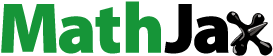
ABSTRACT
In this study, the influence of Na content of dry-cured beef by partial replacement with KCl, L-lysine, and L-histidine was investigated during processing. Dry-cured beef aged over 57 days, compared to control groups (100% NaCl), salt substituted groups (SS, 39.7% of NaCl, 51.3% of KCl and a blend of 7% L-lysine and 2% L-histidine); had a significant impact on the proteolysis index and total free amino acids, lower activities, and gene levels of cathepsin B and L, as well as reduced contents of protein carbonyls and thiol group. The present study provides a successful strategy for improvement of the perceived saltiness and inhibition of the bitterness, while exhibiting no significant negative effects on color or textural properties.
Introduction
Traditional dry-cured beef is a high-quality product with a characteristic texture, produced from raw beef by rubbing with salt followed by air drying.[Citation1] Protein is one of the important macromolecules in dry-cured beef. Changes in the structure of protein affect sensory and nutritional qualities of dry-cured beef. The important ones of factors such as salt type and content, affect proteolysis and protein oxidation which are important biochemical changes during processing, leading to the development of the desired texture and color in the dry-cured meat products.[Citation2] Proteolysis has been attributed to endogenous enzymes. The proteolysis index (PI) and the activity of cathepsin B and L refer to the intensity of proteolysis of dry-cured meat products. Carbonyl content and thiol groups mention the degree of protein oxidation. Interest in low-sodium dry-cured meat products has been growing for decades, given the risk of cardiovascular diseases being associated with excessive sodium (Na) intake.[Citation3] However, reducing sodium chloride (NaCl) content has become a major challenge for the current meat industry as it affects meat texture, color properties, and also the perceived acceptance of these meat products by consumers.
Substitution of the primary salt – sodium chloride used, with inorganic salts such as potassium chloride (KCl), magnesium chloride (MgCl2) or calcium chloride (CaCl2), is an effective way to reduce sodium content.[Citation2] In dry-cured ham (Semimembranosus muscle), Aliño et al.[Citation4] reported that, compared with a 100% NaCl control set, a hybrid compound solution of 50% NaCl/50% KCl did not promote significant (P < 0.05) differences in either color or textural parameters. Armenteros et al.[Citation5] observed that, compared to the 100% NaCl treated meat, dry-cured lacón with a hybrid compound solution of 50% NaCl/50% KCl did not produce a significant change in total free amino acid (FAA) and cathepsins B and B + L activities at the end of processing (270 days); which indicates that NaCl and KCl has a similar effect on the quality and sensory properties of the meat. However, mere substitution with these mono and di-valent salts can lead to perceived bitter or sour tastes in the meat products.[Citation6] Recently, more concern and attention is given to the identification of salt-substitutes including amino acids such as L-lysine and L-histidine.[Citation7] It has been reported earlier that supplementation with organic salts could serve to not only mitigate the health problems associated with Na, but also overcome the bitter taste or sour flavor often attributed to substitutions by KCl and MgCl2.[Citation8] Moreover, L-lysine and/or L-histidine cause conformational transitions in porcine myosin and increase protein solubility.[Citation9] Campagnol et al.[Citation10] has reported that a replacement of 50% NaCl with KCl and other amino acids could reduce the sensory defects in fermented cooked sausages. The addition of L-lysine in pork sausage significantly improved the textural and color properties compared to a control sample group (100% NaCl).[Citation11] Therefore, the use of L-lysine and L-histidine in meat products appears to be another possible and effective substitute for Na, but to the best of the authors knowledge, there is not a single study employed to explore the impact of these alternatives and substitutes on the perceived sensory properties of dry-cured beef.[Citation6]
The focus of this study will be based on the impact of these Na alternatives – salt substitutes containing L-lysine and L-histidine, during the dry-curing process, and all the changes produced during the processing; and their effects on the quality parameters such as proteolysis (proteolysis index, cathepsin B/L activities and free amino acid), protein oxidation (carbonyl content and thiol group levels) the influence on color (lightness, redness and yellowness) and textural properties (shear force and texture profile analysis) of the resulting produce.
Materials and methods
Processing of dry-cured beef semitendinosus
Thirty-six, fresh beef semitendinosus (ST) from Limousin × Luxi steers, with a mean muscle weight of 2.29 ± 0.61 kg and pH values of 5.37 ± 0.12, were obtained from 18 carcasses slaughtered at a Shandong slaughterhouse (Binzhou, Shandong, China). The 18 steers of age 30 (months) were slaughtered and chilled for 48 h at 0–4°C before further processing. Then, the left and right ST muscles of each carcass were separated, labeled, vacuum-packed and immediately frozen at – 40°C until it was required for further use.
Frozen ST muscles were thawed in a refrigerating chamber at 4°C for 5 days. Random carcass parts were chosen for control and treated (salt substitution SS) series of experiments. The ST muscles were rubbed with either salt or SS which comprised of a compound mixture of 39.7% NaCl, 51.3% KCl and a blend of 7% L-lysine and 2% L-histidine. The amount of salt or SS added was 4% of the ST muscle weight. Prior to being stuffed into casings, the raw beef (day 0) was measured for PI, the activities and gene of cathepsin B and L, free amino acid, content of carbonyl and thiol groups, color, shear force, and texture. All ST muscles with salt were stuffed into artificial casings and treated at 90% relative humidity (RH) and 4 ± 1°C for 5 days in order to allow the salt to penetrate. After the salting, the ST muscles were placed in a chamber for ripening at 12°C and 80% RH for 5 days. Subsequently, the ST muscles were dried for ripening at 20°C and 70% RH for 17 days until reaching a 45% weight loss and then kept at 22.5°C for 30 days. Dry-cured beef was evaluated at six processing time points (0, 10, 15, 20, 27, and 57 days). Each ST muscle was chopped into cubes (6 cm × 10 cm × 10 cm), and samples were vacuum-packaged and stored at – 40°C until required for the assay. At each selected time, three cubes were analyzed.
Proteolysis index analysis
Total nitrogen content (TN) was determined according to the Kjeldahl method using a Kjeltec 2300 Analyzer (Foss Tecator AB, Sweden).[Citation12] The proteolysis index (PI) was evaluated for the proteolytic intensity, described by the methods developed by Pérez-Santaescolástica et al.[Citation13] The content of N-terminal α amino groups was expressed as grams of “glycine equivalent” per gram of sample (A). The total protein content was expressed as grams of protein per gram of sample (B). The PI was expressed as the following:
Cathepsin B and L activities
The extracts containing cathepsin B (CTS B, EC 3.4.22.1) and L (CTS L, EC 3.4.22.15) were obtained from 2.5 g samples using the methods described by Armenteros et al.[Citation5] The activity of an enzyme is measured by fluorometric assays using aminoacyl-7-amido-4-methyl coumarin (aa-AMC) (Sigma-Aldrich Co., St. Louis, MO) as fluorescent substrates. Activity levels of cathepsin B (EC 3.4.22.1) and L (EC 3.4.22.15) are determined at pH 6.0 using 0.05 mM N-CBZ-Arg-Arg-AMC and 0.05 mM N-CBZ-Phe-Arg-AMC, respectively.
Gene expression assay
Total RNA from dry-cured beef was extracted using a TRIzol® Plus RNA Purification Kit (Invitrogen, Waltham, MA, USA; 12183–555) and RNase-Free DNase Set (Qiagen, German; 79254) according to the manufacturer’s instructions. The synthesis of the first-strand cDNA was evaluated. PCR assays were conducted using the SuperScript™ III First-Strand Synthesis SuperMix for quantitative real-time PCR (RT-qPCR) for target genes. The primers used in the study are shown in . RT-qPCR assays were conducted by the Power SYBR® Green PCR Master Mix (Applied Biosystems, Foster City, CA, USA). The process parameters for RT-qPCR was set at 95°C for 60 s in the first cycle, followed by 40 cycles at 95°C for 15 s, and a final run at 63°C for 25 s.
Table 1. The primers used for RT-qPCR
Free amino acid analysis
Samples for FAA analysis were estimated according to the methods described by Aro et al.[Citation14] Samples (10 g) were homogenized for 1 min with 90 mL of distilled water. The homogenate was centrifuged for 20 min at 10,000 g. The supernatant was then filtered through a filter and the samples were diluted 1:1 with 4% trichloroacetic acid (TCA) to give a final concentration of 2%. They were subsequently incubated at 37°C for 30 min using a heated electric bath (Shanghai Jing Hong Laboratory Instrument Co., Ltd., China). The supernatant was then filtered again. The solution was ultrafiltered through a Millipore filter (Tianjin Branch Billion Lung Experimental Equipment Co., Ltd., China) with a pore diameter of 0.45 μm. Twenty microlitres of each sample were analyzed using a fully automated amino acid analyzer HITACHI L-8900A (Hitachi Ltd., Japan).
Carbonyl content
Carbonyl contents were determined using the procedure of Armenteros et al.[Citation15] Protein concentration was determined from their absorption at 280 nm through a Beckman Du 730 UV/VIS Spectrophotometer (Beckman Coulter, Inc., US) using BSA as standard. The content of carbonyls was expressed as nmol of carbonyl per mg of protein.
Thiol groups
The thiol groups levels were determined according to the method of Cui et al.[Citation16] The thiol groups level (μmol/g proteins) = 73.53 A412 × D/C. Where 73.53 was equal to 106/1.36 × 104; 1.36 × 104 is the molar absorption coefficient; D is the dilution coefficient (6.04); A412 is the absorbance at 412 nm, and C (mg/mL) was the protein concentration in the tested sample.
Color analysis
The color of the samples was determined according to the methods described by Cui et al.[Citation17] The samples were measured on a freshly cut upper surface of dry-cured beef using a CR-400 colorimeter (Minolta, Osaka, Japan). Before each series of measurements, the instrument was calibrated using a white ceramic tile. Color of the surface was acquired and measured three times for each sample to arrive at the final averaged value. The values are expressed and represented as lightness (L*), redness (a*) and yellowness (b*).
Shear force and instrumental texture profile analysis
The shear force of the samples was determined according to the method of Cui et al.[Citation17] Three samples were taken parallel to the direction of the muscle fiber, avoiding visible fat and external connective tissue. The shear force measurement was carried out with a digital meat tenderness meter (Model C-LM3B, Northeast Agricultural University, Harbin, China). Cylindrical samples (ϕ30 × 10 mm) were cored to shear a fiber direction perpendicular to the cylinder axis.
Texture profile analysis (TPA) was carried out by compression to 60% with a compression probe of 19.85 cm2 surface contact. Force-time curves were recorded at a crosshead speed of 3.33 mm/s. Hardness (kg), springiness (mm), chewiness (kg × mm) and cohesiveness were recorded. These parameters were obtained using the Texture software (Texture Exponent 32, Stable Micro Systems, Vienna Court, UK).
Statistical analysis
Analyses were performed in triplicate, and data were expressed as means followed by the standard deviation. The data obtained on proteolysis index, cathepsin B and L activities, FAA, protein carbonyls and thiol groups, color, and textural parameters were analyzed statistically using the ANOVA from the Statistics 8.1 software package (Analytical Software, St Paul, MN, USA). Data were tested for Linear Models, and differences (P < 0.05) between the control and SS were compared using the Tukey test.
Results and discussion
Proteolysis index (PI)
As shown in , compared with the control, PI value in SS group exhibited significant decrements from 18.99% to 12.96% during 10 and 20 day periods, respectively. However, a slightly increase (10.72%) in the PI value was noted when storage was prolonged to 57 days. Incidentally, there were no significant differences (P > 0.05) between SS and the control in PI at days 0 and 15.
Figure 1. Changes in proteolysis index (PI) in beef during dry-curing
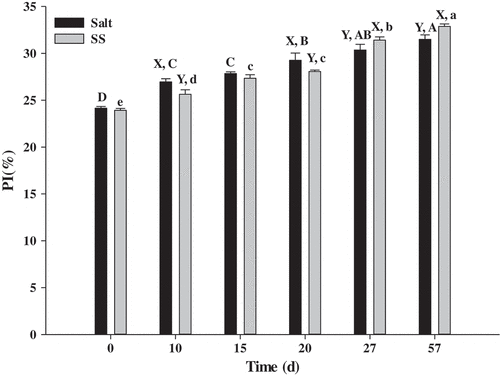
Proteolysis and protein oxidation have important influences on the quality characteristics of dry-cured meat products, being a crucial source that encapsulates characteristics of taste and textural properties.[Citation18] PI refers to the intensity of proteolysis during the processing of dry-cured meat products.[Citation13] PI is influenced by many factors such as the amount of added NaCl and endogenous enzymatic activity.[Citation19] The higher (P < 0.05) PI in SS (days 27 and 57, ) supported by the work of Guo et al.[Citation9] and Li et al.[Citation20], found that L-lysine and/or L-histidine increased the myosin solubility. This results from the presence of L-lysine and/or L-histidine changing myosin secondary structure, that exposes the buried hydrophobic and sulphydryl groups to the surface of myosin, and inhibits myosin aggregation in the process.[Citation20]
Activity and gene expression of cathepsin B and L
Activity and gene expression of cathepsin B and L were also assessed and compared with the control in . Cathepsin B + L activity in SS decreased from 11.76% to 14.15% and then settled at a value of 18.03% during period intervals of 10, 27 and 57 days. While cathepsin B activity in SS decreased from 7.53% to 10.70%, before increasing to 16.21%, and then falling again to 8.24% during period intervals of 10, 15, 20 and 27 days.
Figure 2. Changes in relative activities (a, c) and expression (b, d) of cathepsins B and L in beef during dry-curing
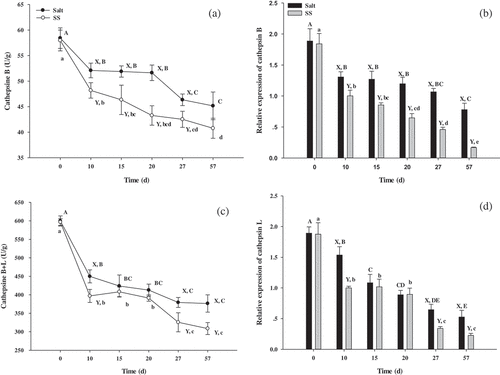
As compared to the control, gene expression of cathepsin B in SS increased from 23.29% to 32.74%, to 45.94%, to 56.94% and then to 78.55% during time intervals of 10, 15, 20, 27 and 57 days (). In addition, gene expression of cathepsin L in SS increased from 34.93% to 47.19% and then 56.93% during 10, 27 and 57 day periods ().
The lower (P < 0.05) PI values in SS (days 10 and 20, ) can be attributed to the lower (P < 0.05) activity of cathepsin B and L (,) in dry-cured beef at days 10 and 20. This is because the intense proteolysis may be mainly attributed to muscle proteinases, since protein degradation shows a strong correlation to the activity of proteolytic enzymes.[Citation19] Cathepsin B and L, the lysosome cysteinyl proteinases, show optimal pH values between 4.0 and 5.5.[Citation21] The lower (P < 0.05) activity of cathepsin B and L in SS (,) is likely due to the down-regulation of gene expression of cathepsin B and L (,) and introduction of L-lysine and L-histidine, which are both basic amino acids and modulates the pH of dry-cured beef.[Citation20] Armenteros et al.[Citation22] found that KCl had a similar effect to NaCl for cathepsin B and L. This suggests that the introduction of L-lysine and L-histidine inhibits the activity of cathepsin B and L which would affect the FAA release. Thus, the introduction of L-lysine and L-histidine results in an increase of the PI values, while inhibiting the activity of cathepsin B and L.
FAA composition comparisons
Changes of FAA content in dry-cured beef for SS and the control are shown in . The total FAA in SS was lower (P < 0.05) than that in the control at 10 and 27 days, while it was higher (P < 0.05) at 15, 20 and 57 days (). Aspartic acid (Asp), cysteine (Cys), glycine (Gly), threonine (Thr), tyrosine (Tyr), serine (Ser), and phenylalanine (Phe) in SS was lower (P < 0.05) than that in the control at 57 days, while alanine (Ala), glutamic acid (Glu), histidine (His), lysine (Lys), and proline (Pro) was found to be higher (P < 0.05).
Table 2. Changes in free amino acids during aging of dry-cured beef with salt or SS treatments (0.01 g × kg−1)
The total amount of FAA significantly increased (P < 0.05) in both SS and the control during the processing time. The highest contents of FAA in the SS and the control were Ala, followed by Glu and Pro at day 0. Lys and Ala in SS and the control were considered to be the FAA present in the highest contents at 57 days, while Cys and Asp were found to be the lowest.
Proteolysis results in the formation of low molecular weight compounds such as peptides and FAAs. When the generation of FAAs is high and exceeds the taste threshold, the taste of the meat products is influenced.[Citation23] The effect of the salting treatment (KCl/NaCl) was no significant on the release of total free amino acids, while the concentrations of some amino acids were significantly different in dry-cured loins and cheese.[Citation24,Citation25] In our study, we find there are significant on the release of total free amino acids and the concentrations of some amino acids. Firstly, the content of Glu in the control and SS was 0.38% and 4.16% (wet basis) in dry-cured beef (), respectively, which was 12.7- and 139 times the taste threshold (0.03%, wet basis). Secondly, the FAA acted as a buffer to maintain the pH near pH 6.0 in the dry-cured beef. At this pH, glutamic acid was present in the form of monosodium glutamate, which mainly contributes to the umami flavor. Thirdly, Kęska and Stadnik[Citation26] found that Lys and Glu in SS imparted a salty and umami flavor, and suppressed the bitter taste. Moreover, the content of FAAs (Leu, Val, Met and Ile) affected the bitterness which was derived from hydrophobic side-chains and α-amino groups.[Citation27] These FAAs had a very low taste threshold in the dry-cured meat products. Therefore, SS dry-cured beef would increase the salty taste and reduce perceived bitterness accordingly.
Protein oxidation comparisons
Compared with the control, SS was lower (P < 0.05) in the protein carbonyls and thiol groups at day 57 (). Protein carbonyl values in SS increased from 29.65% to 35.36% during 27 and 57 days. Values of protein thiol groups in SS increased from 7.50% to 12.70% from day 20 and 57. There were no observable differences (P > 0.05) between SS and the control of other days.
Figure 3. Changes in the content of carbonyl and thiol groups in beef during dry-curing
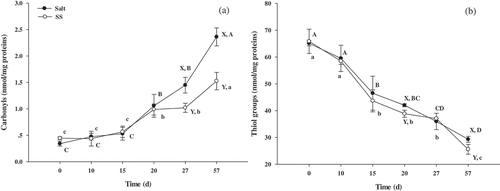
Protein oxidation favors proteolysis, since increased protein hydrophobicity promotes the degradation by proteases, and carbonylated proteins are more susceptible to proteasome-driven proteolysis.[Citation28] Oxidized proteins destroy amino acid and decrease protein solubility due to protein polymerization and loss of enzyme activity, which further has an influence on the texture and color of dry-cured meat products.[Citation29] On one hand, protein oxidation results in the formation of carbonyl compounds which are indicators for evaluating the extent of protein oxidation.[Citation30] The lower (P < 0.05) carbonyl values in SS indicate that the introduction of L-lysine and L-histidine inhibited protein oxidation at days 27 and 57 (). L-Lysine and L-histidine had a strong chelating ability for iron ions which was thought to be based on the tridentate chelates.[Citation31] Moreover, the introduction of L-lysine and/or L-histidine increased the pH value which promoted the stability of metmyoglobin, which then reduced the protein oxidation rate. On the other hand, the thiol content is another important parameter for the evaluation of protein oxidation, and the content of the thiol group is decreased by the oxidation of free thiol group from cysteine residues.[Citation32] The introduction of L-lysine and/or L-histidine increased the loss of thiol groups and accelerated protein oxidation. This result supports the recent work of Xu et al.[Citation30], who found that Lys-treated sausages had lower (P < 0.05) thiol levels than that in the control, and had lower (P < 0.05) carbonyl values. This was because the α, β-unsaturated compounds reacted with thiols in the presence of positively charged Lys, ultimately contributing to the decrease in thiol levels.[Citation30]
Colour and textural parameters
Significant (P < 0.05) decreases of lightness (L*), redness (a*) and yellowness (b*) in both SS and the control were observed from day 0 to day 57 (). SS stimulated a decrease in the L*-values and a*-values at day 10, which was 1.15 and 1.19 times lower (P < 0.05) than that of the control. No pronounced difference (P > 0.05) was obtained for L*-values or a*-values between SS and the control from day 15 to day 57. SS-induced an increment in the b*-values at day 20, which was 1.14 times higher (P < 0.05) than that of the control. There were no significant differences (P > 0.05) in b*-values between SS and the control of other days.
Table 3. Changes in textural and color parameters during aging of dry-cured beef using salt and SS treatments
Shear force in SS was lower (P < 0.05) than that in the control of day 15, and higher at day 20 (). The hardness of SS was higher (P < 0.05) than that of the control at day 10, and lower (P < 0.05) at day 27. The springiness of SS was lower (P < 0.05) than that in the control of day 10, and higher (P < 0.05) at day 27. No pronounced difference (P > 0.05) was obtained for chewiness or cohesiveness between SS and the control during the whole period of processing.
Protein oxidation is related to impaired functionality and altered textural traits of meat products.[Citation33] The texture parameter of dry-cured beef is influenced by other factors, such as L-lysine/L-histidine, pH value, and cathepsin B/L. Firstly, the addition of L-lysine and/or L-histidine may lead to combination with metallic ions, resulting in the dissociation of actomyosin, thus enhancing the gel strength hardness of meat products.[Citation34] Secondly, the introduction of L-lysine and/or L-histidine increased the pH value which improved gel strength.[Citation11] Finally, texture traits were affected by proteolysis which was due to the effect of enzyme activity changing the protein structures of the muscle.[Citation13] The higher activity of cathepsin B in the control was involved in protein degradation which could play a crucial role in the excessive softness defects of dry-cured meat products.[Citation35] García-Garrido et al.[Citation36] found that the residual enzyme activity of cathepsin B + L could be considered as an indicator of textural defects associated with intense proteolysis in Spanish dry-cured hams. The higher content of Tyr () observed in the control suggested that the greater cleavage of the myofibrillar structure was produced by cathepsins in the low pH environment and the action of other enzymes, in turn, are responsible for the production of small peptides and amino acids.[Citation21]
The parameter a*-values, and a decrease in SS, is likely due to lower protein oxidation (), resulting from the decrease in metmyoglobin accumulation. Metmyoglobin accumulation is associated with discoloration of meat surface. Consequently, there were no observable differences in the a*-value of 57-day aged dry-cured beef. This was because nitrosyl myoglobin, and its autoxidation, were not sensitive to changes to the addition of L-lysine and/or L-histidine, as they were the main sources of color in cured meat.[Citation18]
Conclusion
The results of this study showed that protein degradation, color and textural parameters of dry-cured beef were influenced by the use of L-lysine and L-histidine. The addition of L-lysine and L-histidine increased PI, inhibited the activity and gene levels of cathepsin B and L, and reduced the contents of protein carbonyls and thiol group values. Instrumental color parameters of SS dry-cured beef had lower L*-values and a*-values. The consequence from texture profile analysis suggested an effect of the introduction of L-lysine and L-histidine, since SS dry-cured beef was softer than that of the control. In conclusion, the addition of L-lysine and L-histidine could offer a promising alternative to other dry-cured meat products, such as dry-cured pork ham or sausage.
Supplemental Material
Download JPEG Image (97.4 KB)Acknowledgments
The authors would like to thank the National Keypoint Research and Invention Program (2016YFD0401502) for financial support. We would also like to thank Dr. Ron Tume for language help and Dr. Yingqun Nian for his careful revision of this paper. The authors declare that they have no conflict of interest.
Supplementary material
Supplementary data for this article can be accessed here.
Additional information
Funding
References
- Kun, S.; Yu-Miao, L.; Bao-Zhong, S.; Hua-Wei, S.; Hai-Peng, L.; Li, Z.; Yuan-Hua, L.; Hong-Bo, L.; Yang, Z. Changes in Lipid Oxidation, Fatty Acid Profile and Volatile Compounds of Traditional Kazakh Dry-Cured Beef during Processing and Storage. J. Food Process. Preserv. 2017, 41(4), e13059. DOI: 10.1111/jfpp.13059.
- Candek-Potokar, M.; Skrlep, M. Factors in Pig Production that Impact the Quality of Dry-Cured Ham: A Review. Animal. 2012, 6(2), 327–338. DOI: 10.1017/S1751731111001625.
- Inguglia, E. S.; Zhang, Z.; Tiwari, B. K.; Kerry, J. P.; Burgess, C. M. Salt Reduction Strategies in Processed Meat Products – A Review. Trends Food Sci. Technol. 2017, 59, 70–78. DOI: 10.1016/j.tifs.2016.10.016.
- Aliño, M.; Grau, R.; Toldrá, F.; Blesa, E.; Pagán, M. J.; Barat, J. M. Physicochemical Properties and Microbiology of Dry-Cured Loins Obtained by Partial Sodium Replacement with Potassium, Calcium and Magnesium. Meat Sci. 2010, 85(3), 580–588. DOI: 10.1016/j.meatsci.2010.03.009.
- Armenteros, M.; Aristoy, M. C.; Barat, J. M.; Toldrá, F. Biochemical and Sensory Changes in Dry-Cured Ham Salted with Partial Replacements of NaCl by Other Chloride Salts. Meat Sci. 2012, 90(2), 361–367. DOI: 10.1016/j.meatsci.2011.07.023.
- Dos Santos Alves, L. A. A.; Lorenzo, J. M.; Gonçalves, C. A. A.; Dos Santos, B. A.; Heck, R. T.; Cichoski, A. J.; Campagnol, P. C. B. Impact of Lysine and Liquid Smoke as Flavor Enhancers on the Quality of Low-Fat Bologna-Type Sausages with 50% Replacement of NaCl by KCl. Meat Sci. 2017, 123, 50–56. DOI: 10.1016/j.meatsci.2016.09.001.
- Zhu, C. Z.; Zhao, J. L.; Tian, W.; Liu, Y. X.; Li, M. Y.; Zhao, G. M. Contribution of Histidine and Lysine to the Generation of Volatile Compounds in Jinhua Ham Exposed to Ripening Conditions via Maillard Reaction. J. Food Sci. 2018, 83(1), 46–52. DOI: 10.1111/1750-3841.13996.
- Zhang, Y.; Cheng, Q.; Yao, Y.; Guo, X.; Wang, R.; Peng, Z. A Preliminary Study: Saltiness and Sodium Content of Aqueous Extracts from Plants and Marine Animal Shells. Eur. Food Res. Technol. 2014, 238(4), 565–571. DOI: 10.1007/s00217-013-2136-1.
- Guo, X. Y.; Peng, Z. Q.; Zhang, Y. W.; Liu, B.; Cui, Y. Q. The Solubility and Conformational Characteristics of Porcine Myosin as Affected by the Presence of L-Lysine and L-Histidine. Food Chem. 2015, 170, 212–217. DOI: 10.1016/j.foodchem.2014.08.045.
- Campagnol, P. C. B.; Santos, B. A. D.; Morgano, M. A.; Terra, N. N. Application of Lysine, Taurine, Disodium Inosinate and Disodium Guanylate in Fermented Cooked Sausages with 50% Replacement of NaCl by KCl. Meat Sci. 2011, 87(3), 239–243. DOI: 10.1016/j.meatsci.2010.10.018.
- Zhou, C.; Li, J.; Tan, S. Effect of L-Lysine on the Physicochemical Properties of Pork Sausage. Food Sci. Biotechnol. 2014, 23(3), 775–780. DOI: 10.1007/s10068-014-0104-6.
- ISO Determination of nitrogen content. ISO 937:1978 Standard. International standards meat and meat products. International Organization for Standardization: Geneva, Switzerland, 1978.
- Pérez-Santaescolástica, C.; Carballo, J.; Fulladosa, E.; Garcia-Perez, J. V.; Benedito, J.; Lorenzo, J. M. Effect of Proteolysis Index Level on Instrumental Adhesiveness, Free Amino Acids Content and Volatile Compounds Profile of Dry-Cured Ham. Food Res. Int. 2018, 107, 559–566. DOI: 10.1016/j.foodres.2018.03.001.
- Aro Aro, J. M.; Nyam-Osor, P.; Tsuji, K.; Shimada, K.-I.; Fukushima, M.; Sekikawa, M. The Effect of Starter Cultures on Proteolytic Changes and Amino Acid Content in Fermented Sausages. Food Chem. 2010, 119(1), 279–285. DOI: 10.1016/j.foodchem.2009.06.025.
- Armenteros, M.; Heinonen, M.; Ollilainen, V.; Toldrá, F.; Estévez, M. Analysis of Protein Carbonyls in Meat Products by Using the DNPH-method, Fluorescence Spectroscopy and Liquid Chromatography–Electrospray Ionisation–Mass Spectrometry (LC–ESI–MS). Meat Sci. 2009, 83(1), 104–112. DOI: 10.1016/j.meatsci.2009.04.007.
- Cui, C.; Zhou, X. S.; Zhao, M. M.; Yang, B. Effect of Thermal Treatment on the Enzymatic Hydrolysis of Chicken Proteins. Innovative Food Sci. Emerging Technol. 2009, 10(1), 37–41. DOI: 10.1016/j.ifset.2008.09.003.
- Cui, B.; Zhang, Y.; Wang, F.; Jamali, M. A.; Wei, L.; Peng, Z. Technical Note: The Effect of Carcass Deboning Technique on the Meat Quality of Cattle. J. Anim. Sci. 2016, 94(10), 4427. DOI: 10.2527/jas.2016-0550.
- Lorenzo, J. M.; Bermúdez, R.; Domínguez, R.; Guiotto, A.; Franco, D.; Purriños, L. Physicochemical and Microbial Changes during the Manufacturing Process of Dry-Cured Lacón Salted with Potassium, Calcium and Magnesium Chloride as a Partial Replacement for Sodium Chloride. Food Control. 2015, 50, 763–769. DOI: 10.1016/j.foodcont.2014.10.019.
- Petrova, I.; Tolstorebrov, I.; Mora, L.; Toldrá, F.; Eikevik, T. M. Evolution of Proteolytic and Physico-Chemical Characteristics of Norwegian Dry-Cured Ham during Its Processing. Meat Sci. 2016, 121, 243–249. DOI: 10.1016/j.meatsci.2016.06.023.
- Li, S.; Zheng, Y.; Xu, P.; Zhu, X.; Zhou, C. l-Lysine and L-Arginine Inhibit Myosin Aggregation and Interact with Acidic Amino Acid Residues of Myosin: The Role in Increasing Myosin Solubility. Food Chem. 2018, 242, 22–28. DOI: 10.1016/j.foodchem.2017.09.033.
- Arnau, J.; Guerrero, L.; Sarraga, C. The Effect of Green Ham pH and NaCl Concentration on Cathepsin Activities and the Sensory Characteristics of Dry-Cured Hams. J. Sci. Food Agric. 1998, 77(3), 387–392. DOI: 10.1002/(SICI)1097-0010(199807)77:33.0.CO;2-H.
- Armenteros, M.; Aristoy, M. C.; Toldrá, F. Effect of Sodium, Potassium, Calcium and Magnesium Chloride Salts on Porcine Muscle Proteases. Eur. Food Res. Technol. 2009, 229(1), 93–98. DOI: 10.1007/s00217-009-1029-9.
- Zhao, C. J.; Schieber, A.; Gänzle, M. G. Formation of Taste-Active Amino Acids, Amino Acid Derivatives and Peptides in Food Fermentations – A Review. Food Res. Int. 2016, 89, 39–47. DOI: 10.1016/j.foodres.2016.08.042.
- Armenteros, M.; Aristoy, M. C.; Barat, J. M.; Toldrá, F. Biochemical Changes in Dry-Cured Loins Salted with Partial Replacements of NaCl by KCl. Food Chem. 2009, 117(4), 627–633. DOI: 10.1016/j.foodchem.2009.04.056.
- Katsiari, M.; Alichanidis, E.; Voutsinas, L.; Roussis, I. Proteolysis in Reduced Sodium Kefalograviera Cheese Made by Partial Replacement of NaCl with KCl. Food Chem. 2001, 73(1), 31–43. DOI: 10.1016/s0308-8146(00)00275-2.
- Kęska, P.; Stadnik, J. Taste-Active Peptides and Amino Acids of Pork Meat as Components of Dry-Cured Meat Products: An In-Silico Study. J. Sens. Stud. 2017, 32(6), e12301. DOI: 10.1111/joss.12301.
- Tamura, M.; Mori, N.; Miyoshi, T.; Koyama, S.; Kohri, H.; Okai, H. Practical Debittering Using Model Peptides and Related Compounds. Agric. Biol. Chem. 1990, 54(1), 41–51. DOI: 10.1080/00021369.1990.10869906.
- Nyström, T.;. Role of Oxidative Carbonylation in Protein Quality Control and Senescence. Embo J. 2005, 24(7), 1311–1317. DOI: 10.1038/sj.emboj.7600599.
- Kazemi, S.; Ngadi, M. O.; Gariépy, C. Protein Denaturation in Pork Longissimus Muscle of Different Quality Groups. Food Bioprocess. Technol. 2011, 4(1), 102–106. DOI: 10.1007/s11947-009-0201-3.
- Xu, P.; Zheng, Y.; Zhu, X.; Li, S.; Zhou, C. L-Lysine and L-Arginine Inhibit the Oxidation of Lipids and Proteins of Emulsion Sausage by Chelating Iron Ion and Scavenging Radical. Asian-Australas J. Anim. Sci. 2018, 31(6), 905–913. DOI: 10.5713/ajas.17.0617.
- Li, Y.; Jiang, H.; Huang, G. Protein Hydrolysates as Promoters of Non-Haem Iron Absorption. Nutrients. 2017, 9(6), 609. DOI: 10.3390/nu9060609.
- Sun, W.; Cui, C.; Zhao, M.; Zhao, Q.; Yang, B. Effects of Composition and Oxidation of Proteins on Their Solubility, Aggregation and Proteolytic Susceptibility during Processing of Cantonese Sausage. Food Chem. 2011, 124(1), 336–341. DOI: 10.1016/j.foodchem.2010.06.042.
- Estévez, M.;. Protein Carbonyls in Meat Systems: A Review. Meat Sci. 2011, 89(3), 259–279. DOI: 10.1016/j.meatsci.2011.04.025.
- Xiong, Y. L.; Noel, D. C.; Moody, W. G. Textural and Sensory Properties of Low-Fat Beef Sausages with Added Water and Polysaccharides as Affected by pH and Salt. J. Food Sci. 2010, 64(3), 550–554. DOI: 10.1111/j.1365-2621.1999.tb15083.x.
- Russo, V.; Fontanesi, L.; Davoli, R.; Nanni Costa, L.; Cagnazzo, M.; Buttazzoni, L.; Virgili, R.; Yerle, M. Investigation of Candidate Genes for Meat Quality in Dry‐Cured Ham Production: The Porcine Cathepsin B (CTSB) and Cystatin B (CSTB) Genes. Anim. Genet.. 2015, 33(2), 123–131. DOI: 10.1046/j.1365-2052.2002.00835.x.
- Garcíagarrido, J. A.; Quileszafra, R.; Tapiador, J.; Castro, L. D. Activity of Cathepsin B, D, H and L in Spanish Dry-Cured Ham of Normal and Defective Texture. Meat Sci. 2000, 56(1), 1. DOI: 10.1016/S0309-1740(00)00005-X.