscosity increased when heated because of the formation of new structures, hydrophobic interactions, and disulfide bridges.[Citation36] However, the protein solution viscosity decreased as the temperature increases in the present experiment. This result is in agreement with that of Liu, Xu, and Zhao.[Citation37]
Figure 3. Viscosity (η) (a) and shear stress (b) of 3 g/100 g (raw materials/H2O) protein solution at different temperatures (25°C, 35°C, and 45°C).
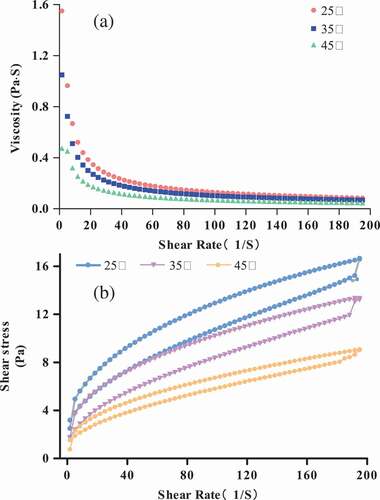
Similar to , shows the shear thinning behavior, and the protein solution viscosity reduces rapidly in the low-shear-rate range (<10 S−1). The apparent viscosity decreases with increasing temperature, resulting in more molecule chain motion at a higher temperature to obtain more energy. and show that the protein solution shear thinning behavior is the same as that of the common polymer solution, which dissolves P. eryngii proteins to form a steady protein solution.
In order to study the effects of temperature on the rheological behavior of P. eryngii protein solution, the 3 g/100 g raw material protein solution was selected. The shear stress curves and shear rates are shown in , which shows thixotropic behavior at different temperatures. As shown in the figure, the shear stress decreased with increasing temperature. The protein solution’s thixotropic property was lower at higher temperatures. This result indicated that the energy required to break down the thixotropic structure was lower at higher temperatures. This result is different from corn starch[Citation38] but is similar to soy protein isolate solution,[Citation37] which means that we can form gels in these systems and need more energy to destroy the structure.
Effects of pH on rheological property: The measurements were performed at different pH values (2.0, 3.5, 5.0, and 7.0) for the 15 g/100 g (raw materials/H2O) protein solution at 70°C. As shown in the mechanical spectrum curves can be observed. Differences can be observed at each pH. According to the previous results for the processing of gelation, the gel strength is lower in each case. In , G’ is always higher than G’’ at both pH levels; however, it has higher dependence of the modulus on frequency. We can observe a cross point at pH 2.0 and 5.0 (,), which means that a quite subtle gel was formed.[Citation12] The electrostatic interactions might play important roles in the protein system at pH 2.0 and 5.0, such as departing isoelectric point (pH 3.6), and the cross point was not observed at pH 3.5 and 7.0. At pH 7.0, G’ and G’’ are very higher compared with those in other pH levels, which showed an evident increase in the protein solution. The protein solutions showed that G’ is twice higher than that in other cases, which means that it cannot form a gel. At pH 3.5, G’ and G’’ are almost parallel, which indicates the formation of gel.
Figure 4. Modulus of 15 g/100 g (raw materials/H2O) protein solution at different pH at 70°C. (a) Modulus of 15 g/100 g (raw materials/H2O) protein solution at pH 2.0. (b) Modulus of 15 g/100 g (raw materials/H2O) protein solution at pH 5.0.
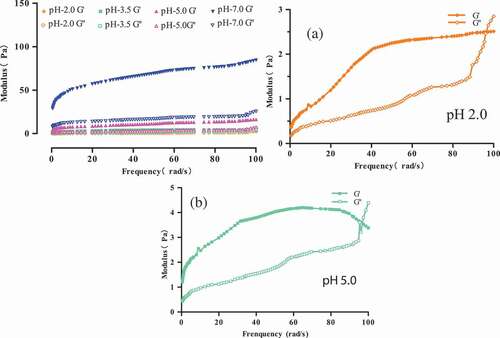
Effects of pH on sulfhydryl and disulfide bond contents are shown in . The content of sulfhydryl bonds (R-SH and T-SH) increases and the content of disulfide bonds increases with pH increase. These results indicated that the content of disulfide bond might be associated with the aggregation of protein due to the formation of chain-chain.[Citation39] In other words, the increase in the disulfide bond might lead to network formation. The connections between physicochemical and rheological properties are shown in . As summarized above, the sulfhydryl and disulfide bond contents were significantly related to the rheological properties of proteins. The sulfhydryl content was correlated with the reduction in η, meaning that the protein might be held closer with higher sulfhydryl bond content and be less coacervation with a higher disulfide bond.
Table 2. Effect of pH on the sulfhydryl and disulfide bond contents.
Table 3. Connection between physicochemical and rheological properties.
The Power Law model demonstrates flow behavior of each protein sample, and the n manifests the deviation degree from Newtonian fluid. From , the n value was lower near the protein isoelectric point and increased deviating from the isoelectric point at different pH concentrations, and it is reported that the viscosity of Newtonian fluid retains constant with strengthening shear rate when n = 1, and the less the n value, the more the degree of deviation from Newtonian fluid, which indicates that the fluid viscosity is more decided by shear rate. At the same pH concentrate, the n value decreased with increasing temperature; this result might be that by improving the moving ability of protein–protein molecules, the viscosity dependence to shear rate decreases, and the higher the temperature, the more the energy enabled to the breakage of entanglements.
Table 4. The Power Law model of protein in different conditions.
Effects of Ca2+ on rheological property: shows the comparison between the evolution of G’ at 0.5 Hz for the 15 g/100 g raw material protein solution with different temperatures by adding different concentrations of Ca2+ (0.01, 0.03, and 0.05 mol/L). Dynamic rheology has been used to describe the heat-induced gelation of proteins, which is usually present in G’ because G’ plays a representative role in the recovered per cycle of sinusoidal shear deformation.[Citation40] In all cases, G’ decreased sharply as the temperature was raised from 25°C to 65°C. In the recovered proteins, G’ was initially increased at approximately 25°C, emerged a peak at 28°C, and reduced to minimum at 65°C without Ca2+. However, the temperature of the emerging peak was decreased. When the Ca2+ concentration was 0.01 mol/L, the peak corresponding to the temperature was approximately 36°C, and when the Ca2+ concentrations were 0.03 and 0.05 mol/L, the peaks corresponding to the temperature were both approximately 42°C; G’ increased at first, which might be related to the cross-linking of proteins, and then, it subsequently decreased, which might be due to the slight denaturation of proteins. Above of that G’ of proteins gradually increased with increasing calcium ionic strength because the system was much more complex with the bio-polymer competition for Ca2+, and when the calcium ionic strength was lower, the interaction between Ca2+ and proteins was relatively weak, leading to lower G’. By contrast, the binding force between the calcium ions and proteins was strong, allowing the protein system to substantially depend on calcium ions.[Citation41]
Surface hydrophobicity (H0)
The surface hydrophobicity (H0) plays one of the leaders in conformation and interaction of protein molecule so as to evaluate the change in protein structure and reflect the number of hydrophobic amino acids on the protein surface.[Citation42] As can be observed from , protein through heat treating and ultrasound treating was characterized by higher H0 than non-treating protein significantly (P < 0.05). This result was similar to that of the previous research like bovine serum albumin[Citation43] and black bean protein isolate.[Citation44] This increase in H0 of protein might mean that the hydrophobic amino acids were exposed to the surface from inside of protein molecules because of generating the cavitation phenomenon triggered by ultrasound and the unfolding of protein molecules under heat treating.[Citation45,Citation46]
Contents of sulfhydryl and disulfide bond
The reactive sulfhydryl content (R-SH) has a significant effect on food protein functional property. The change in R-SH of protein result in sonication is shown in . As observed from , heat treatment increases significantly the R-SH content of protein (P < 0.05); this might be due to heat movement inducing heat treatment, thus exposing more R-SH.[Citation46] Ultrasound treatment increased significantly (P < 0.05) the R-SH and T-SH contents of protein and decreased significantly (P < 0.05) the S-S content, and these results mean that ultrasound treatment might promote protein unfold and break the disulfide bonds, making more decrease in the content of SH, and protein through ultrasound treating could expose potential buried sulfhydryl groups into the protein surface due to the cavitation phenomenon.[Citation45]
Table 5. The contents of sulfhydryl and disulfide bond of protein treated by heat and ultrasound.
Secondary structure
FT-IR spectroscopy is used to determine the secondary structure of the protein in a wide variety of situation, and the amide I region of protein FT-IR is connected with the C=O and C-N stretching vibration.[Citation47] The FT-IR spectra of protein in the region of 400–4,000 cm−1 are shown in , and the results presented that the absorption region and peak shape of protein were not dramatically different between the control group and treated group including heat treating and ultrasound treating; however, we can observe that the absorption strength of peak of protein increased through heat treating and ultrasound treating. The fitted peaks of protein are presented in by analyzing the deconvolution and the overlapping, and the specific distribution of the secondary structure of protein is shown in ; the heat and ultrasound pretreatment increased the content of α-helix and decreased the content of β-sheet and random coil in protein, while these decreased by heat treating and increased by ultrasound heating in β-turn of protein compared with control group. The changes in the secondary structure of protein might be owing to the acoustic cavitation brought by ultrasound like shear force, shock waves, and others[Citation18] and the rearrangement of the inter-linkage between molecules by heating pretreatment[Citation16] (Girgih A T, Chao D & Lin L, 2015), which might have disrupted the order of local amino acids, thus leading to protein molecule rearrange. These results were identical with Liu et al.[Citation48] while contradictory with Jin et al., which found that the zein treated by ultrasound and the secondary structure of protein presented that the ultrasound treatment increased the content of β-sheet.[Citation42] The result was different from that of other studies due to the discrepancy in ultrasound environment like the ultrasound frequency and the method of heat treating.
Table 6. Secondary structure of the Pleurotus eryngii protein.
Thermal properties
Protein features heat resistance at a certain temperature, and the maintenance force of spatial conformation of a protein can be changed under the situation that the outside temperature exceeds its initial denaturation temperature (Tm), thus leading to protein denaturation. shows the DSC and Tp analyses of P. eryngii protein treated by heat and ultrasound; as can been observed from and , the Tm of protein under untreated, heat treated, and ultrasound treated was A, respectively. These results might due to the different hydrophobicity of protein through different treating. The higher the hydrophobicity, the more stable the protein because the hydrophobic interaction was endothermic, thereby increasing the denaturation temperature. Moreover, the enthalpy change (△H) of untreated protein was higher compared with treated by hreat and ultrasound, which might be owing to the different content of disulfide bonds, and the more the disulfide bonds, the greater destroying energy was required. However, there are few researches concentrated on this aspect presently, and the specific mechanism is not clear yet and further research must be needed.
Table 7. The denaturation temperature (Tm) and enthalpy change (△H) of Pleurotus eryngii protein.
Digestive properties of P. eryngii protein and the effect of treatments on protein
Available amino groups (DH) of protein and in vitro digestibility of digestive juice (VDP): As shown in , DH and VDP of different kinds of protein increased with digestion method (stomach digestion and small intestinal digestion). The DH and VDP of protein by trypsin in the intestinal phase were significantly higher than by pepsin in the gastric phase (P < 0.05), and this was because protein in the intestinal digestion was more digestive than in the gastric phase, and trypsin in the small intestinal could break peptide bonds more easier than pepsin in the stomach.[Citation33] According to the previous research, the increased digestibility of heated and ultrasound-treated protein was connected with an increase in protein hydrophobic surface (H0) because the enhancement of H0 could provide more access to proteases that laminate at hydrophobic amino acid residues, thus promoting protein digestibility of heated and ultrasound-treated protein compared to non-treated protein.[Citation49]
Peptides and amino acids of protein digestive juice
As shown in , the peptide content of protein digestive juice was different via different treatment methods. The peptide content of protein digestive products through pepsin digested and intestinal digested was reduced under heat treatment and ultrasoundtreatment of protein, but the effect was significant under the ultrasound treatment (P < 0.05). The amino acid composition of protein digestive products via treating with different methods was shown in . We could observe the difference in composition and ratio of amino acids through treating with different methods (the amino acid content was calculated using standard amino acid samples). In non-treatment group and heat treatment group, five kinds of amino acids were shown, and there are 10 kinds of amino acids in the ultrasound treatment group. These results indicate that protein treated by heat and ultrasound changed the protein structure, promoting the protein digestion, which was consistent with the above results of DH and VDP. However, the systematic research was needed due to the different functions of peptides and amino acids.
Table 8. Amino acid composition of protein digestive juice.
Absorption intrinsic of P. eryngii protein and protein digestive products
The absorption intrinsic of P. eryngii protein and protein digestive products was measured to investigate the change in different kinds of protein spatial structures after heat-treated and ultrasound pre-heated. The intrinsic fluorescence spectrum represents the Trp residues' characteristic peaks excited at 360 nm, and the change of peak position and peak strength reflects the change of Trp residues. The intrinsic fluorescence spectrum of protein and protein digestive products was post and reflected in , and the fluorescence intensity of protein (FIP) and protein digestive products (FIPD) was changed by treating method, and the Trp residues' characteristic peak of protein and protein digestive products was not changed. The FIP and FIDP through heat-treated and ultrasound pre-heated intensified compared to the control group and the enhancement of FIP and FIDP by heating treat surpassed by ultrasound pre-treated; this is because ultrasound pre-treated might destroy the interactions of protein–protein, thereby causing the structure change of protein molecules and making more Trp residues being exposed from inside to outside, lead to the FIP and FIDP increase.[Citation42] In addition, we could observe that the FIDP was more higher than FIP and FIDP through intestinal digestive (FIDP-2) was more higher than stomach digestive (FIDP-1), and as observed in , these results indicated that the micro-condition of Trp residues changed; more Trp residues were transferred from molecule inside to outside. In a word, the protein might be more digestible in the small intestine than stomach, and this might be due to trypsin intestine juice could disrupt more easily on peptide bonds than pepsin stomach juice.[Citation33]
Antioxidant activities of protein digestive products
As shown in , it reflected majorly the antioxidant activity of protein digestive products, which presented higher antioxidant activity through in vitro digestive including pepsin-digested and intestinal-digested except DPPH· free radicals characterized with decreasing. In other words, the ability of protein and the trypsin digest for DPPH· radicals was lower than the pepsin digest. However, in contrast to Wang et al.'s research,[Citation50] this result showed that DPPH· was an oil-soluble free-radical, which can make a more stable product by obtaining an electron. After protein and pepsin interacted with each other in stomach phase, more hydrophobic amino acids were appeared, making protein digestive products more accessible by DPPH·, and this was to promote electron divert from protein digestive products, thus stabilizing DPPH·. However, when the protein further digested with trypsin in the intestinal phase, the peptide bonds were cracked, which forming many shorter peptides like tripeptides and dipeptides and more amino acids, thus leading to less effect of lipid-soluble DPPH· on amino acids and shorter peptides; therefore, the scavenging capacity of DPPH· free radical was different in the two complex digestive systems.[Citation51] This result was concurred with Liu et al.[Citation52] Effects of digestive on superoxide anion radical (O2−) and hydroxyl radical (·OH) and reducing power activities of a protein are presented in . The scavenging activities of protein digestive products were stimulated to increase digesting times presented a regular profile even if treated by different methods, which scavenging superoxide anion and radical hydroxyl radical and reducing power activities were increased after treatment by pepsin for 1 h and then by pancreatin for 4 h. It was worth mentioning that the scavenging activities like superoxide anion, radical hydroxyl radical, and reducing power were higher in pepsin phase than in the intestinal phase. In other words, the antioxidant activities of protein digestive products were also remained in the intestine because the digests after further digestive with trypsin also featured better antioxidant activities. These results were consistent with Rao et al.[Citation53] And it reached a steady state after about 4 h in the intestinal digestive system, which the scavenging superoxide anion, radical hydroxyl radical, and reducing power activities reached. The scavenging activities of protein digestive products treated by heating and ultrasound were higher than non-treated. This result was because that ultrasound pre-treated might cause ultrasound cavitation effect, which could bring a huge shear force and destroy the interactions of protein–protein, thus changing the protein molecule structure and exposing more cleavage sites,[Citation42] which was conducive to the combination of protein and enzyme; therefore, the peptides after digestive presented higher antioxidant activities like superoxide anion radical, hydroxyl radical, and reducing power. Moreover, heat-treat might expose cleavage sites, resulting in the movement of protein–protein molecules, thereby promoting the antioxidant capacity of protein digestive products.
Conclusion
Protein concentration and temperature in the solution system played an important role in the rheological properties and viscosity. The shear stress and viscosity increased as the protein concentration increased from 3 g/100 g raw materials to 9 g/100 g raw materials and decreased as temperature increased from 25°C to 45°C. In addition, pH from 2.0 to 7.0 significantly affects the content of disulfide bond and sulfhydryl bond, which showed that the lowest of disulfide bond content at pH 3.5 and higher of sulfhydryl bond at pH 7.0. The addition of calcium from 0.01 to 0.05 mol/L to the protein system could increase the rheology properties. The protein treated by heat and ultrasound increased significantly the surface (H0) (P < 0.05) and enhanced terminal stability of the protein. The DH and VDP under pre-treatment were higher significantly than non-treatment (P < 0.05), and the kinds of essential amino acids increased. Moreover, heat treatment and ultrasound treatment can enable to unfold structure, which promoted the formation of well-digestible power of protein with excellent functional properties. In addition, the protein digestive products feature strong antioxidant activities, but the DPPH· free radicals characterized with decreasing through pepsin-digestible and intestinal-digestible. Therefore, based on these results of this research, heat treatment and ultrasound treatment is an effective processing way that promotes the digestibility of protein and cultivates its nutritional value.
Additional information
Funding
References
- Chen, J.; Yang, Y.; Weng, M.; Lai, P.; Shen, H. Optimization of Combined Hot-Air and Vacuum Drying Technology for Instant Pleurotus eryngii. Trans. Chin. Soc. Agric. Eng. 2014, 30(14), 331–338.
- Li, S.; Shah, N. P. Antioxidant and Antibacterial Activities of Sulphated Polysaccharides from Pleurotus eryngii and Streptococcus thermophilus ASCC 1275. Food Chem. 2014, 262–270. doi:10.1016/j.foodchem.2014.05.110.
- Mariga, A. M.; Yang, W.; Mugambi, D. K.; Pei, F.; Zhao, L.-Y.; Shao, Y.-N.; Hu, Q. Antiproliferative and Immunostimulatory Activity of a Protein from Pleurotus eryngii. J. Sci. Food Agric. 2014, 94(15), 3152–3162. DOI: 10.1002/jsfa.2014.94.issue-15.
- Yang, Z.; Xu, J.; Fu, Q.; Fu, X.; Shu, T.; Bi, Y.; Song, B. Antitumor Activity of a Polysaccharide from Pleurotus eryngii on Mice Bearing Renal Cancer. Carbohydrate Polymers. 2013, 95(2), 615–620. DOI: 10.1016/j.carbpol.2013.03.024.
- Sun, Y.; Li, W. Activity-Guided Isolation and Structural Identification of Immunomodulating Substances from Pleurotus eryngii, Byproducts. Int. Immunopharmacol. 2017, 51, 82–90. DOI: 10.1016/j.intimp.2017.08.005.
- Bergendiova, K.; Tibenska, E.; Majtan, J. Pleuran (Î2-Glucan from Pleurotus ostreatus) Supplementation, Cellular Immune Response and Respiratory Tract Infections in Athletes. Eur. J. Appl. Physiol. 2011, 111(9), 2033–2040. DOI: 10.1007/s00421-011-1837-z.
- Ma, G.; Yang, W.; Mariga, A. M.; Fang, Y.; Ma, N.; Pei, F.; Hu, Q. Purification, Characterization and Antitumor Activity of Polysaccharides from Pleurotus eryngii Residue. Carbohydr. Polym. 2014, 114, 297–305. DOI: 10.1016/j.carbpol.2014.07.069.
- Sun, Y.; Hu, X.; Li, W. Antioxidant, Antitumor and Immunostimulatory Activities of the Polypeptide from Pleurotus eryngii Mycelium. Int. J. Biol. Macromol. 2017, 97, 323. DOI: 10.1016/j.ijbiomac.2017.01.023.
- Shimizu, K.; Yamanaka, M.; Gyokusen, M.; Kaneko, S.; Tsutsui, M.; Sato, J.; Sato, I.; Sato, M.; Kondo, R. Estrogen-Like Activity and Prevention Effect of Bone Loss in Calcium Deficient Ovariectomized Rats by the Extract of Pleurotus eryngii. Phytotherapy Res. 2006, 20(8), 659. DOI: 10.1002/(ISSN)1099-1573.
- Wang, B.; Timilsena, Y. P.; Blanch, E.; Adhikari, B. Mild Thermal Treatment and In-Vitro Digestion of Three Forms of Bovine Lactoferrin: Effects on Functional Properties. Int. Dairy J. 2017, 64, 22–30. DOI: 10.1016/j.idairyj.2016.09.001.
- Tadpitchayangkoon, P.; Yongsawatdigul, J. Comparative Study of Washing Treatments and Alkali Extraction on Gelation Characteristics of Striped Catfish (Pangasius Hypophthalmus) Muscle Protein. J. Food Sci. 2009, 74, 3. DOI: 10.1111/j.1750-3841.2009.01110.x.
- Felix, M.; Romero, A.; Guerrero, A. Development and Evaluation of Rheological and Bioactive Properties of Rice Protein-Based Gels. J. Cereal Sci. 2016, 91–100. DOI:10.1016/j.jcs.2016.10.004.
- Donato, L.; Garnier, C.; Novales, B.; Doublier, J. L. Gelation of Globular Protein in Presence of Low Methoxyl Pectin: Effect of Na+, and/or Ca2+, Ions on Rheology and Microstructure of the Systems. Food Hydrocolloids. 2005, 19(3), 549–556.
- Arkell, A.; Krawczyk, H.; Jönsson, A. S. Influence of Heat Pretreatment on Ultrafiltration of a Solution Containing Hemicelluloses Extracted from Wheat Bran. Sep. Purif. Technol. 2013, 119(46), 46–50. DOI: 10.1016/j.seppur.2013.09.001.
- Girgih, A. T.; Chao, D.; Lin, L.; He, R.; Jung, S.; Aluko, R. E. Enzymatic Protein Hydrolysates from High Pressure-Pretreated Isolated Pea Proteins Have Better Antioxidant Properties than Similar Hydrolysates Produced from Heat pretreatment[J]. Food Chem. 2015, 188, 510–516. DOI: 10.1016/j.foodchem.2015.05.024.
- Girgih, A. T.; Chao, D.; Lin, L.; He, R.; Jung, S.; Aluko, R. E. Enzymatic Protein Hydrolysates from High Pressure-Pretreated Isolated Pea Proteins Have Better Antioxidant Properties than Similar Hydrolysates Produced from Heat Pretreatment. Food Chem. 2015, 188, 510–516. DOI: 10.1016/j.foodchem.2015.05.024.
- Chemat, F.; Rombaut, N.; Sicaire, A. G.; Meullemiestre, A.; Fabiano-Tixier, A.-S.; Abert-Vian, M. Ultrasound Assisted Extraction of Food and Natural Products. Mechanisms, Techniques, Combinations, Protocols and Applications. A Review. Ultrason. Sonochem. 2017, 34, 540. DOI: 10.1016/j.ultsonch.2016.06.035.
- Hu, H.; Wu, J.; Li-Chan, E. C. Y.; Zhu, L.; Zhang, F.; Xu, X.; Fan, G.; Wang, L.; Huang, X.; Pan, S. Effects of Ultrasound on Structural and Physical Properties of Soy Protein Isolate (Spi) Dispersions. Food Hydrocolloids. 2013, 30(2), 647–655. DOI: 10.1016/j.foodhyd.2012.08.001.
- Liang, Q.; Ren, X.; Ma, H.; Li, S.; Xu, K.; Oladejo, A. O. Effect of Low-Frequency Ultrasonic-Assisted Enzymolysis on the Physicochemical and Antioxidant Properties of Corn Protein Hydrolysates[J]. J. Food Qual. 2017, 4, 1–10. DOI: 10.1155/2017/2784146.
- Ren, X.; Ma, H.; Mao, S.; Zhou, H. Effects of Sweeping Frequency Ultrasound Treatment on Enzymatic Preparations of Ace-Inhibitory Peptides from Zein. Eur. Food Res. Technol. 2014, 238(3), 435–442. DOI: 10.1007/s00217-013-2118-3.
- Zisu, B.; Bhaskaracharya, R.; Kentish, S.; Ashokkumar, M. Ultrasonic Processing of Dairy Systems in Large Scale Reactors. Ultrason. Sonochem. 2010, 17(6), 1075. DOI: 10.1016/j.ultsonch.2009.10.014.
- Shi, R. J.; Guo, F. M.; Feng, C. P. Extracting Pleurotus eryngii Protein by Combining Cellulose Enzymatic Hydrolysis and Acid Precipitation after Alkaline Extraction. Edible Fungi China. 2018, 36(6), 58–63.
- Wang, J.; Chi, Y.; Cheng, Y.; Zhao, Y. Physicochemical Properties, in Vitro Digestibility and Antioxidant Activity of Dry-Heated Egg White Protein. Food Chem. 2018, 246, 18. DOI: 10.1016/j.foodchem.2017.10.128.
- Liu, Y.; Zhao, G.; Zhao, M.; Ren, J.; Yang, B. Improvement of Functional Properties of Peanut Protein Isolate by Conjugation with Dextran through Maillard Reaction. Food Chem. 2012, 131(3), 901–906. DOI: 10.1016/j.foodchem.2011.09.074.
- Omana, D. A.; Plastow, G.; Betti, M. The Use of β-glucan as a Partial Salt Replacer in High Pressure Processed Chicken Breast Meat. Food Chem. 2011, 129(3), 768–776.
- Zhou, C.; Ma, H.; Yu, X.; Liu, B.; Ael-G, Y.; Pan, Z. Pretreatment of Defatted Wheat Germ Proteins (By-Products of Flour Mill Industry) Using Ultrasonic Horn and Bath Reactors: Effect on Structure and Preparation of Ace-Inhibitory Peptides. Ultrason. Sonochem. 2013, 20(6), 1390–1400. DOI: 10.1016/j.ultsonch.2013.04.005.
- Zheng, X. Q.; Wang, J. T.; Liu, X. L.; Sun, Y.; Zheng, Y. J.; Wang, X. J.; Liu, Y. Effect of Hydrolysis Time on the Physicochemical and Functional Properties of Corn Glutelin by Protamex Hydrolysis. Food Chem. 2015, 172(172), 407–415.
- Versantvoort, C. H.; Oomen, A. G.; Van, D. K. E.; Rompelberg, C. J.; Sips, A. J. Applicability of an in Vitro Digestion Model in Assessing the Bioaccessibility of Mycotoxins from Food. Food Chem. Toxicol. 2005, 43(1), 31–40. DOI: 10.1016/j.fct.2004.08.007.
- Fan, D. X.; Li, J. J.; Yang, J. Q.; Shen, Q. Effects of Heat Treatments on the in Vitro Digestibility of Millet Protein. J. Chin. Inst. Food Sci. Technol. 2016, 16(02), 56–61.
- Mulcahy, E. M.; Fargierlagrange, M.; Mulvihill, D. M.; O’Mahony, J. A. Characterisation of Heat-Induced Protein Aggregation in Whey Protein Isolate and the Influence of Aggregation on the Availability of Amino Groups as Measured by the Ortho-Phthaldialdehyde (OPA) and Trinitrobenzene- -Ulfonic Acid (Tnbs) Methods. Food Chem. 2017, 229, 66–74. DOI: 10.1016/j.foodchem.2017.01.155.
- Pelvan, P. E.; Janiak, M. A.; Amarowicz, R.; Alasalvar, C. Protein Precipitating Capacity and Antioxidant Activity of Turkish Tombul Hazelnut Phenolic Extract and Its Fractions. Food Chem. 2017, 218, 584–590. DOI: 10.1016/j.foodchem.2016.09.070.
- Smirnoff, N.; Cumbes, Q. J. Hydroxyl Radical Scavenging Activity of Compatible Solutes. Phytochemistry. 1989, 28, 1057–1060. DOI: 10.1016/0031-9422(89)80182-7.
- Dai, C.; Zhang, W.; He, R.; Xiong, F.; Ma, H. Protein Breakdown and Release of Antioxidant Peptides during Simulated Gastrointestinal Digestion and the Absorption by Everted Intestinal Sac of Rapeseed Proteins. LWT – Food Sci. Technol. 2017, 86, 424–429. DOI: 10.1016/j.lwt.2017.08.026.
- Renkema, J. M.; Gruppen, H.; Van Vliet, T. Influence of pH and Ionic Strength on Heat-Induced Formation and Rheological Properties of Soy Protein Gels in Relation to Denaturation and Their Protein Compositions. J. Agric. Food Chem. 2002, 50(21), 6064–6071.
- Liang, Q.; Zhang, J.; Xu, C.; Dou, J.; Zhang, S. Influence of Temperature, pH, and Ionic Strength on the Rheological Properties of Oviductus ranae Hydrogels. Afr. J. Biotechnol. 2014, 13(24), 2435–2444.
- Wagner, J. R.; Sorgentini, D. A.; Anon, M. C. Effect of Physical and Chemical Factors on Rheological Behavior of Commercial Soy Protein Isolates: Protein Concentration, Water Imbibing Capacity, Salt Addition, and Thermal Treatment. J. Agric. Food Chem. 1992, 40(10), 1930–1937. DOI: 10.1021/jf00022a041.
- Liu, P.; Xu, H.; Zhao, Y.; Yang, Y. Rheological Properties of Soy Protein Isolate Solution for Fibers and Films. Food Hydrocolloids. 2017, 149–156. doi:10.1016/j.foodhyd.2016.11.001.
- Dokic, L.; Dapcevic, T.; Krstonosic, V.; Dokić, P.; Hadnađev, M. Rheological Characterization of Corn Starch Isolated by Alkali Method. Food Hydrocolloids. 2010, 172–177. DOI:10.1016/j.foodhyd.2009.09.002.
- Shiau, S.; Yeh, A. Effects of Alkali and Acid on Dough Rheological Properties and Characteristics of Extruded Noodles. J. Cereal Sci. 2001, 33(1), 27–37. DOI: 10.1006/jcrs.2000.0344.
- Tahergorabi, R.; Sivanandan, L.; Jaczynski, J. Dynamic Rheology and Endothermic Transitions of Proteins Recovered from Chicken-Meat Processing By-Products Using Isoelectric Solubilization/Precipitation and Addition of TiO2. LWT – Food Sci. Technol. 2012, 46(1), 148–155.
- Beaulieu, M.; Turgeon, S. L.; Doublier, J. L. Rheology, Texture and Microstructure of Whey Proteins/Low Methoxyl Pectins Mixed Gels with Added Calcium. Int. Dairy J. 2001, 11(11–12), 961–967. DOI: 10.1016/S0958-6946(01)00127-3.
- Jin, J.; Ma, H.; Wang, B.; Aelg, Y.; Wang, K.; He, R.; Zhou, C. Effects and Mechanism of Dual-Frequency Power Ultrasound on the Molecular Weight Distribution of Corn Gluten Meal Hydrolysates. Ultrason. Sonochem. 2016, 30, 44. DOI: 10.1016/j.ultsonch.2015.11.021.
- Gülseren, I.; Güzey, D.; Bruce, B. D.; Weiss, J. Structural and Functional Changes in Ultrasonicated Bovine Serum Albumin Solutions. Ultrason. Sonochem. 2007, 14(2), 173–183. DOI: 10.1016/j.ultsonch.2005.07.006.
- Jiang, L.; Wang, J.; Li, Y.; Wang, Z.; Liang, J.; Wang, R.; Chen, Y.; Ma, W.; Qi, B.; Zhang, M. Effects of Ultrasound on the Structure and Physical Properties of Black Bean Protein Isolates. Food Res. Int. 2014, 62(6), 595–601. DOI: 10.1016/j.foodres.2014.04.022.
- Jian, J.; Haile, M.; Wenjuan, Q.; Kai, W.; Cunshan, Z.; Ronghai, H.; Yagoub A. E. G. A.; Owusu J.; Ye, X. Effects of Multi-Frequency Power Ultrasound on the Enzymolysis of Corn Gluten Meal: Kinetics and Thermodynamics Study. Ultrason. Sonochem. 2015, 27, 46–53. DOI: 10.1016/j.ultsonch.2015.04.031.
- Ivander, P.; Avan, L.; Hendrickx, M. E. Effect of Heat-Treatment on the Physico-Chemical Properties of Egg White Proteins: A Kinetic Study. J. Food Eng. 2006, 75(3), 316–326. DOI: 10.1016/j.jfoodeng.2005.04.019.
- Pandya, A.; Sutariya, P. G.; Menon, S. K. Protein Mediated Synthesis of Gold Nanobiocatalyst by Microwave: A High Efficient Catalytic Activity for the Selective Oxidation of Benzyl Alcohol. J. Mol. Catal. A. Chem. 2013, 380(4), 78–83. DOI: 10.1016/j.molcata.2013.08.022.
- Liu, B.; Ma, H. L.; Li, S. J.; Tian, W. M.; Wu, B. G. Study on the Structure of Defatted Wheat Germ Protein Isolate under Ultrasonic Treatment]. Spectrosc. Spectral Anal. 2011, 31(8), 2220.
- Lassé, M.; Deb-Choudhury, S.; Haines, S.; Larsen, N.; Gerrard, J. A.; Dyer, J. M. The Impact of pH, Salt Concentration and Heat on Digestibility and Amino Acid Modification in Egg White Protein. J. Food Compost. Anal. 2015, 38, 42–48. DOI: 10.1016/j.jfca.2014.08.007.
- Wang, Z.; Liu, Y.; Li, H.; Yang, L. Rice Proteins, Extracted by Alkali and α-amylase, Differently Affect in Vitro Antioxidant Activity. Food Chem. 2016, 206, 137–145. DOI: 10.1016/j.foodchem.2016.03.042.
- Zhu, L.; Chen, J.; Tang, X.; Xiong, Y. L. Reducing, Radical Scavenging, and Chelation Properties of in Vitro Digests of Alcalase-Treated Zein Hydrolysate. J. Agric Food Chem. 2008, 56(8), 2714–2721. DOI: 10.1021/jf703697e.
- Liu, Y.; Wang, Z.; Li, H.; Liang, M.; Yang, L. In Vitro Antioxidant Activity of Rice Protein Affected by Alkaline Degree and Gastrointestinal Protease Digestion. J. Sci. Food Agric. 2016, 96(15), 4940–4950. DOI: 10.1002/jsfa.2016.96.issue-15.
- Rao, S.; Sun, J.; Liu, Y.; Zeng, H.; Su, Y.; Yang, Y. Ace Inhibitory Peptides and Antioxidant Peptides Derived from in Vitro, Digestion Hydrolysate of Hen Egg White Lysozyme. Food Chem. 2012, 135(3), 1245–1252. DOI: 10.1016/j.foodchem.2012.05.059.