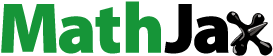
ABSTRACT
The effects of cysteine, initial pH, and thermal treatment on the color and flavor derived from Maillard reaction of flaxseed protein hydrolysates were investigated. Browning and color of Maillard reaction products (MRPs) were inhibited at higher cysteine concentration and lower initial pH. Higher cysteine or initial pH suppressed the cross-linking of 128–1,000 Da peptides and accelerated the degradation of peptides with a higher molecular weight (MW) (>1,000 Da). The higher temperature and longer reaction time resulted into a marked increase in flavor formation in the Maillard reaction model. Partial least squares regression (PLSR) revealed that volatile compounds, MW distribution, and amino acids of MRPs had a significant influence on MRPs sensory characteristics. The generation of umami, mouthfulness, and continuity was observed at relatively lower temperatures and longer heating time. The sulfur- and nitrogen-containing compounds from the low MW peptides (<1,000 Da) may lead to meaty aroma in MRPs.
Introduction
Since long ago, seeds from flax (Linum usitatissimum L.) have been used in the food industry due to the abundance of α-linolenic acid in their oil content.[Citation1,Citation2] Flaxseed meal is a protein-rich by-product obtained during flaxseed oil extraction.[Citation3] Nowadays, there are large number of flax plantations in Russia, Canada, United States, and China. In fact, in many European countries, bakers often use this grain to make food, such as biscuits, cakes, breads, and so on. The Maillard reaction is a thermally induced phenomenon takes place most often at high temperature. Till date, research focus has been primarily directed towards implications of the reaction on food product qualities (browning and flavor formation) in food industries. The Maillard reaction is an important series of reactions for flavor and color formation in foods which has a primary role in the transformation of raw food material into more appetizing foods with a variety of aromas after thermal processing. Recently, it was proved that this reaction can also affect the nutritional quality of food. It is thought to be responsible for the appealing flavors of many thermally processed foods, such as cocoa, coffee, bread, meat, and other savory flavors. Similarly, color formation (browning) can be acceptable, for example in baking and roasting (coffee, cocoa, bread), or undesirable, in spray-dried milk and egg or dehydrated products. Several studies focused on the effect of Maillard chemistry on the nutritional impact such as formation of mutagenic and antioxidant Maillard Reaction Products (MRPs) as well as on human physiological conditions related to protein modification and other pathological complications.[Citation4,Citation5]
Color and flavor formation in the Maillard reaction are influenced by the different factors such as pH, time, temperature, sugar type, type of amino acid residue, water activity/moisture content, reactant-type, and concentration.[Citation6] Consequently, the optimization of the reaction to produce the desired results is a challenging task. Normally, studies evaluating the influence of these parameters are conducted mostly on simple model systems comprising of amino acids and sugars as it is easier to manipulate and interpret the mechanisms of MRPs formation. These factors influenced the reaction conditions on volatile-flavor generation, as numerous MRPs are known to contribute to perceived flavors in food products. Recently, we reported the enzymolysis properties of flaxseed meal and its uses to prepare MRPs.[Citation7] However, many important factors affecting the Maillard reaction, for instance cysteine addition, initial pH, and thermal treatment were not considered in our previous report. Therefore, previously obtained flaxseed protein hydrolysate was used for the development of MRPs and the corresponding effects of cysteine addition, initial pH, and thermal treatment on sensory characteristics were assessed.
Materials and methods
Preparation of MRPs
As reported in our recent study on flaxseeds (Linum usitatissimum L.), previously obtained flaxseed protein hydrolysates were used in the current study as starting material.[Citation7] For the Maillard reaction, three main ingredients such as 2.0 g of flaxseed protein hydrolysates, 1.0 g of D-xylose, and L-cysteine (0.05–0.25 g) were mixed with distilled water (DW) to obtain 10% (w/v) as the final concentration followed by initial pH adjustment (6.5–8.5). The above suspensions were allowed to stand in a thermostatic oil bath (100–140°C) with magnetic stirring for 0–180 min followed by immediate cooling.
Composition and browning intensity
Moisture, fat, protein, total sugar, crude fiber, and ash were analyzed according to AOAC Method (2005).[Citation8] Total sugar was analyzed according to Li et al.[Citation9] For sample preparation, same procedure was followed as mentioned in section 2.1. The freshly prepared samples were diluted 50-fold by using DW and the absorbance was measured at 420 nm and 294 nm representing the browning index.[Citation10]
Color measurement
The following CIE Lab parameters were obtained: ΔL* (lightness), Δa* (+ red, – green) and Δb* (+ yellow, – blue). The total color difference ΔE = (ΔL*2+ Δa*2+ Δb*2)1/2 was calculated according to the equations using DW as reference.[Citation11]
Sensory evaluation
Sensory evaluation criteria such as meaty; umami; salty; caramel; bitterness; mouthfulness; continuity; and total acceptance were selected based on previous research methods.[Citation12,Citation13] For sensory evaluation, 14 trained panelists (six males and eight females, aged within 23–48 years) were selected from Laboratory of Sensory Science, Hefei University of Technology (Hefei, China) by corroborating the conditions for descriptive analysis.
Volatile compounds analysis
Two microliters of 1,2-dichlorobenzene (50 μg, in 1 mL of methanol) was added to each sample as an internal control which was used to quantify the volatile compounds (1). The volatile compounds were sampled with SPME-fiber (75 μm, carboxen/poly-dimethylsiloxane) and identified through comparison of data obtained from gas chromatography-mass spectrometry (Agilent GC-MS 7890 and DB-5MS 30 m × 0.25 mm × 0.25 μm, Agilent) and NIST 08 (Gaithersburg, MD, USA).[Citation14] The column flow rate was set as 1.0 mL/min, using helium as a carrier gas. The column temperature program was maintained at 40°C for 2 min, 40–100°C at 2°C/min, 100–150°C at 10°C/min, 150–280°C at 20°C/min. The GC was equipped with a mass spectrometric detector which was set at a scanning range of 35 to 450 m/z at a rate of 4.45 scans/s.
where Cv and Ci represent the concentration of volatile compound and internal standard, respectively; Sv and Si corresponded to the peak area of volatile compound and internal standard.
Estimation of MW distribution
MW distribution profiles of the prepared samples were determined by Waters e2695 Alliance HPLC system.[Citation10] The chromatographic conditions were as follows: TSK gel 2000 SWXL 7.8 i.d. × 300 mm (Tosoh Co., Tokyo, Japan) at 30°C. The effluent was monitored at the wavelengths of 220 nm. The mobile phase consisting of acetonitrile/water/trifluoroacetic acid (45/55/0.1, v/v/v) was set at a flow rate of 0.5 mL/min with an injection volume of 10.0 μL.
Total and free amino acid
For determination of free amino acids and total amino acids generated, samples were prepared as per the previous method described by Karangwa et al.[Citation10] The quantification was performed by C-18 Inertsil ODS-SP columns and the Waters e2695 Alliance HPLC system (Waters, Milford, MA, USA).[Citation15]
Statistical analysis
One-way ANOVA through SPSS Statistics 20.0 (SPSS, Inc., Chicago, IL, USA) were used for data analysis at a significance level of P < 0.05. PLSR was performed by using Unscrambler version 9.7 (CAMO ASA, Oslo, Norway) to obtain the potential correlations among sensory characteristics considered in the present study.
Results and discussion
Flaxseed protein hydrolysates composition
From our previous report, it was clear that defatted flaxseed meal consisted of 38.57% protein content, 11.54% crude fiber content, 1.92% fat content, and 3.12% moisture content.[Citation7] The proximate determination of the flaxseed protein hydrolysates revealed the composition such as fat content (0.32%), moisture content (2.66%), protein content (34.81%), crude fiber content (6.40%), total sugar content (40.12%), and ash content (6.10%) which was almost similar to defatted flaxseed meal. However, hydrolysis confirmed the breaking down of high MW proteins of defatted flaxseed meal into peptides with a MW less than 3,000 Da.[Citation7] It was noticed that the Maillard reaction activity of samples was improved after hydrolytic treatment.
Effect on browning of MRPs
The significant effects of cysteine addition, initial pH, and thermal treatment were observed on MRPs derived from above Maillard reaction model. Therefore, cysteine was added at the concentration range of 0, 0.05, 0.10, 0.15, 0.20, and 0.25 g at initial pH 7.5. The initial pH of the model was adjusted to 6.5, 7.0, 7.5, 8.0, and 8.5 after addition of 0.15 g of cysteine at the thermal treatment (100–140°C) for 0–180 min.
Browning intensity of MRPs can be expressed by measuring the observance values at 294 nm and 420 nm. The value at 294 nm represents the uncolored intermediate compounds of the MRPs, which indicated the accumulation of intermediate products.[Citation16] Absorbance at 420 nm represents the MRPs in the final stage during the reaction, such as the brown polymers.[Citation17] With the accumulation of the brown polymers, the MRPs color turns brown. (a) shows that the change in A420 reduced sharply during cysteine addition (0 to 0.10 g) (P < 0.05) and the lowest browning intensity was observed during cysteine addition (0.10 to 0.25 g) at initial pH of 7.5 (P > 0.05). This phenomenon ascribed the inhibition of browning and color formation after the addition of cysteine during Maillard reaction.[Citation18] The initial pH plays an essential role, as many of the Maillard pathways are acid-based catalyzed. The equilibrium between the protonated and unprotonated amino groups is dependent on pH and pKa of the amino acid. At lower pH, a higher proportion of the amino groups is protonated and therefore, it is less nucleophilic, thus, the reactivity leads to lowering of the pH.[Citation19] (b) shows that MRPs with an initial pH of 6.5–7.0 had lower browning (P < 0.05) than MRPs with initial pH of 8.0–8.5. This indicates that browning of the Maillard reaction system is inhibited at lower initial pH. As shown in (c,d), browning intensity (294 nm and 420 nm) of our MRPs improved significantly (P < 0.05) with rising thermal temperature and time and later became relatively stable (P > 0.05). However, the browning intensity did not change significantly (P > 0.05) during the low temperature and short time reaction stages (100°C, 0–60 min). The criterion for color variety was similar to as reported in the previous study.[Citation20]
Effect on color of MRPs
The color difference values of the MRPs were measured to achieve accurate analysis of the apparent color of the samples.[Citation21] The parameters ΔL*, Δa*, Δb*, and ΔE were used to measure the change in MRPs as shown in . The ΔL*, Δa*, Δb*, and ΔE values of MRPs were improved with rising cysteine concentrations (P < 0.05) as shown in (a–d). MRPs (without cysteine) heated at 120°C for 2.0 h at an initial pH of 7.5 displayed the lowest ΔL* and ΔE values which may be the reason for their dark color compared to other MRPs. On the other hand, MRPs with cysteine (0.15 to 0.25 g) heated at 120°C for 2.0 h at an initial pH of 7.5 were the lightest samples compared to other MRPs. The MRPs with an initial pH of 6.5 to 7.5 showed significantly lower ΔL*, Δa*, Δb*, and ΔE values than the MRPs at an initial pH of 8.0 to 8.5 ((e–h)). The values of ΔL* from small to large range (or from negative to positive) indicates that the color of the sample turned from black to white. It was clear from our data that the higher cysteine concentration and increased initial pH did not favor the formation of brown color. The brown components are melanoidins derived from amino-group and xylose.[Citation22] As shown in (i–l), at higher heating temperature and time, all the values (ΔL*, Δa*, Δb*, and ΔE) showed a normal decreasing trend (P < 0.05). Heating temperature and time are the key factors for the initiation of the Maillard reaction. In agreement with previous reports for other materials[Citation23,Citation24], our research has also proved that the color formation in a sample increases with rising temperature.
Sensory evaluation
The sensory properties of MRPs with cysteine addition, initial pH, and thermal treatment were assessed by well-trained team of 14 personnel. The data revealed that the sensory properties of the MRPs were markedly affected by cysteine addition, initial pH, and thermal treatment (). Cysteine addition had a significant (P < 0.05) effect on meaty and caramel taste ((a)). At 0.15 g of cysteine, the Maillard reaction system possessed the maximum taste of meat and caramel. Previous studies have shown that lower cysteine addition leads to less pronounced meaty taste; while, its higher levels increase the sulfur odor.[Citation24] Initial pH had a significant (P < 0.05) effect on meaty taste and total acceptance ((b)). At the initial pH of 8.0, the system showed the highest meaty taste. However, the highest total acceptance was achieved at initial pH of 7.5. The mechanisms and kinetics of Amadori degradation reactions are known to be dependent on the initial pH. The 2,3-enolization is favored over 1,2-enolization reactions under higher pH conditions.[Citation25,Citation26] Generally, 2,3-enolization products are precursors of many important flavor compounds.[Citation27] The Amadori degradation derived from glucose and proline system was reported to increase with pH from 6.0 to 8.0 and correlated to the enhanced formation of selected aroma compounds.[Citation28] As shown in (c, f, g, h, and j), at 100–110°C, the scores for meaty taste, mouthfulness, and continuity were augmented gradually. However, at 120°C and longer treatment time, these sensory values improved rapidly (P < 0.05). In addition, a sharp increase of burned taste was observed after 60 min of heating at 130°C and 140°C (P < 0.05), which also led to the significant (P < 0.05) decrease of meaty taste, umami, mouthfulness, continuity, and total acceptance. Only saltiness was noticed with non-significant (P > 0.05) poor score for thermal treatment among all the sensory characteristics ((e)). However, the caramel and bitterness values of MRPs were increased with rising heating temperature and time (P < 0.05) ((d, i)). Generally, the volatile compounds found in samples were increased with enhanced thermal treatment; while, excessively high heating temperature and time could form undesirable flavor components.[Citation20]
Effect on volatile compound formation of MRPs
Furans: Our results showed that the furans content was increased at lower cysteine concentrations (P < 0.05) and increasing initial pH. As reported previously, furans could be engendered by sugar caramelization.[Citation29] MRPs with 0.05 g of cysteine (1.529 μg/g) and MRPs with the initial pH of 8.5 (1.102 μg/g) were observed with the maximum furans content than other MRPs. Therefore, cysteine addition in the model did not benefit the formation of furans. We found that furans increased rapidly with at lower cysteine concentration and increasing initial pH. Carbonyl-amino condensation reaction producing Amadori compounds is a reversible process. Amadori compounds tend to produce 1,2-enolization forming 3-deoxysone in acid condition; while, under neutral/alkaline conditions, 2,3-enolization is converted into 1-deoxysone.[Citation25,Citation26] 1-Deoxysone is the precursor of many flavor compounds. Dehydration of deoxyketone leads to the development of a huge amount of furan-type compounds. At the lower thermal temperature and time system (110°C, 90 min), low content of furans was observed which increased gradually. A clear rise in furans generation was measured at 90–150 min of heating at 110°C, 120°C, and 130°C. Our study reported that higher caramel was due to high concentrations of furans and furanones in MRPs which was consistent with Liu et al.[Citation20]
Sulfur containing compounds: The sulfur containing compounds are the key constituents produced in the MRPs with cysteine addition providing meaty, roast, and boiled flavors[Citation30]. depicted the increasing content of sulfur-containing volatile components with the increase of cysteine addition, initial pH, heating temperature and heating time. Previous studies on heat treatment and sulfur-containing amino acids obtained similar reaction patterns.[Citation10,Citation20,Citation24] In our study, high levels of thiophene and thiol in volatile constituents of sulfur were noticed. It can be seen from previous data that thiophenes and mercaptans are the important sources of meat.[Citation27,Citation31] Sulfur substituted furans and aliphatic sulfur compounds have low odor threshold values with strong meat-like and/or roast aromas. MRPs without cysteine and MRPs with initial pH of 6.5 and 7.0 did not possess sulfur substituted furans and aliphatic sulfur compounds. However, MRPs with cysteine and MRPs with initial pH of 7.5 to 8.5 generally contained sulfur substituted furans and aliphatic sulfur compounds. 2-Methyl-3-furanthiol and furfuryl mercaptan with low odor threshold values were important sulphur substituted furans for meat-like and/or roast aromas. A previous study reported that furfuryl mercaptan showed strong coffee scents, and 2-Methyl-3-furanthiol presented roast meat or coffee aroma.[Citation31] In case of thiazoles, 1,3-thiazole, 5-ethylthiazole, and 4,5-dimethyl-thiazole were only detected in MRPs with cysteine addition (0.20 and 0.25 g) () besides other six thiophenes. Liu et al.[Citation32] reported that high temperature may lead to increased thiophene concentration. Thiophenes, thiazoles were not detected in samples treated at 110°C for 90–150 min and 120°C for 90 min. However, the contents of thiophenes, thiazoles, thiols, sulfur substituted furans, and aliphatic sulfur compounds were enhanced with the rising thermal temperature and time. Samples at 130°C showed a higher concentration of thiophenes and thiazoles compared with other samples.
Table 1. Effect of cysteine, initial pH and thermal treatment on volatile compounds (µg/g) in MRPs. 110–90 indicated the sample was heated at 110°C for 90 min. Others indicated the corresponding thermal treatment
Nitrogen-containing compounds: As shown in , the pyrazines of MRPs derived from model system decreased with the increasing cysteine concentration (P < 0.05) and increased with the rising initial pH (P < 0.05). Pyrazines constitute an important class of volatile compounds formed during Maillard reaction. Their main flavor characteristics are roasted and nutty flavors. Previous studies showed that the generation of pyrazines, pyridines, and thiazoles in the MRPs of xylose and chicken peptide require a higher temperature.[Citation20] Pyrazines content ascended with the increasing heating temperature and time (). Particularly, samples treated at 130°C for 150 min possessed the maximum pyrazines content (0.103 μg/g). In our study, one pyridine and one pyrimidine were identified with the higher concentration at high temperature. The Strecker degradation was responsible for the formation of pyrazines which pointed out the higher temperature during Strecker degradation.[Citation33]
Oxygen-containing compounds: According to Karangwa et al.[Citation10] most of the oxygen-containing compounds have a minor effect on the sensory properties of MRPs due to their high odor threshold values compared to sulfur-containing compounds and furans. The contents of ketones, alcohols, and esters in MRPs were increased with the rising cysteine concentration (P < 0.05), while the contents of alcohols and esters in MRPs were increased with the growing initial pH values (P < 0.05). Changes in aldehydes content were more complicated with the increase of cysteine addition and initial pH. Concentration of oxygen-containing compounds enhanced with the increasing thermal temperature and time. Therefore, Strecker aldehydes in the aroma-impact compounds were increased markedly with the strong heat treatment conditions.
The above results showed that MRPs with cysteine, MRPs with an initial pH of 7.5 to 8.5 systems, and higher heating temperature and time could enhance the formation of sulfur containing compounds. On the other hand, MRPs without cysteine, MRPs with initial pH of 7.5 to 8.5 systems, and higher heating temperature and time resulted into elevated levels of furans, nitrogen-containing compounds, and oxygen-containing compounds.
Effect on MW distribution of MRPs
As flavor precursors with high reaction activity, the MRPs generated from peptides with 1,000 to 5,000 Da could also significantly affect the umami, mouthfulness, and continuity taste.[Citation12] The MW distribution was significantly affected by cysteine addition, initial pH, and thermal treatment for MRPs (). The increase of cysteine addition and initial pH and the peptide distribution of MRPs showed a similar trend. The MW distribution of >3,000 Da, 1,000–3,000 Da, 500–1,000 Da, 128–500 Da, and <128 Da were used to study the change in the samples. As shown in (a,b), the MW distribution of >3,000 Da, 1,000–3,000 Da, and <128 Da of MRPs reduced with the increasing cysteine addition and initial pH except MRPs without cysteine addition (P < 0.05). On the other hand, the MW distribution of 500–1,000 Da and 128–500 Da of MRPs was increased with the rising cysteine concentration and initial pH (P < 0.05). Our results confirmed that the increase in cysteine concentration and initial pH could accelerate the degradation rate of peptides. Ultimately, the larger peptides were degraded into smaller fractions which were in accordance with previous report.[Citation11] On the other hand, the reducing cross-linking effect of cysteine may elucidate the color-inhibition phenomenon, which efficiently inhibited the formation of the peptides with higher MW (>3,000 Da) such as melanoidins. The peptide distribution of the MRPs treated under different thermal temperature and time can be seen in (c–g). At 110–130°C, the high MW peptide (>3,000 Da and 1,000–3,000 Da) were continuously increased along with the decrease of <1,000 Da peptide, which indicated that the intermediate compounds of the Maillard reaction were predominant with the extension of thermal time. As the temperature was raised, this trend was significantly improved (P < 0.05). Numerous studies have shown that there is thermal degradation of peptides during the initial phase of the Maillard reaction[Citation20,Citation34]; however, this phenomenon was not observed in our study. It might owe to the complete thermal degradation of the peptide before the 90 min of thermal time. Lan et al.[Citation23] reported the cross-linking of peptides at 110°C. To summarize, our data affirmed that 128–500 Da peptides would be the main participants in this cross-linking reaction along with amino acids (<128 Da) with high reaction activity. This information indicated that the peptides with lower molecular weights can be more reactive. This statement did not comply with the previous report which suggested that 1,000–5,000 Da peptide was the most active component in Maillard reaction.[Citation12]
Effect on amino acid content of MRPs
Due to the significant effect of amino acids on the sensory characteristics of MRPs, the amino acid compositions of MRPs are shown in . The amounts of free amino acids, total amino acids, free/total bitter amino acids (Tyr, Ile, Leu, Val, Phe, and Lys), free/total umami amino acids (Glu and Asp), and free/total sulfur-containing amino acids (Cys and Met) were measured.[Citation35] It can be seen from , that the free umami and bitter amino acids increased significantly (P < 0.05) after the addition of cysteine, which may be due to the additional cysteine competition to react with xylose. On the other hand, free amino acids and free sulfur-containing amino acids increased significantly (P < 0.05) due to the cysteine addition. also revealed the non-significant differences among all the samples for total amino acids, total umami amino acids, total bitterness amino acids, and total sulfur-containing amino acids (P > 0.05). However, free amino acids, free umami amino acids, free bitterness amino acids, and free sulfur-containing amino acids were significantly increased (P < 0.05) with the growing initial pH. Blank et al.[Citation28] reported that the increase of pH can improve the rate of Maillard reaction. The mechanisms and kinetics of Amadori degradation reactions are also known to be dependent on pH. For example, 2,3-enolization is favored over 1,2-enolization reactions under higher pH conditions.[Citation26] According to , with the increase of heating temperature and time, a significant (P < 0.05) downward trend was observed in both free amino acids (FA, FU, FB, and FS) and total amino acids (TA, TU, TB, and TS). Maillard reaction involves amino acids or short peptides as reactants to form a large number of rich flavor substances under the reaction of reducing sugar. Liu et al.[Citation20] reported that the temperature of 120°C produced optimum yield of pyrazines in a glucose-ammonia system after 2 h of thermal treatment; while, pyrazine formation was inhibited at a temperature below 70°C. Likewise, Coleman & Perfetti[Citation36] reported that the optimum temperature of pyrazine formation in the glucose-asparagine was 120°C or higher and it can be explained by the higher activation energy required for the generation of pyrazines. Therefore, the Maillard reaction of many kinds of amino acids and short peptides can contribute for the formation of rich taste, and the further the addition of cysteine can emphasize the meat taste.
Table 2. Effect of cysteine, initial pH, and thermal treatment on amino acid content (mg/g) in MRPs
Relationship between browning, color, volatile compounds, MW, amino acid, and sensory characteristics
The correlation between the various factors was established by using ANOVA with PLSR. The browning, color, volatile compounds, MW distribution, and amino acid composition of the MRPs were affected by cysteine addition, initial pH, and thermal treatment. In , the X-matrix represented the browning, color, volatile compounds, MW distribution, and amino acid composition, whereas the Y-matrix presented the MRPs with the increasing cysteine addition, initial pH, thermal treatment, and the sensory evaluation (). The two large circles inside the plot corresponded to 50% and 100% explained variances, respectively. For cysteine addition, the calibrated explained variances for these models were PC1 = 93%, PC2 = 6%, and PC3 = 1%, respectively. For initial pH, variances were PC1 = 74%, PC2 = 23%, and PC3 = 2%, respectively. For thermal treatment, variances were PC1 = 99%, PC2 = 0%, and PC3 = 0%.
Figure 5. An overview of the variation found in the mean data from the PLSR correlation loadings plot for MRPs samples. The model was derived from browning, color, volatile compounds, MW, and amino acids as the X-matrix and sensory characteristics as the Y-matrix for (a) PC1 versus PC2 for cysteine, (b) PC1 versus PC3 for cysteine, (c) PC1 versus PC2 for initial pH, (d) PC1 versus PC3 for initial pH, (e) PC1 versus PC2 for thermal treatment, (f) PC1 versus PC3 for thermal treatment. Ellipses represented r2 = 0.5 and 1, respectively
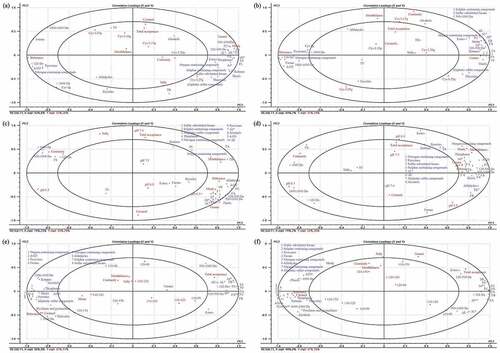
As indicated in (a,b), cysteine additions of 0–0.10 g were located at the left side; whereas, 0.15–0.25 g of cysteine additions were located at the right side. The cysteine addition of 0–0.10 g showed bitterness characteristics which were positively influenced by A420, nitrogen-containing compounds, furans as well as peptides with MW lower than 128 Da, 1,000–3,000 Da, and higher than 3,000 Da. Contrary to that, the cysteine addition of 0.15–0.25 g showed meaty and umami characteristics which were positively influenced by ΔL*, Δa*, Δb*, ΔE, free amino acid (include FA, FU, FB, FS), total amino acid (include TA, and TS), sulfur-containing compounds, oxygen-containing compounds as well as peptides with MW 128–500 Da, and 500–1,000 Da. However, the increase in sulfur-containing amino acids was due to the addition of cysteine to the samples. As shown in (c,d), initial pH 6.5 was located at the left side, whereas initial pH 7.0–8.5 were located at the right side. The initial pH 6.5 showed continuity characteristics which were positively influenced by FS, and peptides with MW lower than 128 Da, 1,000–3,000 Da, and higher than 3,000. However, the sensory characteristics of initial pH 7.0–8.5 were found more complex with the correlation of browning, color, volatile compounds, amino acids, and MW distribution. It can be seen from (c,d) that the meaty, umami, bitterness, and mouthfulness of the sample increased with the initial pH as compared to initial pH 6.5. On the other hand, the initial pH 7.0–8.5 showed these sensory characteristics which were positively influenced by more and more volatile compounds (include furans, and, sulfur, nitrogen, oxygen-containing compounds) and smaller MW distribution (peptides with MW 128–500 Da and 500–1,000 Da). Generally, 2,3-enolization has favored over 1,2-enolization reactions under higher initial pH conditions, whereas 2,3-enolization products further react to produce more flavor components than 1,2-enolization products.[Citation25,Citation26] This trend was found consistent with our findings. Thermal treatments of 120–150, 130–90, 130–120, and 130–150 were located at the left side, whereas thermal treatment of 110–90, 110–120, 110–150, 120–90, and 120–150 were located at the right side in (e,f). The thermal treatment of left side in (e,f) showed meaty, caramel, and bitterness characteristics, while the meaty flavor gradually decreased and the bitterness increased with the rising thermal temperature. Further analysis showed that the thermal treatment of left side in (e,f) have positively influenced the absorbance values (A294 and A420), volatile compounds (include furans, and, sulfur, nitrogen, oxygen-containing compounds), and lower MW distribution (peptides with MW 1,000–3,000 Da and higher than 3,000 Da). On the other hand, the thermal treatment of right side in (e,f) showed umami and total acceptance characteristics which were positively influenced by ΔL*, Δa*, Δb*, ΔE, free amino acid (include FA, FU, FB, FS), total amino acid (include TA, TU, TB, and TS), esters as well as peptides with MW lower than 128, and 128–1,000 Da. Liu et al.[Citation20] reported the changes in the heat-treated flavor of chicken peptides and our findings were consistent with these data. To conclude, cysteine addition, initial pH, and thermal treatment had significantly affected the color and sensory characteristics of MRPs which can be supported by the previous studies.[Citation13,Citation37]
The results of PLSR correlation were found consistent with our chemical analysis data. We assume that cysteine inhibited the browning probably due to inhibition of the formation of high molecular weight protein melanin. Therefore, when cysteine was added at a low level, the content of substances larger than 3,000 Da and melanoid (A420) increased. The effect of thermal treatment on MRPs in PLSR correlation analysis was also consistent with the chemical analysis data. The effect of heat treatment on MRPs in PLSR correlation analysis was also consistent with the result observed in chemical analysis. For example, with the increase of reaction temperature and time, protein melanin (A420) and high molecular weight substance (more than 3,000 Da) were increased.
Conclusion
The cysteine addition, initial pH, and thermal treatment could significantly affect the MRPs derived from xylose, cysteine, and flaxseed protein hydrolysates model system. The higher concentration of cysteine or lower initial pH could significantly inhibit the browning and color of MRPs. MW distribution showed that higher concentration of cysteine or initial pH suppressed the cross-linking of 128–1,000 Da peptides and accelerated the degradation of peptides with MW higher than 1,000 Da. The higher temperature and time (120–150, 130–90, 130–120, and 130–150) could remarkably enhance the flavor formation in the Maillard reaction model. PLSR regression revealed that volatile compounds, MW distribution, and amino acids of MRPs had a significant influence on MRPs sensory characteristics. Lower temperatures and longer heating time (110–90, 110–120, 110–150, and 120–90) were responsible for the generation of umami, mouthfulness, and continuity relatively. Volatile compounds analysis confirmed that the generation of sulfur- and nitrogen-containing compounds can lead to meaty aroma in the Maillard reaction system. Altogether, MRPs derived from 1.0 g of flaxseed protein hydrolysates, 0.3 g of xylose, 0.15 g of cysteine at initial pH of 7.5, and thermal treatment at 120°C for 120 min can impart potential meat flavor with favorable sensory characteristics.
Supplemental Material
Download MS Word (337.5 KB)Supplementary material
Supplemental data for this article can be accessed here.
Additional information
Funding
References
- Keunhee, C.; Hyojeong, H.; Kyungok, S.; Woomin, J.; Kyungsoon, C. Effects of Perilla Oil on Plasma Concentrations of Cardioprotective (N-3) Fatty Acids and Lipid Profiles in Mice. Nutr. Res. Pract. 2013, 7, 256–261. DOI: 10.4162/nrp.2013.7.4.256.
- Bekhit, E. D. A.; Shavandi, A.; Jodjaja, T.; Birch, J.; Teh, S.; Ahmed, I. A. M.; Al-Juhaimi, F. Y.; Saeedi, P.; Bekhit, A. A. Flaxseed: Composition, Detoxification, Utilization, and Opportunities. Biocatal. Agric. Biotechnol. 2018, 13, 129–152. DOI: 10.1016/j.bcab.2017.11.017.
- Peksa, A.; Miedzianka, J. Amino Acid Composition of Enzymatically Hydrolysed Potato Protein Preparations. Czech J. Food Sci. 2014, 32, 265–272. DOI: 10.17221/286/2013-CJFS.
- Arena, S.; Renzone, G.; D’Ambrosio, C.; Salzano, A. M.; Scaloni, A. Dairy Products and the Maillard Reaction: A Promising Future for Extensive Food Characterization by Integrated Proteomics Studies. Food Chem. 2017, 219, 477–489. DOI: 10.1016/j.foodchem.2016.09.165.
- Stadler, R. H.; Blank, I.; Varga, N.; Robert, F.; Hau, J.; Guy, P. A.; Robert, M.; Riediker, S. Food Chemistry: Acrylamide from Maillard Reaction Products. Nature. 2002, 419, 449–450. DOI: 10.1038/419449a.
- Tamanna, N.; Mahmood, N. Food Processing and Maillard Reaction Products: Effect on Human Health and Nutrition. Int. J. Food Sci. 2015, 2015, 526762. DOI: 10.1155/2015/526762.
- Wei, C. K.; Thakur, K.; Liu, D. H.; Zhang, J. G.; Wei, Z. J. Enzymatic Hydrolysis of Flaxseed (Linum Usitatissimum L.) Protein and Sensory Characterization of Maillard Reaction Products. Food Chem. 2018, 263, 186–193. DOI: 10.1016/j.foodchem.2018.04.120.
- AOAC, Association of Official Analytical Chemists. Official Methods of Analysis of the Association Analytical Chemists, 18th ed.; Gaithersburg
- Li, L.; Liao, B. Y.; Thakur, K.; Zhang, J. G.; Wei, Z. J. The Rheological Behavior of Polysaccharides Sequential Extracted from Polygonatum Cyrtonema Hua. Int. J. Biol. Macromol. 2017, 109, 761–771. DOI: 10.1016/j.ijbiomac.2017.11.063.
- Karangwa, E.; Zhang, X.; Murekatete, N.; Masamba, K.; Raymond, L. V.; Shabbar, A.; Zhang, Y.; Duhoranimana, E.; Muhoza, B.; Song, S. Effect of Substrate Type on Sensory Characteristics and Antioxidant Capacity of Sunflower Maillard Reaction Products. Eur. Food Res. Technol. 2015, 240, 939–960. DOI: 10.1007/s00217-014-2398-2.
- Huang, M.; Liu, P.; Song, S.; Zhang, X.; Hayat, K.; Xia, S.; Jia, C.; Gu, F. Contribution of Sulfur-Containing Compounds to the Colour-Inhibiting Effect and Improved Antioxidant Activity of Maillard Reaction Products of Soybean Protein Hydrolysates. J. Sci. Food Agric. 2011, 91, 710–720. DOI: 10.1002/jsfa.4240.
- Ogasawara, M.; Katsumata, T.; Egi, M. Taste Properties of Maillard-Reaction Products Prepared from 1000 to 5000 Da Peptide. Food Chem. 2006, 99, 600–604. DOI: 10.1016/j.foodchem.2005.08.040.
- Ogasawara, M.; Yamada, Y.; Egi, M. Taste Enhancer from the Long-Term Ripening of Miso (Soybean Paste). Food Chem. 2006, 99, 736–741. DOI: 10.1016/j.foodchem.2005.08.051.
- Song, S. Q.; Zhang, X. M.; Hayat, K.; Huang, M. G.; Liu, P.; Karangwa, E.; Gu, F.; Jia, C.; Xia, S.; Xiao, Z.; et al. Contribution of Beef Base to Aroma Characteristics of Beeflike Process Flavour Assessed by Descriptive Sensory Analysis and Gas Chromatography Olfactometry and Partial Least Squares Regression. J. Chromatogr. A. 2010, 1217, 7788–7799. DOI: 10.1016/j.chroma.2010.10.046.
- Checa-Moreno, R.; Manzano, E.; Mirón, G.; Capitán-Vallvey, L. F. Revisitation of the Phenylisothiocyanate-Derivatives Procedure for Amino Acid Determination by HPLC-UV. J. Sep. Sci. 2008, 31, 3817–3828. DOI: 10.1002/jssc.200800363.
- Lertittikul, W.; Benjakul, S.; Tanaka, M. Characteristics and Antioxidative Activity of Maillard Reaction Products from a Porcine Plasma Protein–Glucose Model System as Influenced by pH. Food Chem. 2007, 100, 669–677. DOI: 10.1016/j.foodchem.2005.09.085.
- Kim, J. S.; Lee, Y. S. Characteristics and Antioxidant Activity of Maillard Reaction Products from Fructose-Glycine Oligomer. Food Sci. Biotechnol. 2010, 19, 929–940. DOI: 10.1007/s10068-010-0131-x.
- Matmaroh, K.; Benjakul, S.; Tanaka, M. Effect of Reactant Concentrations on the Maillard Reaction in a Fructose-Glycine Model System and the Inhibition of Black Tiger Shrimp Polyphenoloxidase. Food Chem. 2006, 98, 1–8. DOI: 10.1016/j.foodchem.2005.05.029.
- Lund, M. N.; Ray, C. Control of Maillard Reactions in Foods: Strategies and Chemical Mechanisms. J. Agric Food Chemi. 2017, 65, 4537–4552. DOI: 10.1021/acs.jafc.7b00882.
- Liu, J.; Liu, M.; He, C.; Song, H.; Chen, F. Effect of Thermal Treatment on the Flavor Generation from Maillard Reaction of Xylose and Chicken Peptide. LWT – Food Sci. Technol. 2015, 64, 316–325. DOI: 10.1016/j.lwt.2015.05.061.
- Delgadoandrade, C.; Morales, F. J.; Seiquer, I.; Navarro, M. P. Maillard Reaction Products Profile and Intake from Spanish Typical Dishes. Food Res. Int. 2010, 43, 1304–1311. DOI: 10.1016/j.foodres.2010.03.018.
- Kumar, R.; Rajamanickam, R.; Nadanasabapathi, S. Effect of Maillard Reaction Products (Mrp) on Chlorophyll Stability in Green Peas. Food Nutr. Sci. 2013, 4, 879–883. DOI: 10.4236/fns.2013.49115.
- Lan, X. H.; Liu, P.; Xia, S. Q.; Jia, C. S.; Mukunzi, D.; Zhang, X. M.; Xia, W.; Tian, H.; Xiao, Z. Temperature Effect on the Non-Volatile Compounds of Maillard Reaction Products Derived from Xylose-Soybean Peptide System: Further Insights into Thermal Degradation and Cross-Linking. Food Chem. 2010, 120, 967–972. DOI: 10.1016/j.foodchem.2009.11.033.
- Eric, K.; Raymond, L. V.; Abbas, S.; Song, S.; Zhang, Y.; Masamba, K.; Zhang, X. Temperature and Cysteine Addition Effect on Formation of Sunflower Hydrolysate Maillard Reaction Products and Corresponding Influence on Sensory Characteristics Assessed by Partial Least Square Regression. Food Res. Int. 2014, 57, 242–258. DOI: 10.1016/j.foodres.2014.01.030.
- Cui, H.; Jia, C.; Hayat, K.; Yu, J.; Deng, S.; Karangwa, E.; Duhoranimana, E.; Xia, S.; Zhang, X. Controlled Formation of Flavor Compounds by Preparation and Application of Maillard Reaction Intermediate (Mri) Derived from Xylose and Phenylalanine. RSC Adv. 2017, 7, 45442–45451. DOI: 10.1039/C7RA09355A.
- Rizzi, G. P.;. The Strecker Degradation of Amino Acids: Newer Avenues for Flavor Formation. Food Rev. Int. 2008, 24, 416–435. DOI: 10.1080/87559120802306058.
- Hou, L.; Xie, J.; Zhao, J.; Zhao, M.; Fan, M.; Xiao, Q.; Liang, J.; Chen, F. Roles of Different Initial Maillard Intermediates and Pathways in Meat Flavor Formation for Cysteine-Xylose-Glycine Model Reaction Systems. Food Chem. 2017, 232, 135–144. DOI: 10.1016/j.foodchem.2017.03.133.
- Blank, I.; Devaud, S.; Matthey-Doret, W.; Robert, F. Formation of Odorants in Maillard Model Systems Based on L-Proline as Affected by pH. J. Agric. Food Chem. 2003, 51, 3643–3650. DOI: 10.1021/jf034077t.
- Shibamoto, T.;. Heterocyclic Compounds Found in Cooked Beef. J. Agric Food Chemi. 1980, 28, 237–243. DOI: 10.1021/jf60228a031.
- Stetzer, A. J.; Cadwallader, K.; Singh, T. K.; Mckeith, F. K.; Brewer, M. S. Effect of Enhancement and Ageing on Flavor and Volatile Compounds in Various Beef Muscles. Meat Sci. 2008, 79, 13–19. DOI: 10.1016/j.meatsci.2007.07.025.
- Wang, R.; Yang, C.; Song, H. Key Meat Flavour Compounds Formation Mechanism in a Glutathione-Xylose Maillard Reaction. Food Chem. 2012, 131, 280–285. DOI: 10.1016/j.foodchem.2011.08.079.
- Liu, Q.; Niu, H.; Zhao, J.; Han, J.; Kong, B. Effect of the Reactant Ratio on the Characteristics and Antioxidant Activities of Maillard Reaction Products in a Porcine Plasma Protein Hydrolysate-Galactose Model System. Int. J. Food Prop. 2016, 19, 99–110. DOI: 10.1080/10942912.2015.1017048.
- Van, L. F.; Adams, A.; De Kimpe, N. De, K. N. Impact of the N-Terminal Amino Acid on the Formation of Pyrazines from Peptides in Maillard Model Systems. J. Agric Food Chemi. 2012, 60(18), 4697–4708. DOI: 10.1021/jf301315b.
- Xu, Q.; Liu, J.; Song, H.; Zou, T.; Liu, Y.; Zhang, S. Formation Mechanism of Volatile and Non-Volatile Compounds in Peptide-Xylose Maillard Reaction. Food Res. Int. 2013, 54, 683–690. DOI: 10.1016/j.foodres.2013.07.066.
- Yu, M.; He, S.; Tang, M.; Zhang, Z.; Zhu, Y.; Sun, H. Antioxidant Activity and Sensory Characteristics of Maillard Reaction Products Derived from Different Peptide Fractions of Soybean Meal Hydrolysate. Food Chem. 2018, 243, 249–257. DOI: 10.1016/j.foodchem.2017.09.139.
- Coleman, W.; Perfetti, T. The Roles of Amino Acids and Sugars in the Production of Volatile Materials in Microwave Heated Tobacco Dust Suspensions. Beiträge Zur Tabakforschung. 1997, 17, 75–95. DOI: 10.2478/cttr-2013-0660.
- Kocadağlı, T.; Žilić, S.; Taş, N. G.; Vančetović, J.; Dodig, D.; Gökmen, V. Formation of α-dicarbonyl Compounds in Cookies Made from Wheat, Hull-Less Barley and Colored Corn and Its Relation with Phenolic Compounds, Free Amino Acids and Sugars. Eur. Food Res. Technol. 2016, 242, 51–60. DOI: 10.1007/s00217-015-2517-8.