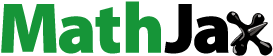
ABSTRACT
The purpose of our study was to evaluate the effect of the addition of Lactobacillus casei and Lactobacillus plantarum to Cheddar cheese on the antioxidant properties during its ripening time and after simulated gastrointestinal conditions. During the whole ripening time, the scavenging of DPPH and hydroxyl radicals both reached their maximum activity at the 16th week, while reducing power activity was reached at the 20th week. The antioxidant values of probiotics cheese were significantly higher than that of control (p< 0.05). Similar results were found after simulated gastrointestinal conditions. The simulated gastric digestion can significantly reduce the activity of DPPH radicals-scavenging (p< 0.05), but after intestinal condition, the values came back to the same level compared with that before digestion. The electrophoretic patterns indicated that adding probiotic to cheese could promote the hydrolysis of protein to enhance the antioxidant activity, especially with mixed probiotics. The addition of probiotic bacteria to Cheddar cheese had nearly no effect on its composition, texture profile analysis, and sensory analysis.
Introduction
Nowadays, people draw more attention to probiotic food for their healthy functions. Probiotics are living microorganisms, which upon ingestion in certain numbers, exert health benefits on the host upon ingestion.[Citation1] Some strains of probiotics have the ability to increase both the safety and the quality of fermented products because they produce various antimicrobial compounds that may prevent the growth of pathogenic and spoilage microorganisms[Citation2,Citation3]. However, probiotics are pretty sensitive to adverse conditions, and their survival rates are relatively poor in food products[Citation4]. Cheese’s compact structure, higher fat content, and lower oxygen content make it a perfect carrier for probiotics application.[Citation5] Numerous researches have indicated the development of probiotic cheeses, including cottage cheese[Citation6], crescenza cheese[Citation7], Cheddar cheese[Citation8], gouda cheese[Citation9], fresh cheese[Citation10], semi-hard cheese[Citation11], and white-brined cheese.[Citation12] Cheddar cheese was selected for this research because of the high popularity of this product[Citation13].
Cheddar cheese is a type of hard cheese characterized by a long ripening period. There is a series of biochemical changes during the cheese ripening period, in which the degradation of protein is the major one. Proteins are degraded by protease and peptidase produced by rennet and starter culture. These peptides not only contribute to the formation of flavor and texture of ripened cheese, but also attain bioactivities in certain time of ripening.[Citation14] A large number of studies have shown that peptides with lower molecular weight are easier to digest than protein and amino acids[Citation15], and possess bioactive properties, including opioid, immunomodulatory, antibacterial, and antioxidant abilities.[Citation16–Citation19] Many studies have identified the proteins within the milk as responsible for its antioxidant activity. Casein can produce antioxidant substances under enzymatic hydrolysis, consequently the antioxidant property of cheese during ripening is related to the degradation of casein. In addition, antioxidative peptides are affected by many factors in the gastrointestinal environment. At present, researchers often use the in vitro simulated digestion technique to study the changes in biological activity[Citation20], but there are nearly no studies on changes of antioxidant peptides in cheese after digestion. So, it is important to study the stability of antioxidant peptides during and after the process of simulated digestion.
This study aimed to evaluate the effect of the addition of probiotics to Cheddar cheese on its antioxidant activity during ripening time and under simulated gastrointestinal conditions. It is important for reasonable evaluation of Cheddar cheese maturity and determination of the best edible period.
Materials and methods
Culture conditions and bacterial strains
Commercial culture (Lactococcus lactis subsp. cremoris and Lactococcus lactis subsp. lactis) was obtained from Chr. Hansen (Chr. Hansen, Bayswater, Victoria, Australia). Probiotic strains of Lactobacillus casei ACCC10171 and Lactobacillus plantarum ACCC10639 were obtained from China Center of Industrial Culture Collection. The Lactobacillus strains were isolated from healthy human intestinal.
Propagation of starter and probiotic organisms
Cheese starter organisms were activated by the method of Liu.[Citation21] The cheese starter organisms were activated by growing at least two times at 30°C overnight in 12% (w/v) sterile reconstituted skim milk (RSM) containing 2% (w/v) glucose and 1.2% (w/v) yeast extract, prior to inoculation (2%, v/v) of the bulk culture in the same medium. The probiotic strains were subcultured (10 μL/mL) two consecutive times at 37°C overnight in 120 mg/mL sterile RSM prior to use as a bulk culture (20 μL/mL).
Cheese manufacture
Cheddar cheeses were manufactured with 15 L of pasteurized milk and 1.5% (v/v) inoculums of the mixed-strain starter culture. The first batch was a control batch containing only starter lactococci (1.5%v/v) (Batch 1), the second batch was made with starter lactococci (1.5%v/v) and Lactobacillus casei (1.2%v/v) (Batch2), the third one with starter lactococci (1.5%v/v) and Lactobacillus plantarum (1.2%v/v) (Batch 3), and the last one with starter lactococci (1.5%v/v), Lactobacillus casei (0.6%v/v), and Lactobacillus plantarum (0.6%v/v) (Batch 4). Cheeses were made on three separate occasions.
Cheese composition
Salt from cheese samples was determined by AOAC 975.20 (1990), fat content by AOAC 905.02 (1990), moisture by GB 5009.3 (2010), and total protein by AOAC 920.123 (1990). A quantity of 10 g of cheese mixed with 6 mL of H2O was used to measure pH using a pH meter (Model 8417, Hanna Instruments Pty. Ltd., Singapore). Cheese composition was analyzed after ripening for 36 weeks at 8°C.
Preparation of water-soluble extracts and Gel electrophoresis
Water-soluble extracts (WSE) of cheese were produced according to the method of Gómez-Ruiz, Ramos, and Recio.[Citation22] The extracts were freeze-dried and kept at −20°C. To evaluate the protein hydrolysis profile of each batch ripened after 36 weeks, SDS-PAGE was carried out according to the method described by Wang.[Citation23]
Antioxidant activity of WSE
Antioxidant activities of cheese WSE were tested by DPPH (2,2- diphenyl-picrylhydrazyl), reducing power and hydroxyl radical-scavenging activity. WSE concentration for reducing power was 15 mg/mL, while 50 mg/mL was used for other analyses. All experiments were performed in triplicate. Cheese samples for analysis were taken at each week until 36.
Scavenging activity of DPPH free radicals
The method of Shimada, Fujikawa, Yahara, and& Nakamura[Citation24] was used to assess the DPPH free radicals-scavenging ability of WSE from Cheddar cheeses. A quantity of 0.1 mL WSE was added to 3.9 mL 60 µM methanolic solution of DPPH, which was freshly prepared. After reacting at room temperature in the dark for 45 min, the absorbance of the resulting solutions was measured at 517 nm with TU-190C ELISA spectrophotometer. The distilled water was used instead of the WSE sample as a control. DPPH free radicals-scavenging activity was calculated by the following formula:
where As is the absorbance value of the sample, and Ac is the absorbance value of the control group. IC50 value was the concentration of the sample used to scavenge 50% of the DPPH free radicals.
Reducing power
The method of Duh, Tu, and Yen[Citation25] was used to assess the reducing power. Samples (15 mg mL−1) dissolved in 2.5 mL phosphate buffer (0.2 mol L−1, pH 6.6) were mixed with 2.5 mL 10 g/L potassium ferricyanide solution, and the mixture was placed in temperature-controlled water bath of 50°C for 20 min. Trichloroacetic acid (2.5 mL of a 10% solution, w/v) was added to the mixture, and then centrifuged at 3000 g for 10 min. The supernatant obtained (2.5 mL) was mixed with 2.5 mL of distilled water after the addition of 0.5 mL of ferric chloride solution (1%, w/v). The absorbance of the reaction mixture was measured at 700 nm. Butylated hydroxy toluene with the same concentration as samples was used as a positive control. OD value represents the ability of reducing power.
Hydroxyl radical-scavenging activity
Scavenging ability of hydroxyl radical was determined with the method mentioned by Liu.[Citation26] A mixture was made out of 1 mL 1,10-phenanthroline (0.75 mM), 1 mL FeSO4 (0.75 mM), 1 mL H2O2 (0.01%, v/v),1.5 mL sodium phosphate buffer (0.15 M, pH 7.4), and 1.0 mL sample solution. The mixture was placed at 37°C for 30 min. The absorbance of the mixture was measured at 536 nm.
where A0 was the absorbance of the deionized water instead of H2O2 and Ac was the absorbance without WSE.
Antioxidant activity after simulated gastrointestinal conditions
The simulated gastrointestinal juice was produced with the method of Mozzi[Citation27] with some modification. The composition of simulated gastric juice consists of KCl (1.12 g/L), NaCl (2.0 g/L), CaCl2 (0.11 g/L), KH2PO4 (0.4 g/L), and pepsin (0.2 g/L), and was adjusted to pH 2.0 with 1 M HCl. The simulated intestinal juice (1.95 g/L) with trypsin was adjusted to pH 7.0 with 1 M NaHCO3. The samples before digestion were prepared as described in “Preparation of WSE.” The samples after digestion were prepared according to the method described by Chaves.[Citation28] A quantity of 10 g cheese was placed in conical flask with 100 mL simulated gastric juice and held for 120 min at 37 °C. The cheese was digested in the simulated intestinal juice for 4 h in the same way. Each digestive juice was centrifuged at 3000 g for 30 min, then filtered through No. 2 Whatman filter paper. Finally, permeate was freeze-dried and kept at −20°C for further use.
Determination of antioxidant activity after simulated digestion
Antioxidant property was tested as the determination of scavenging of DPPH free radicals. The method used was mentioned before.
Instrumental texture profile analysis (TPA)
Texture parameters of Cheddar cheese were determined using the method of Liu.[Citation21] Samples were analyzed after 36 weeks.
Sensory analysis
The sensory evaluation of four batches was performed according to the method of Toba[Citation29] by 12 professional panelists to assess the cheese by flavor (10 points), appearance (10 points), and texture (10 points). The total points are 10. Each batch was analyzed in triplicate.
Design and statistical analysis
Each experiment was performed in triplicate. Statistical analyses were carried out using General Linear Models procedure in Statistix 8.1 software (Analytical Software, St.Paul, MN, USA). One-way analysis of variance was used to study the differences between means, with a significant level at α = 0.05. All data are presented as mean standard error of means.
Results and discussion
Cheese composition
Changes in composition of all samples stored for 36 weeks at 8°C are shown in . The moisture content in cheese of our research (36–39%) was within the typical moisture range for normal Cheddar cheese.[Citation30] The difference of the salt content between cheeses is an indicator of the difficulties in controlling the cheese-making parameters in laboratory scale production. No significant differences were found among each batch of cheese concerning their moisture, fat, protein contents, and pH. The results showed that addition of probiotic bacteria has no influence on the main composition of Cheddar cheese. Similar results were obtained by Ong.[Citation31]
Table 1. Composition of control and probiotic Cheddar cheeses
Gel electrophoresis
The electrophoretic patterns of WSE from each batch are given in . The small molecular stripe of probiotic cheese was markedly lower than that of control cheese, which indicated that the addition of probiotics could promote the degradation of the protein. Similarly, the pattern of Batch 4 was lower than that of Batch 2 or 3. This result may be due to the fact that the addition of a variety of bacteria increased enzyme sites, and further accelerated the hydrolysis of proteins and peptides.[Citation6]
Antioxidant activity of WSE
Scavenging activity of DPPH radical
During the ripening time, scavenging ability of DPPH free radicals increased first, then decreased and finally tended to be gentle, which reached the maximum at 16th weeks (). The values of three probiotic batches were 47.30%, 48.65%, and 51.72%, respectively, which were significantly higher than that in the control (Batch 1) (P < 0.05). And the value of Batch 4 was also significantly higher than that form Batch 2 and Batch 3. At the end of the 36th week, there were significant differences in the control (Batch 1) (35.40%), individual probiotic group (39.40% and 39.42%), and mixed probiotic group (42.19%), respectively (P < 0.05). In each group, the scavenging of DPPH radicals from first 6 weeks showed significant changes (P < 0.05), but not in the last 3 weeks (P> 0.05).
Figure 2. DPPH radical scavenging activity of WSE obtained from Cheddar cheese. Results are expressed as mean ± standard error of means; n = 3 sets of data analysed in duplicate. A-D Means in the same stages of ripening by different samples are significantly different (P<0.05).a-g Means in the same sample by different stages of ripening are significantly different (P < 0.05).Batch 1: control; Batch 2: Lactobacillus casei; Batch 3: Lactobacillus plantarum; Batch 4: Lactobacillus plantarum and Lactobacillus casei
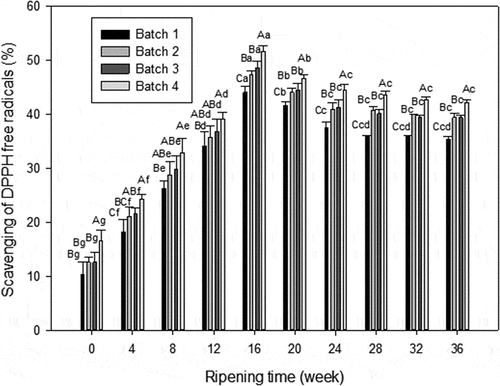
The results indicated that with the extension of ripening time, the degree of protein degradation became higher and higher. In the 16th week, some peptides fractions possibly with antioxidant activity may react with free radicals and transform them to more stable substances, which may cause the fractions to become smaller such as amino acids with no antioxidant activity. The Cheddar cheeses manufactured with the addition of probiotics bacteria showed significantly differences compared with the control cheese (P < 0.05). Moreover, mixed probiotic bacteria showed the best activity of DPPH free radical-scavenging. The reason could be that the probiotic bacteria can promote the degradation of protein in Cheddar cheeses, and the mixed probiotic bacterial may have good assistance with each other, as addressed by previous research.[Citation32] Abadía-García[Citation6] has drawn the similar conclusion that adding probiotics with better proteolytic ability could generate potentially bioactive peptides like antioxidant ones. Meanwhile, the minimum IC50 values () displayed in the 16th week of ripening were 10.76, 9.78, 9.88, and 7.76 mg of protein per mL for each batch, respectively and that of the last week were 14.19, 12.77, 12.92, and 10.37, respectively.
Figure 3. DPPH radical scavenging activity (IC50 value in mg/mL) of WSE obtained from Cheddar cheese. Results are expressed as mean ±standard error of means; n = 3 sets of data analysed in duplicate. Batch 1: control; Batch 2: Lactobacillus casei; Batch 3: Lactobacillus plantarum; Batch 4: Lactobacillus plantarum and Lactobacillus casei
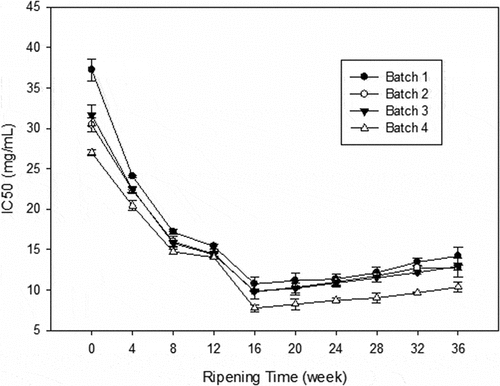
Reducing power
The reducing power of the WSE from all cheese samples are given in . The reduction power of the four kinds of cheese increased first over ripening time, which reached the maximum at 20th week, and decreased in next 2 weeks, then tended to be gentle in last 3 weeks. Both in 20th and 36th week, there were significant difference of reducing power among control, individual probiotic, and mixed probiotic cheese (P < 0.05).
Figure 4. Reducing power of WSE obtained from Cheddar cheese. Results are expressed as mean ± standard error of means; n = 3 sets of data analysed in duplicate. A-D Means in the same stages of ripening by different samples are significantly different (P<0.05).a-g Means in the same sample by different stages of ripening are significantly different (P<0.05). Batch 1: control; Batch 2: Lactobacillus casei; Batch 3: Lactobacillus plantarum; Batch 4: Lactobacillus plantarum and Lactobacillus casei
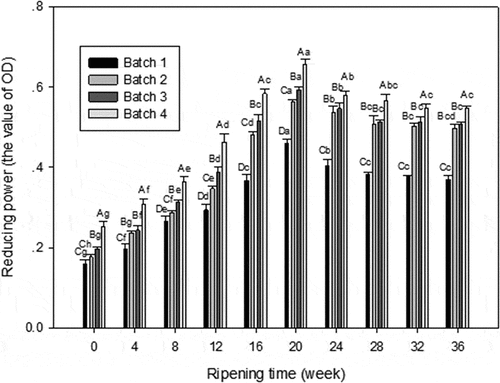
The result showed that with the degradation of protein, the reducing power of WSE increased, it might be explained by the formation of lower molecular substance with metal chelating activity.[Citation16] Meira[Citation33] suggested that the chelating activity decreased as the hydrolysis time increased. Therefore, the reducing power of each batch did not show obvious changes in the last 3 weeks because of the decreased degree of hydrolysis.
Hydroxyl radical-scavenging activity
Hydroxyl radical could go through cell walls and cell membranes and react with the majority of biomolecules easily, and finally inflict on tissue or lead to the death of the cell itself.[Citation34] So, eliminating hydroxyl radicals is necessary for protecting health. The hydroxyl scavenging ability of the WSE from all samples are depicted in . It can be noticed a similar tendency as that of DPPH radical-scavenging. The activity of hydroxyl free radicals-scavenging in cheese increased significantly in the first 4 weeks (P < 0.05), and reached the maximum of 43.86%, 46.19%, 46.66%, and 47.43%. In the 20th and 24th weeks, all of them show significant decrease (P < 0.05). But in the last 3 weeks, no significant differences were observed (P> 0.05). In the 16th week, there were no significant differences (P> 0.05) between mixed and individual probiotic cheese, however, the value of mixed one was significantly higher than that of control cheese (P < 0.05). Similarly, at the end of ripening, the activity of hydroxyl radicals-scavenging from blank cheese was significantly lower than that from other cheeses (P < 0.05).
Figure 5. Hydroxyl radical scavenging activity of WSE obtained from Cheddar. Results are expressed as mean ± standard error of means; n = 3 sets of data analysed in duplicate. A-D Means in the same stages of ripening by different samples are significantly different (P<0.05).a-g Means in the same sample by different stages of ripening are significantly different (P<0.05). Batch 1: control; Batch 2: Lactobacillus casei; Batch 3: Lactobacillus plantarum; Batch 4: Lactobacillus plantarum and Lactobacillus casei
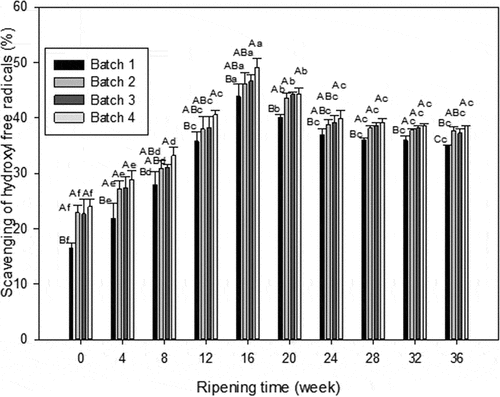
The different behavior of the same WSE with the two methods assessed can be caused by different structure of the radicals that could react in a diverse way depending on the antioxidant substance present in the WSE. But both experiments indicated that adding probiotics can enhance the activity of scavenging free radicals in cheese, especially mixed probiotics. Tong[Citation35] indicated that the antioxidant property of protein was mainly related to their ability to scavenge radicals, therefore, adding probiotic into cheese could enhance the antioxidant ability.
DPPH radicals-scavenging activity after simulated gastrointestinal conditions
indicates that the DPPH free radicals-scavenging of each batch after simulated gastric digestion decreased to 21.24,%, 24.73%, 24.44%, and 27.45, which showed the significant differences (P < 0.05) compared that without digestion (35.40%, 39.40%, 39.42%, and 42.19%). This is consistent with the degradation of β-casein by pepsin studied by Schmelzer.[Citation36] This result may be caused by the degradation of highly bioactive peptides, which prevented their reaction with free radicals.[Citation37] In the process simulated gastric digestion, the DPPH radicals-scavenging activity of cheese with probiotic was significantly higher than that of control (P < 0.05). However, after simulated intestinal digestion, the scavenging of DPPH radicals from all groups (34.37%, 38.47%, 38.61%, and 41.48%) almost returned to the level before digestion without significant difference (P > 0.05). This observation can be explained by the fact that the substance digested by pepsin would break into smaller peptides and amino acid with antioxidant activity due to trypsin and chymotrypsin. Researchers have shown that peptides with low molecular weight exhibited higher antioxidant activity.[Citation38] Similarly, the values of mixed probiotic cheese after simulated intestinal condition weresignificantly higher than that with individual probiotic (P < 0.05), which was also significantly higher than that of control (P < 0.05).
Figure 6. The DPPH radical scavenging activity of WSE before and after simulated gastrointestinal digestion. Results are expressed as mean ± standard error of means; n = 3 sets of data analyzed in duplicate. A-D Means in the same stages of digestion by different samples are significantly different (P < 0.05).a-g Means in the same sample by different stages of digestion are significantly different (P < 0.05). Batch 1: control; Batch 2: Lactobacillus casei; Batch 3: Lactobacillus plantarum; Batch 4: Lactobacillus plantarum and Lactobacillus casei
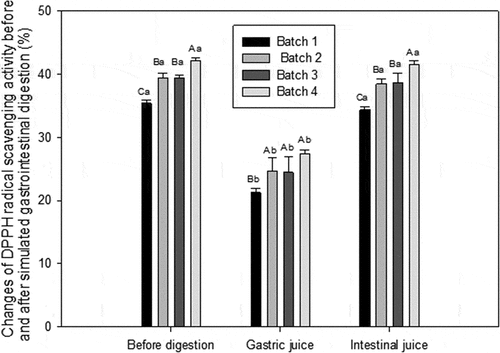
Instrumental texture profile analysis (TPA)
The texture characteristic is related to the composition, structure, and intermolecular stretching force of cheese, which is also an important indicator of changes during cheese ripening. All measured TPA parameters are given in . Compared with the other Cheddar cheese, hardness of control group was significantly higher (P < 0.05). This could be due to the addition of probiotic bacteria, which promoted proteolysis in Cheddar cheeses, producing a large amount of water-soluble substances, and breaking the initial network structure of casein.[Citation39] The Cheddar cheese with two kinds of probiotics was significantly different (P < 0.05) from the blank Cheddar cheese in adhesiveness and springiness, which could have been caused by the addition of probiotics. The cohesiveness of the control was much lower (P < 0.05) than the other batches during ripening. This might be due to less protein degradation, the smaller change of the interactions between proteins, and the remaining of inner bonds in control cheese.
Table 2. Texture profile analysis (TPA) of control and probiotic Cheddar cheeses
Sensory analysis
Sensory scores of all cheeses ripened at 8°C after 36 weeks are given in . No differences were found among the probiotic cheeses, regarding flavour, appearance, and texture, but there was a significant difference (P < 0.05) between control and probiotic cheeses. Probiotic Cheddar cheeses were preferred by the panel, and had higher scores, especially the Cheddar cheese with mixed probiotic bacteria. This could be caused by the higher content of acid in probiotic cheese resulting in more acidic taste and more fragile texture.[Citation40] Considering the positive appreciation by the panel, it can be concluded that Cheddar Cheese is a good carrier for probiotics application, maintaining all desirable sensory characteristics.
Table 3. Sensory scores for control and probiotic Cheddar cheeses
Conclusion
Adding adjunct probiotic cultures (Lactobacillus casei and Lactobacillus plantarum) to Cheddar cheese can increase its antioxidant activity during ripening time and after simulated gastrointestinal digestion for promoting the hydrolysis of protein and peptides, especially mixed probiotic bacteria. The DPPH and hydroxyl radicals-scavenging of all batches both reached their maximum activity at the 16th week, and reducing power at the 20th week. In the last 3 weeks, the antioxidant properties did not show significant differences (P < 0.05). Besides, the addition of probiotics had no significant effect on composition, TPA, and sensory analysis of cheese except hardness and bitterness (P < 0.05).
Additional information
Funding
References
- Guarner, F.; Schaafsma, G. J.; Probiotics. Int. J. Food Microbiol. 1998, 39, 237–238.
- Ross R P; Fitzerald G; Collins K; Stanton C. Cheese Delivering Biocultures–Probiotic Cheese. Aust. J. Dairy Technol. 2002, 57, 71–78.
- Ahmadova A; Todorov S D; Choiset Y; Rabesona H; Zadi T M; Kuliyev A; Franco B D G M; Chobert J M; Haertlé T. Evaluation of Antimicrobial Activity, Probiotic Properties and Safety of Wild Strain Enterococcus Faecium, AQ71 Isolated from Azerbaijani Motal Cheese. Food Control. 2013, 30, 631–641. DOI: 10.1016/j.foodcont.2012.08.009.
- Cook M T; Tzortzis G; Charalampopoulos D; Khutoryanskiy V V. Microencapsulation of Probiotics for Gastrointestinal Delivery. J. Control. Release. 2012, 162, 56–67.
- Karimi, R.; Mortazavian, A. M.; Da Cruz, A. G.; Cruz A. G. D. Viability of Probiotic Microorganisms in Cheese during Production and Storage: A Review. Dairy Sci. Technol. 2011, 91, 283–308. DOI: 10.1007/s13594-011-0005-x.
- Abadía-García, L.; Cardador, A.; Martín Del Campo, S. T.; Arvízu, S. M.; Castaño-Tostado, E.; Regalado-González, C.; García-Almendarez, B.; Amaya-Llano, S. L. Influence of Probiotic Strains Added to Cottage Cheese on Generation of Potentially Antioxidant Peptides, Anti-Listerial Activity, and Survival of Probiotic Microorganisms in Simulated Gastrointestinal Conditions. Int. Dairy J. 2013, 33, 191–197. DOI: 10.1016/j.idairyj.2013.04.005.
- Burns, P.; Patrignani, F.; Serrazanetti, D.; Vinderola, G. C.; Reinheimer, J. A.; Lanciotti, R.; Guerzoni, M. E. Probiotic Crescenza Cheese Containing Lactobacillus Casei and Lactobacillus Acidophilus Manufactured with High-Pressure Homogenized Milk. J. Dairy Sci. 2008, 91, 500–512. DOI: 10.3168/jds.2007-0516.
- Phillips, M.; Kailasapathy, K.; Tran, L. Viability of Commercial Probiotic Cultures (L. Acidophilus, Bifidobacterium Sp. L. Casei, L. Paracasei and L. Rhamnosus) in Cheddar Cheese. Int. J. Food Microbiol. 2006, 108, 276–280. DOI: 10.1016/j.ijfoodmicro.2005.12.009.
- Gomes A. M.; Malcata F X; Klaver F A; Grande H. Incorporation and Survival of Bifidobacterium Sp. Strain Bo and Lactobacillus Acidophilus Strain Ki in a Cheese Product. Ned. Melk En Zuiveltijdschrift. 1995, 49, 71–95.
- Buriti F. C. A.; Rocha J. S. D.; Assis E. G; Saad S M I. Probiotic Potential of Minas Fresh Cheese Prepared with the Addition of Lactobacillus Paracasei. LWT - Food Sci. Technol. 2005, 38, 173–180.
- Bergamini, C. V.; Hynes, E. R.; Zalazar, C. A. Influence of Probiotic Bacteria on the Proteolysis Profile of a Semi-Hard Cheese. Int. Dairy J. 2006, 16, 856–866.
- Özer B; Kirmaci H A; Şenel E; Atamer M; Hayaloğlu A. Improving the Viability of Bifidobacterium Bifidum, BB-12 and Lactobacillus Acidophilus, LA-5 in White-Brined Cheese by Microencapsulation. Int. Dairy J. 2009, 19, 22–29. DOI: 10.1016/j.idairyj.2008.07.001.
- Donaghy, J. A.; Totton, N. L.; Rowe, M. T. Persistence of Mycobacterium Paratuberculosis during Manufacture and Ripening of Cheddar Cheese. Appl. Environ. Microbiol. 2004, 70, 4899–4905.
- Saito T, Nakamura T, Kitazawa H; Kawai Y; Itoh T. Isolation and Structural Analysis of Antihypertensive Peptides that Exist Naturally in Gouda Cheese. J. Dairy Sci. 2000, 83, 1434. DOI: 10.3168/jds.S0022-0302(00)75013-2.
- Otte, J.; Lenhard, T.; Flambard, B.; Sørensen, K. I. Influence of Fermentation Temperature and Autolysis on ACE-inhibitory Activity and Peptide Profiles of Milk Fermented by Selected Strains of Lactobacillus Helveticus and Lactococcus Lactis. Int. Dairy J. 2011, 21, 229–238. DOI: 10.1016/j.idairyj.2010.12.008.
- Corrêa A. P.; Daroit D. J.; Coelho J; Meira S M; Lopes F C; Segalin J; Risso P H; Brandelli A. Antioxidant, Antihypertensive and Antimicrobial Properties of Ovine Milk Caseinate Hydrolyzed with a Microbial Protease. J. Sci. Food Agric. 2011, 91, 2247.
- Korhonen, H.; Pihlanto, A. Bioactive Peptides from Food Proteins. Handbook of Food Products Manufacturing. John Wiley & Sons, Inc. 2001.
- Park, Y. W. Bioactive Components in Goat Milk. Bioact. Compon. Milk Dairy Prod. Wiley‐Blackwell.2009.
- Silva, S. V.; Malcata, F. X. Caseins as Source of Bioactive Peptides. Int. Dairy J. 2005, 15, 1–15.
- Picariello, G.; Ferranti, P.; Fierro, O.; Mamone, G.; Caira, S.; Di Luccia, A.; Monica, S.; Addeo, F. Peptides Surviving the Simulated Gastrointestinal Digestion of Milk Proteins: Biological and Toxicological Implications. J. Chromatogr. B. 2010, 878, 295–308. DOI: 10.1016/j.jchromb.2009.11.033.
- Liu, L.; Li, X.; Bi, W.; Zhang, L.; Ma, L.; Ren, H.; Li, M. Isomaltooligosaccharide Increases the Lactobacillus Rhamnosus Viable Count in Cheddar Cheese. Int. J. Dairy Technol. 2015, 68, 389–398. DOI: 10.1111/idt.2015.68.issue-3.
- Gomezruiz, J. A.; Ramos, M.; Recio, I. Angiotensin-Converting Enzyme-Inhibitory Peptides in Manchego Cheeses Manufactured with Different Starter Cultures. Int. Dairy J. 2002, 12, 697–706.
- Wang, X.; Hou, J. M.; Cong, X. L. Composition Analysis of the Whey Fermentation Product with the Antioxidant Activity. J. Jilin Univ. 2012, 42, 444-448.
- Shimada, K.; Fujikawa, K.; Yahara, K.; Nakamura, T. Antioxidant Properties of Xanthan on the Autoxidation of Soybean Oil in Cyclodextrin Emulsion. J. Agric. Food Chem. 1992, 40, 945–948. DOI: 10.1021/jf00018a005.
- Duh, P. D.; Tu, Y. Y.; Yen, G. C. Antioxidant Activity of Water Extract of Harng Jyur (Chrysanthemum Morifolium Ramat). LWT - Food Sci. Technol. 1999, 32, 269–277.
- Liu, J.; Luo, J.; Ye, H.; Sun, Y.; Lu, Z.; Zeng, X. Production, Characterization and Antioxidant Activities in Vitro, of Exopolysaccharides from Endophytic Bacterium Paenibacillus Polymyxa, EJS-3. Carbohydr. Polym. 2009, 78, 275–281. DOI: 10.1016/j.carbpol.2009.03.046.
- Mozzi F; Gerbino E; Valdez G F D; Torino M I. Functionality of Exopolysaccharides Produced by Lactic Acid Bacteria in an in Vitro Gastric System. J. Appl. Microbiol. 2009, 107, 56–64. DOI: 10.1111/j.1365-2672.2009.04182.x.
- Chaves, K. S.; Gigante, M. L. Prato Cheese as Suitable Carrier for Lactobacillus Acidophilus La5 and Bifidobacterium Bb12. Int. Dairy J. 2016, 52, 10-18.
- Toba, T.; Uemura, H.; Mukai, T.; Fujii, T.; Itoh, T.; Adachi, S. A New Fermented Milk Using Capsular Polysaccharide-Producing Lactobacillus Kefiranofaciens Isolated from Kefir Grains. J. Dairy Res. 1991, 58, 497–502. DOI: 10.1017/S0022029900030107.
- Fox P F . Cheese and Fermented Milk Foods. Int Dairy J, 1997, 7, 360-361.
- Ong, L.; Henriksson, A.; Shah, N. P. Development of Probiotic Cheddar Cheese Containing Lactobacillus Acidophilus, Lb. Casei, Lb. Paracasei, and Bifidobacterium, Spp. And the Influence of These Bacteria on Proteolytic Patterns and Production of Organic Acid. Int. Dairy J. 2006, 16, 446–456. DOI: 10.1016/j.idairyj.2005.05.008.
- Gupta, A.; Mann, B.; Kumar, R.; Sangwan, R. B. Antioxidant Activity of Cheddar Cheeses at Different Stages of Ripening. Int. J. Dairy Technol. 2009, 62, 339–347. DOI: 10.1111/idt.2009.62.issue-3.
- Smm M; Daroit D. J.; Helfer V. E.; Corrêa A P F; Segalin J; Carro, S; Brandelli A. Bioactive Peptides in Water-Soluble Extracts of Ovine Cheeses from Southern Brazil and Uruguay. Food Res. Int. 2012, 48, 322–329.
- Yuan J F; Zhang Z Q; Fan Z C; Yang J X. Antioxidant Effects and Cytotoxicity of Three Purified Polysaccharides from Ligusticum Chuanxiong, Hort. Nat. Prod. Res. Dev. 2008, 74, 822–827.
- Tong L. M.; Sasaki S.; Mcclements D. J; Decker E A. Antioxidant Activity of Whey in a Salmon Oil Emulsion. J. Food Sci. 2010, 65, 1325–1329.
- Schmelzer C. E. H.; Schöps R; Reynell L; Ulbrich-Hofmann R; Neubert R H H; Raith K. Peptic Digestion of β-casein: Time Course and Fate of Possible Bioactive Peptides. J. Chromatogr. A. 2007, 1166, 108–115.
- Chen, M.; Jing, A. O.; Bo, L. I. Effect of Molecular Weight on the Antioxidant Activity of Casein Peptide. Sci. Technol. Food Ind. 2012, 33, 95–99.
- Chen, D.; Guangqing, M. U. Effects of Casein Peptides with Different Molecular Weight on Free Radical Scavenging; China Brewing, 2010, 4, 33–35.
- Gunasekaran, S.; Ak, M. M. Cheese Rheology and Texture. Rev. Bras. Cienc. Farm. 2003, 40, 269.
- Fca, B.; Da, R. J.; Smi, S. Incorporation of Lactobacillus Acidophilus in Minas Fresh Cheese and Its Implications for Textural and Sensorial Properties during Storage. Int. Dairy J. 2005, 15, 1279–1288.