ABSTRACT
Cancer is a complex and evolving disease that is the leading cause of death in the twenty-first century, and colorectal and liver cancers specifically are in the top 10 cancer types in terms of incidence and mortality; therefore, there has been a shift towards researching prevention strategies, amongst which chemoprevention is key. Coffee is a natural product that has recently gained importance in this area due to its chemopreventive potential and its high level of consumption worldwide. In this review, recent epidemiological and experimental evidence of the chemopreventive effect of coffee against these two types of cancer are presented, and the role of the different compounds present in coffee in cell proliferation, apoptosis and response to oxidative stress are discussed.
Introduction
Coffee is one of the most commonly consumed beverages worldwide; as such, its effect on human health is of great interest. Until recently, coffee consumption has been believed to be associated with adverse effects; however, a great number of epidemiological and experimental studies have been conducted worldwide over the last decade revealing significant beneficial properties in cancer prevention, longevity, cardiovascular health, liver health, diabetes, and stroke, among others.[Citation1]
Cancer is of particular interest, as according to the International Agency for Research on Cancer, the cancer burden in 2018 has risen to 18.1 million new cases and 9.6 million cancer deaths worldwide[Citation2], which means that cancer is the leading cause of death in the 21st century. Given that cancer is such a burden on public health care, in the scientific community there has been a shift towards researching cancer prevention strategies. One of these approaches is chemoprevention, which is defined as the intake of pharmacologic or dietary agents in order to prevent, suppress, or reverse the initiation or progression of carcinogenesis.[Citation3] Therefore, there is an increasing interest in the chemopreventive effects of coffee on cancer, especially for colorectal cancer (CRC), as the colon is directly exposed to the bioactive compounds in coffee when it is ingested. The effects of coffee consumption on liver health are also of interest, as the liver is the major metabolic organ in the body and as such, substances ingested are first processed by the liver before reaching the systemic circulation. Colorectal and liver cancers are in the top 10 cancer types for estimated cases and deaths worldwide for both men and women.[Citation2] In terms of incidence, CRC is the third most commonly diagnosed cancer (10.2%) and liver cancer is the sixth most diagnosed (4.7%); while CRC is the second cause of death by cancer (9.2%) and liver cancer is the fourth (8.2%). This article presents recent evidence of the chemopreventive effect of coffee against these two types of cancer.
Bioactive compounds in coffee
The green coffee bean is composed of minerals, carbohydrates, lipids, proteins, alkaloids (such as caffeine and trigonelline), and carboxylic and phenolic acids (see ). The health benefits of coffee have been mainly attributed to alkaloids, diterpenes and chlorogenic acids[Citation4]; thereby we will focus on these components in the next sections.
Figure 1. Main components in coffee beans. Percentages correspond to the contribution of each component to the total dry weight. 3-caffeoylquinic acid (3-CQA), 4-caffeoylquinic acid (4-CQA), 5-caffeoylquinic acid (5-CQA), 3,4-dicaffeoylquinic acid (3,4-diCQA), 3,5-dicaffeoylquinic acid (3,5-diCQA), 4,5-dicaffeoylquinic acid (4,5-diCQA)
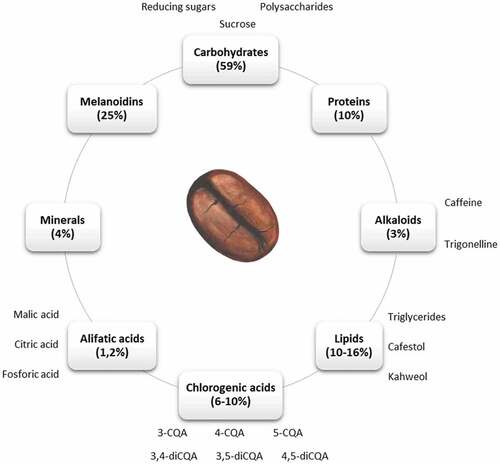
Caffeine (1,3,7- trimethylxantine) is an alkaloid present in coffee, which is almost completely metabolized in the liver, with around 70–80% converted to 1,7-dimethylxanthine (1,7-DMX) by CYP1A2.[Citation5] A wide range of biological effects have been explored for caffeine and related metabolites, including antioxidant, antiproliferative and anti-inflammatory effects. Among others, 1-methylxantine and 1-methyluric acid that are formed from 1,7-DMX have been related to in vitro antioxidant activity.[Citation6,Citation7] Trigonelline is another alkaloid that can be found in green coffee beans, which is partially degraded into other compounds such as nicotinic acid during the roasting process.[Citation8] Nrf2 is a transcription factor that plays a key role in cancer development and chemoresistance; and it has been reported that trigonelline is an effective inhibitor of Nrf2, thus increasing the sensitivity of chemoresistant cell lines to anticancer drugs.[Citation9] Two important diterpenes in the lipid fraction of the coffee bean are kahweol and cafestol. It has been shown that treatment with cafestol and kahweol induces apoptosis of malignant cells in vitro[Citation10], and reduces cell proliferation and migration.[Citation11] There is also increasing evidence that these components counteract oxidative stress.[Citation12]
Chlorogenic acids (CGAs) are esters formed between caffeic and quinic acids, and represent an abundant group of polyphenols present in green and roasted coffee beans. The major CGAs in coffee include 3-caffeoylquinic acid (3-CQA), 4-caffeoylquinic acid (4-CQA), 5-caffeoylquinic acid (5-CQA), 3,4-dicaffeoylquinic acid (3,4-diCQA), 3,5-dicaffeoylquinic acid (3,5-diCQA), and 4,5-dicaffeoylquinic acid (4,5-diCQA).[Citation13] Among these, 5-CQA is the main CGA present in coffee, and accounts for approximately 10 g per 100 g of the coffee bean.[Citation14] There is considerable evidence to show that CGAs have antioxidant, and anti-inflammatory activities.[Citation15] During roasting, different chemical reactions occur which change the content of CGAs in the roasted coffee bean.[Citation8] It has been suggested that caffeic acid (CA) and quinic acid are chemically incorporated into melanoidins during roasting.[Citation16] Melanoidins are molecules that can be formed at high temperatures, due to the Maillard reaction involving polysaccharides, proteins, and CGAs in the coffee bean. These compounds are of interest due to their antioxidant, antimicrobial, anticarcinogenic, anti-inflammatory, antihypertensive, and antiglycative activity.[Citation17]
Colorectal cancer
Overview of colorectal cancer
Current statistics from Globocan 2018 indicate that CRC is the second most prevalent cause of death by cancer in both men and women worldwide, after lung cancer. CRC develops when epithelial cells that line the colon or rectum begin to proliferate uncontrollably due to the accumulation of inherited or acquired gene mutations and epigenetic alterations.[Citation18] In most cases of CRC (60–80%), the DNA mutations that lead to cancer are acquired rather than having been inherited, and often these individuals do not possess a specific genetic marker of susceptibility to this cancer. The remaining 20–40% of cases correspond to familial CRC, in which individuals with family members who have the disease have a greater risk of developing CRC. The most common variants of the inherited type of CRC are familial adenomatous polyposis (FAP) and hereditary non-polyposis colon cancer (HNPCC), which are related to specific mutations in tumor suppressor genes, protoconcogenes, and genes involved in DNA repair.[Citation19]
The intestinal epithelium is a dynamic tissue with a high capacity for regeneration. At the base of the colonic crypt there are stem and progenitor cells that undergo differentiation, and these differentiated cells in the upper part of the crypt eventually undergo apoptosis. The balance between proliferation, differentiation, and apoptosis maintains tissue homeostasis. During the development and progression of CRC a deregulation of cell proliferation and differentiation is observed, as well as a progressive inhibition of apoptosis, which is an important mechanism by which colonocytes with DNA damage escape normal mechanisms of elimination and thus continue to replicate to become invasive tumors.[Citation20]
There are many factors that are known to increase the risk of CRC, some of which are modifiable and others are not modifiable. Non-modifiable risk factors include a family history of CRC or FAP, a personal history of inflammatory bowel disease, racial and ethnic background (African Americans and Ashkenazi Jews), and having type 2 diabetes. On the other hand, the modifiable or lifestyle-related risk factors include physical inactivity, obesity, certain types of diets, smoking and heavy alcohol consumption.
Diet is a very influential risk factor in the development of CRC, and it is estimated that changes in eating habits could reduce the incidence of this type of cancer by up to 70%.[Citation21] N-nitroso compounds (NOCs) are potential human carcinogens which are found in processed meats or can be formed endogenously in the bowel by the bacterial flora as it degrades bile salts into nitrosamines and nitrosamides, a process which is also promoted by heme found in red meat.[Citation22] Therefore, there is a correlation between a diet high in animal fats, and red or processed meats, and the incidence of CRC.[Citation23] It has also been reported that cooking meats at very high temperatures generates heterocyclic amines and polycyclic aromatic hydrocarbons, which are believed to have carcinogenic properties.[Citation24] Nowadays, it is well known that diets high in vegetables, fruits, and whole grains are essential for bowel health, and both epidemiological and experimental studies have reported that these types of foods may lower the risk of CRC.[Citation25]
Epidemiological evidence
In the literature, there is ample epidemiological evidence of the chemopreventive effect of coffee against CRC, summarized in . An epidemiological study which included 816 cases of CRC and 815 controls, reported a significant inverse association between the consumption of coffee or polyphenols derived from coffee and CRC, especially in distal colon cancer.[Citation26] Similarly, an analysis of two case-control studies in Japanese population reported a 22% reduction in risk of CRC with three or more cups of coffee a day, especially for distal colon cancer and rectal cancer, suggesting the possibility of a heterogeneous effect of coffee on CRC by anatomical subsite.[Citation27] In 2012, another important epidemiological study on the relationship between coffee consumption and CRC was published.[Citation28] In this study, 489,706 men and women were followed for 10 years, during which time 2,863 cases of proximal colon cancer, 1,993 cases of distal colon cancer, and 1,874 cases of rectal cancer were reported. Those participants who drank more than four cups of coffee per day exhibited a lower risk of developing CRC, especially proximal tumors. These results were the same in the participants who predominantly consumed decaffeinated coffee.
Table 1. Epidemiological association between coffee and CRC
The American Cancer Society’s Cancer Prevention Study-II (CPS-II) is a recently published prospective cohort study of 1.2 million participants between 28 and 94 years of age, who were cancer-free at baseline in 1982 and followed through to 2012. Cox proportional hazards regression was used to estimate the association between coffee consumption and death from all cancer types. These analyses showed a nonlinear association between coffee consumption and death from cancer in smokers. Among non-smokers, a two-cup/day increase in coffee consumption was inversely associated with death from CRC.[Citation29] This highlights the importance of taking smoking status into account when assessing the association between coffee consumption and cancer mortality. In another recently published study, a meta-analysis was performed with 19 studies involving 2,046,575 participants and 22,629 patients with CRC. The authors reported that for every four cups of coffee consumed per day the risk of CRC decreased by 7%; however, a higher intake of coffee showed an increased risk, so this seems to be a dose-dependent response.[Citation30]
A case-control study of confirmed CRC cases and their matched controls from an area in northern Israel demonstrated that at the highest level of coffee consumption measured (>2.5 cups per day) there was a 54% reduction in the risk of developing CRC.[Citation31] This result was consistent for decaffeinated and boiled coffees, but not for instant or filtered coffee. Stratification according to anatomical subsite showed that results were the same for colon and rectal cancers. As in this geographical area there are several ethnic groups represented, this was also analyzed. Arabs reported the highest level of consumption (3.3 cups per day), followed by Sephardi Jews (2.1 cups per day), and finally by Ashkenazi Jews (1.8 cups per day). The statistically significant inverse dose-response relation between coffee and risk of CRC was maintained in the two largest ethnic subgroups (Ashkenazi and Sephardi Jews), but was limited in the Arab population, indicating that ethnic background may influence the chemoprotective effect of coffee. For example, studies in Japanese population have shown mixed results. A recent meta-analysis of 26 studies carried out in Japan, found no statistically significant association between coffee consumption and risk of CRC in 14 cohort studies, while in the remaining 12 case-control studies there was only a weak association.[Citation32] A meta-analysis of 21 cohort studies of CRC found a similar response.[Citation33]
A study carried out in Japan followed 320,322 participants, of which 6,711 developed CRC. In this study women who drank three or more cups of coffee a day exhibited a lower risk than those who did not consume coffee, and this protective effect seemed to be greater in women in the postmenopausal stage; however, no association was observed in the male participants.[Citation34] It is suggested that this protective effect in women is a result of the action of coffee on the metabolism of estrogen. An in vitro study on the effect of coffee on the expression of estrogen sulfotransferases (SULT), proteins that inactivate estrogen by sulfation, showed a 60% reduction in the expression of SULT1E1 in Caco-2 colorectal carcinoma cells treated with coffee for 24 h.[Citation37] Therefore, the daily consumption of coffee can reduce the activity of estrogen sulfotransferases thus improving the activity of estrogen in the colon. However, contradicting results were reported in a large observational cohort of postmenopausal women in the United States in which participants were grouped as non-drinkers, moderate (1–4 cups per day) and high (>4 cups per day) drinkers.[Citation35] Compared to non-drinkers, moderate and high coffee drinkers showed an increased incidence of CRC in the multivariate analysis.
Remarkably, it seems that coffee is not only chemopreventive but is also beneficial for patients who already have CRC. An American prospective study following 953 patients with stage III colon cancer analyzed the influence of coffee on cancer recurrence and mortality during and 6 months after adjuvant chemotherapy.[Citation36] Patients who consumed four or more cups of coffee a day exhibited a significantly reduced risk of cancer recurrence or mortality compared to non-coffee drinkers. Non-herbal tea and decaffeinated coffee drinkers, however, did not experience this risk reduction.
Experimental evidence
As previously discussed, the results of the large epidemiological studies show that coffee consumption reduces the risk of developing CRC, however, it is important to elucidate the molecular mechanisms involved in this chemopreventive effect. It has been proposed that the inverse association between coffee consumption and colon cancer could be explained, at least in part, by the effect of CGAs[Citation38], which when ingested are metabolized to CA, among other molecules, in the colon.[Citation39,Citation40] In 2012, Oleaga and colleagues published a paper in which they explored the effect of coffee on gene expression, using microarrays. When HT29 cells were treated with instant (caffeinated) coffee, 57 genes were found to be upregulated, and 161 genes were downregulated. On the other hand, treatment with CA alone induced the overexpression of 12 genes and reduced the expression of 32.[Citation41] Among the over-expressed genes, 33% were transcription factors, 25% were involved in cell cycle, and 16.7% were genes involved in processes of biosynthesis or immune response. Among the downregulated genes, 30% were cell cycle genes, 15% were involved in biosynthesis processes and 12% were transcription factors.
Experimental studies using in vitro and in vivo models of CRC have shown that coffee affects cell cycle progression, proliferation, and apoptosis, in a dose-dependent manner (see ). In Caco-2 cells treated with roasted and ground coffee infusions, proliferation was completely inhibited at a concentration of 3.75% without affecting cell viability.[Citation42] However, this effect was not observed when the cells were treated with caffeine, CA or CGA. Cells treated with the coffee infusion also exhibited a reduced expression of KRAS, which is important as CRC is associated with the abnormal activation of the KRAS signaling pathway.[Citation52] When the degree of roasting was increased the reduction in KRAS was more prominent, suggesting that melanoidins formed during roasting may be responsible for this effect.
Table 2. Experimental evidence of the chemopreventive effect of coffee against CRC
In a very recently published study, the mechanism of chemoprevention by p-coumaric acid (p-CA), a hydroxycinnamic acid present in coffee beans, was explored in rats.[Citation43] The authors reported that in rats supplemented with p-CA there was a downregulation in proteins involved in cell cycle and proliferation; however, proteins involved in detoxification and apoptosis were up-regulated. These results explain how p-CA present in coffee reduces polyp formation in an animal model. A similar effect on apoptosis has been observed in an in vitro model of CRC. In HCT-15 and HT-29 cells treated with p-CA more cells were arrested at sub-G1 in the cell cycle analysis, which resulted in a reduction in cell proliferation and colony formation.[Citation44] Additionally, mitochondrial membrane potential diminished and ROS formation increased, leading to an increase in apoptosis.
Kahweol, a coffee-specific diterpene, has a similar effect. In human CRC cell lines (HCT116, SW480, LoVo, and HT-29) treated with kahweol, expression of activating transcription factor 3 (ATF3) increased proportionally to the dose of kahweol used.[Citation45] ATF3 expression contributed to kahweol-mediated apoptosis, although the molecular mechanism has not been fully elucidated. Another study with HT-29 human colon adenocarcinoma cells reported that treatment with kahweol increased the expression of caspase-3, which is a pro-apoptotic factor, and decreased the expression of anti-apoptotic factors, such as Bcl-2 and phosphorylated Akt, as well as reducing the expression of 70-kDa heat shock proteins (HSP70), all of these leading to an increase in apoptosis.[Citation46]
CA derivatives also promote apoptosis in human colon cancer cells HCT-116 and SW-480 in a dose-dependent manner.[Citation47] These cells treated with caffeic acid phenethyl ester (CAPE) and caffeic acid phenylpropyl ester (CAPPE) presented G0/G1 cell cycle arrest and thus an inhibition of proliferation. In order to explore the molecular mechanisms by which cell cycle arrest is induced, the authors measured the effects of CAPE and CAPPE on the PI3-K/Akt, mTOR, and AMPK signaling pathways. Treatment with the CA derivatives inhibited phosphorylation of PDK1, Akt, and mTOR, whereas the expression of 14–3-3 protein, N-cadherin and phosphorylation of the FOXO3 proteins were upregulated. To verify these findings, the effects of CAPE and CAPPE were measured on the growth of HCT-116 cells in a mouse xenograft model. By the end of the study period, mice who were supplemented with CAPE or CAPPE had reduced tumor weights compared to the control group.
Many in vitro and in vivo studies have also shown that compounds present in coffee have an anti-inflammatory effect on the intestinal epithelium. Recently, human intestinal Caco-2 cells were treated with the six major CGA isomers: 3-caffeoylquinic acid (3-CQA), 4-caffeoylquinic acid (4-CQA), 5-caffeoylquinic acid (5-CQA), 3,4-dicaffeoylquinic acid (3,4-diCQA), 3,5-dicaffeoylquinic acid (3,5-diCQA), and 4,5-dicaffeoylquinic acid (4,5-diCQA), and then challenged with inflammatory mediators.[Citation48] Oxidative stress was measured and was found to be reduced in cells treated with the CGA isomers, specially by diCQA. In these cells, ROS was reduced, reduced glutathione (GSH) was increased, and the Nrf2 signaling pathway was activated leading to upregulation of GPX2, KEAP1, and NFE2L2, which are Nrf2 target genes. These results confirm that CGA isomers present in coffee lessen oxidative stress in in vitro models of CRC. In a similar study[Citation49], Caco-2 cells were treated with lyophilized coffee infusions and then exposed to oxidative stress. In cells pretreated with coffee, ROS values decreased as compared to non-treated cells, thus increasing cell viability. Furthermore, Caco-2 cells treated with CGA and CA exhibited a reduction in H2O2-induced ROS, a reduction in both IL-8 and nuclear factor kappa-light-chain-enhancer of activated B cells (NF-κB) transcriptional activity, suppressed nuclear translocation of the p65 subunit, phosphorylation of IκB kinase (IKK), and protein kinase D (PKD).[Citation50] Therefore, CGA and CA suppress PKD-NF-κB signaling. The anti-inflammatory effect of CGA on IL-8 production had previously been shown in Caco-2 cells, and C57BL/6 mice with induced colitis symptoms.[Citation51] This study showed that in Caco-2 cells, NFα and IL-8 production induced by exposure to H2O2 were reduced in cells treated with CGA; furthermore, in the animal model mRNA expression of proinflammatory cytokines (colonic macrophage inflammatory protein 2 and IL-1β) was downregulated in mice with CGA supplementation, thus suggesting that dietary CGA reduces intestinal inflammation.
Hepatocellular carcinoma
Overview of hepatocellular carcinoma
Liver cancer is the fifth most diagnosed cancer worldwide with an increasing incidence each year, making it the second cause of cancer-related death globally. Hepatocellular carcinoma (HCC) represents the major histologic type of primary liver cancer, accounting for 70% to 85% of the total liver cancer burden worldwide.[Citation53] In HCC, hepatocytes accumulate somatic genetic alterations that combine mutations in both driver and passenger genes.[Citation54] Distribution of HCC closely follows the global distribution of HBV and HCV infection, with the highest rates of HCC seen in those regions where these infections are endemic. Considering this, the major risk factors for developing HCC are infection by HBV or HCV, followed by alcoholic liver disease, and nonalcoholic fatty liver disease (NAFLD).[Citation55] Most of these risk factors lead to chronic hepatitis and cirrhosis, which is present in 80–90% of HCC patients[Citation56], which generate a tissue microenvironment of inflammation and increased proliferation that affects the normal physiology of the hepatocytes, which in normal circumstances have a low turnover rate and a life expectancy of more than a year. In response to parenchymal cell loss due to injury, the liver can regenerate by proliferation of the main epithelial cell compartments (hepatocytes and cholangiocytes), followed by proliferation of mesenchymal cell types (hepatic stellate cells) and endothelial cells[Citation53], which triggers a progressive accumulation of extracellular matrix (ECM) components enriched in collagen.[Citation57]
Hepatic stellate cells (HSCs) are considered to be the main producers of ECM during liver fibrosis, as upon acute or chronic liver injury, a complex network of autocrine/paracrine fibrogenic signals promotes transdifferentiation of quiescent HSCs to a myofibroblastic phenotype.[Citation57] Paracrine factors such as platelet-derived growth factor (PDGF), transforming growth factor β (TGFβ), and connective tissue growth factor (CTGF) activate signaling pathways of cell proliferation, migration, ECM protein secretion, and contractility that promote transdifferentiation of precursor mesenchymal cells. Proliferating myofibroblasts are the key source of excess ECM molecules such as collagen type I and III, as well as other proteins that constitute pathologic fibrous tissues with fibrotic nodules characteristic of cirrhosis, setting the stage for dysplastic nodules and epigenetic and genetic changes resulting in the development of HCC.[Citation55,Citation58]
Hepatocarcinogenesis is a process connected to the disruption of several signaling pathways involved in cell survival, proliferation, differentiation, and apoptosis, like MAPK-ERK, TGF-β y Wnt/β-catenin. These signaling cascades in hepatoma cells are usually interconnected and are affected by the aberrant expression of proto-oncogenes or loss of tumor suppressor genes, like p53 and retinoblastoma (Rb). Dysregulation of these genes results in distinct cell-cycle checkpoints or resistance to apoptosis. In addition, genomic instability is common due to DNA damage caused by oxidative stress, defects in chromosome segregation and alterations in the length of telomeres.[Citation56]
Epidemiological evidence
Cohort and case-control studies have reported an inverse association between coffee consumption and the degree of liver fibrosis as well as the development of liver cancer[Citation59] and these beneficial effects have been confirmed by large meta-analyses (summarized in ). A protective effect derived from coffee consumption has been observed in case and control population studies and meta-analysis between 2005 and 2008.[Citation60] Several of these important works have been carried out in Japan, all of them reporting a protective effect. One such cohort study found 90,452 individuals, of whom 250 men and 84 women developed HCC after 10 years of follow-up.[Citation61] When comparing the rate of HCC in this cohort, those who did not consume coffee had a higher rate (547.2 vs. 214.6 per 100,000 people). Those who reported regular coffee consumption were 50% less likely to develop HCC compared to those who did not report higher consumption (hazards ratio (HR) = 0. 49, 95% CI = 0.36–0.66). Additionally, an association dependent on frequency of consumption was observed in this study; specifically, the HR for 1–2 cups per day was 0.52 (95% CI = 0. 38–0.73), for 3–4 cups per day was 0.48 (95% CI = 0.28–0.83), and greater five cups per day was 0.24 (95% CI = 0. 08–0.77).
Table 3. Epidemiological evidence of the chemopreventive effect of coffee against HCC
The first meta-analysis that quantitatively assessed the relation between coffee consumption and the risk of liver cancer was published by Larsson & Wolk in 2007, that included nine studies (four cohort and five case-control studies) from Japan and southern Europe (Italy and Greece), involving a total of 2,260 cases of HCC and 239,146 healthy individuals of both sexes. All studies reported an inverse relationship between coffee consumption and risk of HCC, but in only six studies was this association statistically significant.[Citation62] This result is consistent with population studies and in vivo models that support that coffee protects against liver damage, an effect determined by measuring transaminase enzymes and a lower risk of developing cirrhosis, an injury that predisposes to the appearance of HCC.[Citation67]
In a recent meta-analysis of eight cohort, and eight case-control studies, including a total of 3,153 cases of HCC, Bravi and colleagues found that the risk of HCC was reduced by 40% for any coffee consumption versus no consumption.[Citation63] They reported a Risk Ratio (RR) of 0.60 (95% CI: 0.50–0.71) in coffee drinkers compared to non-drinkers, with similar results in case-control (RR: 0.56, 95% CI: 0.42–0.75) and cohort studies (RR: 0.64, 95% CI: 0.52–0.78). Compared with no coffee consumption, the summary RR was 0.72 (95% CI, 0.61–0.84) for low consumption and 0.44 (95% CI, 0.39–0.50) for high consumption. In addition, a risk reduction was observed in both patients at low risk of HCC or at high risk (chronic carriers of hepatitis B and C markers, and heavy alcohol drinkers), and in studies from Europe, North America, and Asia. The inverse relationship between coffee and HCC risk was consistent regardless of the subjects’ sex, alcohol consumption, or history of hepatitis or liver disease.
A large meta-analysis published in 2016, included nine cohort studies measuring the relation between high versus low coffee intake and HCC, and seven dose-response studies. The summary RR was 0.46 (95% CI = 0.37–0.57, P = 0) suggesting an inverse association between coffee consumption and liver cancer incidence. However, an analysis by gender revealed there was no significant relationship between coffee intake and the risk of liver cancer in women. The dose-response analysis showed a statistically significant inverse association (RR = 0.73, 95% CI = 0.67–0.79) with an increased intake of two cups of coffee per day.[Citation33]
In a more recent meta-analysis of 12 cohort studies, including 3,414 cases of HCC, the same researchers reported a RR of 0.66 (95% CI: 0.55–0.78) in habitual coffee drinkers compared with non-drinkers or occasional drinkers.[Citation64] The RR was 0.78 (95% CI: 0.66–0.91) in patients reporting moderate coffee consumption and 0.50 (95% CI: 0.43–0.58) in patients reporting high consumption. The same meta-analysis also considered five studies on cirrhosis, a condition associated with the pathogenesis of HCC. On the basis of a total of more than 1400 cases, the RR of cirrhosis was 0.62 (95% CI: 0.47–0.82) in habitual coffee drinkers compared with non-drinkers or occasional drinkers, 0.72 (95% CI: 0.59–0.88) in moderate drinkers, and 0.35 (95% CI: 0.22–0.56) in heavy drinkers.
Another meta-analysis including 18 cohorts and 8 case-control studies, reported a reduction of 27% (RR 0.73, 95% CI 0.63 to 0.85) and 14% (RR 0.86, 95% CI 0.74 to 1.00) in the risk of HCC in participants who drank two cups of caffeinated or decaffeinated coffee, respectively. This study included participants with pre-existing liver disease, which did not significantly alter the relation between coffee consumption and risk of HCC.[Citation65]
In a nested case-control study that included 125 cases of HCC and 250 controls, 1 cup of coffee a day was associated with a lower risk of HCC (RR: 0.87, 95% CI: 0.77, 0.98). An intake of ≥4 cups of coffee a day compared with <2 cups, the multivariable-adjusted RR was 0.25 (95% CI: 0.11, 0.62; P-trend = 0.006). Interestingly, coffee intake was positively associated with C-peptide and inversely associated with IL-6, GLDH, ALT, AST, GGT, alkaline phosphatase, total bilirubin, and AFP, which are biomarkers associated with a higher risk of HCC.[Citation66]
Experimental evidence
Many in vitro and in vivo studies have also shown that compounds present in coffee have an anti-inflammatory effect, as well as a protective effect against liver fibrosis and HCC progression, which will be discussed in this section, and summarized in . One of the main alkaloids present in coffee is caffeine, for which a wide range of biological effects have been explored, including antioxidant, antiproliferative and anti-inflammatory. In a study where the effects of caffeine were evaluated in hepatic stellate cells (HSCs), it was reported that treatment with caffeine increased apoptosis, increased the expression of intracellular F-actin and cAMP, and inhibited the expression of procollagen type Ic and α-SMA; which suggests that caffeine can attenuate the progression of liver fibrosis by inhibiting HSCs adhesion and activation.[Citation68] The authors confirmed these results in a rat model where caffeine decreased peri-portal inflammation, levels of inflammatory cells and fibrosis. Another study testing an oral sustained release hepatoprotective formula with caffeine showed a 36% reduction in cell viability of human hepatocellular carcinoma cells (HEPG2).[Citation69] Additionally, measurement of liver enzymes (ALT and AST), oxidative stress biomarkers and inflammatory marker protein kinase C, in a mouse model of carbon tetrachloride-induced liver fibrosis, showed that treatment with the caffeine formula reduced these levels significantly. However, evidence is mixed, as another study reports that caffeine has no protective effect against oxidative stress in human hepatocellular cells.[Citation70] In this study, HepG2 cells were pretreated with either a green coffee bean extract, hydroxycinnamic acids (5-CQA and 3,5-DCQA), or caffeine prior to inducing oxidative stress with tert-butylhydroperoxide (t-BOOH). Pre-treatment with the green coffee bean extract or the hydroxycinnamic acids prevented cellular and macromolecular damage induced by t-BOOH, reduced cytotoxicity and ROS levels, increased GSH levels, and reduced levels of antioxidant enzymes (GPx and GR). However, none of these effects against oxidative stress and inflammatory response were observed when cells were treated with caffeine.
Table 4. Experimental evidence of the chemopreventive effect of coffee against hepatocellular carcinoma
Another component found in coffee that has been found to ameliorate liver inflammation is kahweol. A recently published study reported a protective effect by inhibiting liver inflammation in primary Kupffer cells and primary hepatocyte cultures of C57BL/6 rats.[Citation71] After inducing inflammation with Lipopolysaccharide (LPS), treatment with kahweol decreased production of pro-inflammatory cytokines such as interleukin (IL)-1, IL-6, and tumor necrosis factor-α (TNF-α), and decreased phosphorylated NFκB level. This is supported by the findings from another study by the same authors, where kahweol treatment was shown to reduce hepatic fibrosis in a thioacetamide (TAA)-induced hepatic fibrosis mouse model, as well as in the mouse hepatocyte AML-12 cell line and the LX2 human hepatic stellate cell line.[Citation72] Connective tissue growth factor (CTGF) and type I collagen are known to be overexpressed in fibrotic liver disease, and are stimulated by TGF-β. Treatment with kahweol decreased TGF-β, thus inhibiting CTGF expression and type I collagen accumulation. Markers of liver damage, such as serum ALT and AST were also reduced post-treatment with kahweol. Additionally, kahweol significantly decreased the expression and phosphorylation of activator of transcription 3 (STAT3), extracellular signal-regulated kinases (ERK), and c-Jun N-terminal protein kinase (JNK), which are involved in fibrogenic signals that trigger CTGF expression.
Chlorogenic acid (CGA) represents the major phenolic component found in coffee. CGAs are mainly esters of quinic acid with caffeic, ferulic, or coumaric acids. A recent study reported that in HepG2 cells treated with CGA, above 250 μM, cell viability dropped by more than half, and cell cycle analysis showed that cells were arrested in the S-phase.[Citation73] Immunodeficient mice with HepG2 xenografts were treated with CGA and exhibited a significant reduction in tumor progression (up to 90%). Treatment with CGA remarkably inhibited phosphorylation of ERK1/2 in both HepG2 cells and the nude mice, which is consistent with the key role that the MAPK signaling pathway plays in proliferation. Expression of matrix metalloproteinases (MMPs) was also reduced in cells and mice treated with CGA, indicating that there is a reduction in the degradation of the ECM, and therefore in tumor growth and invasion. CGA has also been shown to increase the efficacy of chemotherapeutic agents, such as fluorouracil (5-FU). In a study with HepG2 and HepG3 human HCC cells, treatment with 5-FU reduced cell viability to 62.75%, while treatment with CGA alone reduced viability to 38%, which is an even greater effect.[Citation74] A combined treatment approach with 5-FU and CGA enhanced the effect and reduced cell viability up to 33.43%. Treatment with 5-FU induces significant ROS production, which was even more prominent when combined with CGA. One type of stress that induces activation of the MAPK pathway is oxidative stress caused by ROS, so the authors evaluated phosphorylation of ERK. Treatment with 5-FU or CGA alone presented no changes in ERK1/2; however, when cells were treated with a combination of 5-FU and CGA phosphorylation of ERK1/2 was significantly reduced, most likely due to the increase in ROS production.
Molecular mechanism
To date, the molecular mechanisms that explain the chemoprotective effect of coffee have not been widely described, however, we know that in part this effect is mediated by major components such as CGAs, caffeine, cafestol, and kahweol, as well as melanoidins in the roasted coffee bean. In we present an overview of the molecular mechanisms involved in the chemoprotective effect of coffee in CRC and HCC. Based on experimental studies it is known that coffee influences oxidative stress mechanisms, reducing ROS production and increasing mechanisms of ROS scavenging, as well as reducing the expression of inflammatory cytokines. When levels of oxidative stress are high, coffee induces cell cycle arrest which in turn reduces cell proliferation, and activates apoptosis.
Figure 2. Overview of the molecular mechanisms involved in the chemoprotective effect of coffee in CRC and HCC. The main experimental findings related to caffeine, chlorogenic acids and/or coffee diterpenes are summarized. Liver-specific effects are shown on the right and CRC specific effects are shown on the left. Oxidative stress mechanisms play a central role in the chemopreventive effect of coffee, in the negative regulation of cell proliferation and tumor growth, as well as increasing apoptosis and autophagy
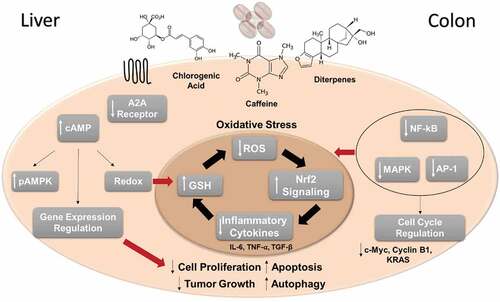
Conclusion
Coffee is one of the most consumed beverages worldwide. It is a complex mixture of bioactive compounds, many of which have been shown to have beneficial health effects, specifically in terms of chemoprevention. Epidemiological studies suggest a dose-dependent effect in reducing the risk of developing CRC and HCC. Caffeinated coffee seems to have an enhanced effect as compared to decaffeinated coffee, which demonstrates that caffeine plays a role in this chemoprotective effect, although it may not be the main responsible component. According to experimental studies, coffee polyphenols, such as CGAs, seem to be the key players in promoting antioxidant, anti-inflammatory, antiproliferative and pro-apoptotic effects in human cells. Treatment with these components results in a decrease in markers of inflammation and oxidative stress. Specific diterpenes of coffee such as kahweol and other phenolic compounds such as CA and FA promote expression of pro-apoptotic molecules and decrease the expression of apoptosis inhibitors, as well as promoting cell cycle arrest, thus they have pro-apoptotic and anti-proliferative effects in cancerous cells. Additionally, these compounds have been shown to regulate multiple signaling pathways including KRAS, PI3K/AKT/mTOR, TGF-B, MAPK-ERK, and Wnt/B-catenin. All these effects, in sum, support the antitumor effect of coffee. However, more studies into the biological mechanisms, as well as clinical trials, are needed to further understand the mechanisms by which coffee prevents tumor development and progression and whether molecular targets can be identified.
Acknowledgments
This work was supported by the Instituto Tecnológico Metropolitano and by a Colciencias grant (Project Code: 115077758163 CT 693-2018). All authors participated in the elaboration this article, including selection, reading, and critical analysis of all articles used as reference, as well as in writing and proofreading. The authors declare no conflict of interest.
Additional information
Funding
References
- Poole, R.; Kennedy, O. J.; Roderick, P.; Fallowfield, J. A.; Hayes, P. C.; Parkes, J. Coffee Consumption and Health: Umbrella Review of Meta-Analyses of Multiple Health Outcomes. Br. Med. J. 2017, 359, 1–17. DOI: 10.1136/bmj.j5024.
- Bray, F.; Ferlay, J.; Soerjomataram, I.; Siegel, R. L.; Torre, L. A.; Jemal, A. Global Cancer Statistics 2018: GLOBOCAN Estimates of Incidence and Mortality Worldwide for 36 Cancers in 185 Countries. CA Cancer J. Clin. 2018, 68, 394–424. DOI: 10.3322/caac.21492.
- Dunn, B. K.; Umar, A.; Richmond, E. Introduction: Cancer Chemoprevention and Its Context. Semin. Oncol. 2016, 43, 19–21. DOI: 10.1053/j.seminoncol.2015.11.002.
- Gaascht, F.; Dicato, M.; Diederich, M. Coffee Provides a Natural Multitarget Pharmacopeia against the Hallmarks of Cancer. Genes Nutr. 2015, 10, 1–17. DOI: 10.1007/s12263-015-0501-3.
- Thorn, C. F.; Aklillu, E.; McDonagh, E. M.; Klein, T. E.; Altman, R. B. PharmGKB Summary: Caffeine Pathway. Pharmacogenet. Genomics. 2012, 22, 389–395. DOI: 10.1097/FPC.0b013e3283505d5e.
- Miller, M. G.; Shukitt-Hale, B. Coffee and Alzheimer’s Disease: Animal and Cellular Evidence; Wiley-Blackwell: Coffee, 2012, pp 77–96. DOI: 10.1002/9781119949893.ch4.
- Farah, A.;. Coffee Constituents. In Coffe Emerg. Heal. Eff. Dis. Prev. First Edit; John Wiley & Sons I, Ed.; Blackwell Publishing Ltd:Hoboken, NJ 2012; pp 21–58
- Furihata, K.; Tanokura, M.; Koda, M.; Wei, F.; Hu, F.; Miyakawa, T. Roasting Process of Coffee Beans as Studied by Nuclear Magnetic Resonance: Time Course of Changes in Composition. J. Agric. Food Chem. 2012, 60, 1005–1012. DOI: 10.1021/jf205315r.
- Arlt A, Sebens S, Krebs S, Geismann C, Grossmann M, Kruse M-L, Schreiber S, Schäfer H. Inhibition of the Nrf2 Transcription Factor by the Alkaloid Trigonelline Renders Pancreatic Cancer Cells More Susceptible to Apoptosis through Decreased Proteasomal Gene Expression and Proteasome Activity. Oncogene 2013, 32, 4825–4835. DOI: 10.1038/onc.2012.493.
- Lee, K.-A.; Chae, J.-I.; Shim, J.-H. Natural Diterpenes from Coffee, Cafestol and Kahweol Induce Apoptosis through Regulation of Specificity Protein 1 Expression in Human Malignant Pleural Mesothelioma. J. Biomed. Sci. 2012, 19, 60. DOI: 10.1186/1423-0127-19-60.
- Moeenfard M, Cortez A, Machado V, Costa R, Luís C, Coelho P, Soares R, Alves A, Borges N, Santos A. Anti-Angiogenic Properties of Cafestol and Kahweol Palmitate Diterpene Esters. J. Cell. Biochem. 2016, 117, 2748–2756. DOI: 10.1002/jcb.25573.
- Lee, K. J.; Choi, J. H.; Jeong, H. G. Hepatoprotective and Antioxidant Effects of the Coffee Diterpenes Kahweol and Cafestol on Carbon Tetrachloride-Induced Liver Damage in Mice. Food Chem. Toxicol. 2007, 45, 2118–2125. DOI: 10.1016/j.fct.2007.05.010.
- Crozier, A.; Jaganath, I. B.; Clifford, M. N. Dietary Phenolics: Chemistry, Bioavailability and Effects on Health. Nat. Prod. Rep. 2009, 26, 1001. DOI: 10.1039/b802662a.
- Perrone, D.; Donangelo, R.; Donangelo, C. M.; Farah, A. Modeling Weight Loss and Chlorogenic Acids Content in Coffee during Roasting. J. Agric. Food Chem. 2010, 58, 12238–12243. DOI: 10.1021/jf102110u.
- Liang, N.; Kitts, D. D. Role of Chlorogenic Acids in Controlling Oxidative and Inflammatory Stress Conditions. Nutrients. 2015, 8. DOI: 10.3390/nu8010016.
- Bekedam, E. K.; Loots, M. J.; Schols, H. A.; Van Boekel, M. A.; Smit, G. Roasting Effects on Formation Mechanisms of Coffee Brew Melanoidins. J. Agric. Food Chem. 2008, 56, 7138–7145. DOI: 10.1021/jf800999a.
- Moreira, A. S. P.; Nunes, F. M.; Domingues, M. R.; Coimbra, M. A. Coffee Melanoidins: Structures, Mechanisms of Formation and Potential Health Impacts. Food Funct. 2012, 3, 903. DOI: 10.1039/c2fo30048f.
- Mundade, R.; Imperiale, T. F.; Prabhu, L.; Loehrer, P. J.; Lu, T. Genetic Pathways, Prevention, and Treatment of Sporadic Colorectal Cancer. Oncoscience. 2014, 1, 400–406. DOI: 10.18632/oncoscience.
- Arvelo, F.; Sojo, F.; Cotte, C. Biology of Colorectal Cancer. Ecancermedicalscience. 2015, 9, 520. DOI: 10.3332/ecancer.2015.520.
- Watson, A. J. M.;. Apoptosis and Colorectal Cancer. Gut. 2004, 53, 1701–1709. DOI: 10.1136/gut.2004.052704.
- Willett, W. C.;. Diet and Cancer: An Evolving Picture. JAMA. 2005, 293, 233–234. DOI: 10.1001/jama.293.2.233.
- Tricker, A. R.;. N-Nitroso Compounds and Man: Sources of Exposure, Endogenous Formation and Occurrence in Body Fluids. Eur. J. Cancer Prev. 1997, 6, 226–268. DOI: 10.1097/00008469-199706000-00003.
- Loh, Y. H.; Jakszyn, P.; Luben, R. N.; Mulligan, A. A.; Mitrou, P. N.; Khaw, K.-T. N-Nitroso Compounds and Cancer Incidence: The European Prospective Investigation into Cancer and Nutrition (Epic)–Norfolk Study. Am. J. Clin. Nutr. 2011, 93, 1053–1061. DOI: 10.3945/ajcn.111.012377.
- Santarelli, R. L.; Pierre, F.; Corpet, D. E. Processed Meat and Colorectal Cancer: A Review of Epidemiologic and Experimental Evidence. Nutr. Cancer. 2008, 60, 131–144. DOI: 10.1080/01635580701684872.
- Aune, D.; Chan, D. S. M.; Lau, R.; Vieira, R.; Greenwood, D. C.; Kampman, E.; Norat, T. Dietary Fibre, Whole Grains, and Risk of Colorectal Cancer: Systematic Review and Dose-Response Meta-Analysis of Prospective Studies. BMJ. 2011, 343, d6617. DOI: 10.1136/bmj.d6617.
- Wang, Z.-J.; Ohnaka, K.; Morita, M.; Toyomura, K.; Kono, S.; Ueki, T.; Tanaka, M.; Kakeji, Y.; Maehara, Y.; Okamura, T.;, et al. Dietary Polyphenols and Colorectal Cancer Risk: The Fukuoka Colorectal Cancer Study. World J. Gastroenterol. 2013, 19, 2683–2690. DOI: 10.3748/wjg.v19.i17.2683.
- Nakagawa-Senda, H.; Ito, H.; Hosono, S.; Oze, I.; Tanaka, H.; Matsuo, K. Coffee Consumption and the Risk of Colorectal Cancer by Anatomical Subsite in Japan: Results from the HERPACC Studies. Int. J. Cancer. 2017, 141, 298–308. DOI: 10.1002/ijc.30746.
- Sinha, R.; Cross, A. J.; Daniel, C. R.; Graubard, B. I.; Wu, J. W.; Hollenbeck, A. R.; Gunter, M. J.; Park, Y.; Freedman, N. D. Caffeinated and Decaffeinated Coffee and Tea Intakes and Risk of Colorectal Cancer in a Large Prospective Study. Am. J. Clin. Nutr. 2012, 96, 374–381. DOI: 10.3945/ajcn.111.031328.
- Gapstur, S. M.; Anderson, R. L.; Campbell, P. T.; Jacobs, E. J.; Hartman, T. J.; Hildebrand, J. S.; Wang, Y.; McCullough, M. L. Associations of Coffee Drinking and Cancer Mortality in the Cancer Prevention study-II. Cancer Epidemiol. Biomarkers Prev. 2017, 26, 1477–1486. DOI: 10.1158/1055-9965.EPI-17-0353.
- Gan, Y.; Wu, J.; Zhang, S.; Li, L.; Cao, S.; Mkandawire, N.; Ji, K.; Herath, C.; Gao, C.; Xu, H.; et al. Association of Coffee Consumption with Risk of Colorectal Cancer: A Meta-Analysis of Prospective Cohort Studies. Oncotarget. 2017, 8, 18699–18711. DOI: 10.18632/oncotarget.8627.
- Schmit, S. L.; Rennert, H. S.; Rennert, G.; Gruber, S. B. Coffee Consumption and the Risk of Colorectal Cancer. Cancer Epidemiol. Biomarkers Prev. 2016, 25, 634–639. DOI: 10.1158/1055-9965.EPI-15-0924.
- Horisaki, K.; Takahashi, K.; Ito, H.; Matsui, S. A Dose-Response Meta-Analysis of Coffee Consumption and Colorectal Cancer Risk in the Japanese Population: Application of A Cubic-Spline Model. J. Epidemiol. 2018, 28, 503–509. DOI: 10.2188/jea.je20170201.
- Wang A, Wang S, Zhu C, Huang H, Wu L, Wan X, Yang X, Zhang H, Miao R, He L, Sang X, Zhao H. Coffee and Cancer Risk: A Meta-Analysis of Prospective Observational Studies. Sci. Rep. 2016, 6. DOI: 10.1038/srep33711.
- Lee, K.-J.; Inoue, M.; Otani, T.; Iwasaki, M.; Sasazuki, S.; Tsugane, S. Coffee Consumption and Risk of Colorectal Cancer in a Population-Based Prospective Cohort of Japanese Men and Women. Int. J. Cancer. 2007, 121, 1312–1318. DOI: 10.1002/ijc.22778.
- Larson, J. C.; Groessl, E. J.; Snetslaar, L. G.; Allison, M. A.; Tharp, K. M.; Stefanick, M. L. Coffee Consumption and the Incidence of Colorectal Cancer in Women. J. Cancer Epidemiol. 2016, 2016, 1–8. DOI: 10.1155/2016/6918431.
- Guercio, B. J.; Sato, K.; Niedzwiecki, D.; Ye, X.; Saltz, L. B.; Mayer, R. J.; Mowat, R. B.; Whittom, R.; Hantel, A.; Benson, A.; et al. Coffee Intake, Recurrence, and Mortality in Stage III Colon Cancer: Results from CALGB 89803 (Alliance). J. Clin. Oncol.. 2015, 33, 3598–3607. DOI: 10.1200/JCO.2015.61.5062.
- Isshiki, M.; Ohta, H.; Tamura, H. Coffee Reduces SULT1E1 Expression in Human Colon Carcinoma Caco-2 Cells. Biol. Pharm. Bull. 2013, 36, 299–304. DOI: 10.1248/bpb.b12-00902.
- Nkondjock, A.;. Coffee Consumption and the Risk of Cancer: An Overview. Cancer Lett. 2009, 277, 121–125. DOI: 10.1016/j.canlet.2008.08.022.
- Olthof, M. R.; Hollman, P. C. H.; Buijsman, M. N. C. P.; van Amelsvoort, J. M. M.; Katan, M. B. Chlorogenic Acid, Quercetin-3-Rutinoside and Black Tea Phenols are Extensively Metabolized in Humans. J. Nutr. 2003, 133, 1806–1814. DOI: 10.1093/jn/133.8.2622.
- Monteiro, M.; Farah, A.; Perrone, D.; Trugo, L. C.; Donangelo, C. Chlorogenic Acid Compounds from Coffee are Differentially Absorbed and Metabolized in Humans. J. Nutr. 2007, 137, 2196–2201. DOI: 10.1093/jn/137.10.2196.
- Oleaga, C.; Ciudad, C. J.; Noé, V.; Izquierdo-Pulido, M. Coffee Polyphenols Change the Expression of STAT5B and ATF-2 Modifying Cyclin D1 Levels in Cancer Cells. Oxid. Med. Cell. Longev. 2012, 2012, 390385. DOI: 10.1155/2012/390385.
- Nakayama, T.; Funakoshi-Tago, M.; Tamura, H. Coffee Reduces KRAS Expression in Caco-2 Human Colon Carcinoma Cells via Regulation of miRNAs. Oncol. Lett. 2017, 14, 1109–1114. DOI: 10.3892/ol.2017.6227.
- Sharma, S. H.; Rajamanickam, V.; Nagarajan, S. Supplementation of P-Coumaric Acid Exhibits Chemopreventive Effect via Induction of Nrf2 in a Short-Term Preclinical Model of Colon Cancer. Eur. J. Cancer Prev. 2018. DOI: 10.1097/cej.0000000000000496.
- Jaganathan, S. K.; Supriyanto, E.; Mandal, M. Events Associated with Apoptotic Effect of P -Coumaric Acid in HCT-15 Colon Cancer Cells. World J. Gastroenterol. 2013, 19, 7726–7734. DOI: 10.3748/wjg.v19.i43.7726.
- Park, G. H.; Song, H. M.; Jeong, J. B. Kahweol from Coffee Induces Apoptosis by Upregulating Activating Transcription Factor 3 in Human Colorectal Cancer Cells. Biomol. Ther. 2017, 25, 337–343. DOI: 10.4062/biomolther.2016.114.
- Choi, D. W.; Lim, M. S.; Lee, J. W.; Chun, W.; Lee, S. H.; Nam, Y. H.; Park, J. M.; Choi, D. H.; Kang, C. D.; Lee, S. J.; et al. The Cytotoxicity of Kahweol in HT-29 Human Colorectal Cancer Cells Is Mediated by Apoptosis and Suppression of Heat Shock Protein 70 Expression. Biomol. Ther. 2015, 23, 128–133. DOI: 10.4062/biomolther.2014.133.
- Chiang EPI, Tsai SY, Kuo YH, Pai MH, Chiu HL, Rodriguez RL, Tang FY. Caffeic Acid Derivatives Inhibit the Growth of Colon Cancer: Involvement of the PI3-K/Akt and AMPK Signaling Pathways. PLoS One. 2014, 9. DOI: 10.1371/journal.pone.0099631.
- Liang, N.; Kitts, D. D. Amelioration of Oxidative Stress in Caco-2 Cells Treated with Pro-Inflammatory Proteins by Chlorogenic Acid Isomers via Activation of the Nrf2–Keap1–ARE-Signaling Pathway. J. Agric. Food Chem. 2018, 66, 11008–11017. DOI: 10.1021/acs.jafc.8b03983.
- Bedoya-Ramírez, D.; Cilla, A.; Contreras-Calderón, J.; Alegría-Torán, A. Evaluation of the Antioxidant Capacity, Furan Compounds and Cytoprotective/Cytotoxic Effects upon Caco-2 Cells of Commercial Colombian Coffee. Food Chem. 2017, 219, 364–372. DOI: 10.1016/j.foodchem.2016.09.159.
- Shin, H. S.; Satsu, H.; Bae, M. J.; Totsuka, M.; Shimizu, M. Catechol Groups Enable Reactive Oxygen Species Scavenging-Mediated Suppression of PKD-NFkappaB-IL-8 Signaling Pathway by Chlorogenic and Caffeic Acids in Human Intestinal Cells. Nutrients. 2017, 9. DOI: 10.3390/nu9020165.
- Shin HS, Satsu H, Bae MJ, Zhao Z, Ogiwara H, Totsuka M, Shimizu M. Anti-Inflammatory Effect of Chlorogenic Acid on the IL-8 Production in Caco-2 Cells and the Dextran Sulphate Sodium-Induced Colitis Symptoms in C57BL/6 Mice. Food Chem. 2015, 168, 167–175. DOI: 10.1016/j.foodchem.2014.06.100.
- Cicenas, J.; Tamosaitis, L.; Kvederaviciute, K.; Tarvydas, R.; Staniute, G.; Kalyan, K.; Meskinyte-Kausiliene, E.; Stankevicius, V.; Valius, M. KRAS, NRAS and BRAF Mutations in Colorectal Cancer and Melanoma. Med. Oncol. 2017, 34, 26. DOI: 10.1007/s12032-016-0879-9.
- Govaere, O.; Roskams, T. Pathogenesis and Prognosis of Hepatocellular Carcinoma at the Cellular and Molecular Levels. Clin. Liver Dis. 2015, 19, 261–276. DOI: 10.1016/j.cld.2015.01.002.
- Nault, J. C.;. Pathogenesis of Hepatocellular Carcinoma according to Aetiology. Best Pract. Res. Clin. Gastroenterol. 2014, 28, 937–947. DOI: 10.1016/j.bpg.2014.08.006.
- Dhanasekaran, R.; Limaye, A.; Cabrera, R. Hepatocellular Carcinoma: Current Trends in Worldwide Epidemiology, Risk Factors, Diagnosis, and Therapeutics. Hepat. Med. 2012, 4, 19–37. DOI: 10.2147/HMER.S16316.
- Ding, J.; Wang, H. Multiple Interactive Factors in Hepatocarcinogenesis. Cancer Lett. 2014, 346, 17–23. DOI: 10.1016/j.canlet.2013.12.024.
- Mallat, A.; Lotersztajn, S. Cellular Mechanisms of Tissue Fibrosis. 5. Novel Insights into Liver Fibrosis. Am. J. Physiol. Cell Physiol. 2013, 305, C789–99. DOI: 10.1152/ajpcell.00230.2013.
- Higashi, T.; Friedman, S. L.; Hoshida, Y. Hepatic Stellate Cells as Key Target in Liver Fibrosis. Adv. Drug Deliv. Rev. 2017, 121, 27–42. DOI: 10.1016/j.addr.2017.05.007.
- Salomone, F.; Galvano, F.; Volti, G. L. Molecular Bases Underlying the Hepatoprotective Effects of Coffee. Nutrients. 2017, 9. DOI: 10.3390/nu9010085.
- Arab, L.;. Epidemiologic Evidence on Coffee and Cancer. Nutr. Cancer. 2010, 62, 271–283. DOI: 10.1080/01635580903407122.
- Inoue M, Yoshimi I, Sobue T, Tsugane S, Hanaoka T, Ogata J, JPHC Study Group. Influence of Coffee Drinking on Subsequent Risk of Hepatocellular Carcinoma: A Prospective Study in Japan. J. Natl. Cancer Inst. 2005, 97, 293–300. DOI: 10.1093/jnci/dji040.
- Larsson, S. C.; Wolk, A. Coffee Consumption and Risk of Liver Cancer: A Meta-Analysis. Gastroenterology. 2007, 132, 1740–1745. DOI: 10.1053/j.gastro.2007.03.044.
- Bravi, F.; Bosetti, C.; Tavani, A.; Gallus, S.; La Vecchia, C. Coffee Reduces Risk for Hepatocellular Carcinoma: An Updated Meta-Analysis. Clin. Gastroenterol. Hepatol. 2013, 11, 1413–1421.e1. DOI: 10.1016/j.cgh.2013.04.039.
- Bravi, F.; Tavani, A.; Bosetti, C.; Boffetta, P.; La Vecchia, C. Coffee and the Risk of Hepatocellular Carcinoma and Chronic Liver Disease: A Systematic Review and Meta-Analysis of Prospective Studies. Eur. J. Cancer Prev. 2017, 26, 368–377. DOI: 10.1097/CEJ.0000000000000252.
- Kennedy, O. J.; Roderick, P.; Buchanan, R.; Fallowfield, J. A.; Hayes, P. C.; Parkes, J. Coffee, Including Caffeinated and Decaffeinated Coffee, and the Risk of Hepatocellular Carcinoma: A Systematic Review and Dose-Response meta-Analysis. BMJ Open. 2017, 7. DOI: 10.1136/bmjopen-2016-013739.
- Aleksandrova, K.; Bamia, C.; Drogan, D.; Lagiou, P.; Trichopoulou, A.; Jenab, M.; Fedirko, V.; Romieu, I.; Bueno-de-Mesquita, H. B.; Pischon, T.; et al. The Association of Coffee Intake with Liver Cancer Risk Is Mediated by Biomarkers of Inflammation and Hepatocellular Injury: Data from the European Prospective Investigation into Cancer and Nutrition. Am. J. Clin. Nutr. 2015, 102, 1498–1508. DOI: 10.3945/ajcn.115.116095.
- Freedman, N. D.; Everhart, J. E.; Lindsay, K. L.; Ghany, M. G.; Curto, T. M.; Shiffman, M. L.; Lee, W. M.; Lok, A. S.; Di Bisceglie, A. M.; Bonkovsky, H. L.; et al. Coffee Intake Is Associated with Lower Rates of Liver Disease Progression in Chronic Hepatitis C. Hepatology. 2009, 50, 1360–1369. DOI: 10.1002/hep.23162.
- Shim, S. G.; Jun, D. W.; Kim, E. K.; Saeed, W. K.; Lee, K. N.; Lee, H. L.; Lee, O. Y.; Choi, H. S.; Yoon, B. C. Caffeine Attenuates Liver Fibrosis via Defective Adhesion of Hepatic Stellate Cells in Cirrhotic Model. J. Gastroenterol. Hepatol. 2013, 28, 1877–1884. DOI: 10.1111/jgh.12317.
- Elmotasem, H.; Farag, H. K.; Salama, A. A. A. In Vitro and in Vivo Evaluation of an Oral Sustained Release Hepatoprotective Caffeine Loaded W/O Pickering Emulsion Formula – Containing Wheat Germ Oil and Stabilized by Magnesium Oxide Nanoparticles. Int. J. Pharm. 2018, 547, 83–96. DOI: 10.1016/j.ijpharm.2018.05.038.
- Baeza, G.; Amigo-Benavent, M.; Sarriá, B.; Goya, L.; Mateos, R.; Bravo, L. Green Coffee Hydroxycinnamic Acids but Not Caffeine Protect Human HepG2 Cells against Oxidative Stress. Food Res. Int. 2014, 62, 1038–1046. DOI: 10.1016/j.foodres.2014.05.035.
- Seo, H.-Y.; Kim, M.-K.; Lee, S.-H.; Hwang, J. S.; Park, K.-G.; Jang, B. K. Kahweol Ameliorates the Liver Inflammation through the Inhibition of NF-κB and STAT3 Activation in Primary Kupffer Cells and Primary Hepatocytes. Nutrients. 2018, 10, 863. DOI: 10.3390/nu10070863.
- Seo, H.-Y.; Jung, Y.-A.; Lee, S.-H.; Hwang, J. S.; Park, K.-G.; Kim, M.-K.; Jang, B. K. Kahweol Decreases Hepatic Fibrosis by Inhibiting the Expression of Connective Tissue Growth Factor via the Transforming Growth Factor-Beta Signaling Pathway. Oncotarget. 2017, 8, 87086–87094. DOI: 10.18632/oncotarget.19756.
- Yan, Y.; Liu, N.; Hou, N.; Dong, L.; Li, J. Chlorogenic Acid Inhibits Hepatocellular Carcinoma in Vitro and in Vivo. J. Nutr. Biochem. 2017, 46, 68–73. DOI: 10.1016/j.jnutbio.2017.04.007.
- Yan Y, Li J, Han J, Hou N, Song Y, Dong L. Chlorogenic Acid Enhances the Effects of 5-Fluorouracil in Human Hepatocellular Carcinoma Cells through the Inhibition of Extracellular Signal-Regulated Kinases. Anticancer Drugs 2015, 26, 540–546. DOI: 10.1097/CAD.0000000000000218.
- Tunde Olayinka, E.; Solomon Ola, O.; Ore, A.; Adewumi Adeyemo, O. Ameliorative Effect of Caffeic Acid on Capecitabine- Induced Hepatic and Renal Dysfunction: Involvement of the Antioxidant Defence System. Medicines. 2017, 4. DOI: 10.3390/medicines4040078.
- Wilkins, L. R.; Brautigan, D. L.; Wu, H.; Yarmohammadi, H.; Kibicka, E. Cinnamic Acid Derivatives Enhance Efficacy of Transarterial Embolization in a Rat Model of Hepatocellular Carcinoma. Cardiovasc. Interv. Radiol. 2017, 40, 430–437. DOI: 10.1111/pan.12678.Revisiting.
- Brautigan, D. L.; Gielata, M.; Heo, J.; Kubicka, E.; Wilkins, L. R. Selective Toxicity of Caffeic Acid in Hepatocellular Carcinoma Cells. Biochem. Biophys. Res. Commun.. 2018, 505, 612–617. DOI: 10.1016/j.bbrc.2018.09.155.
- Ezhuthupurakkal PB, Ariraman S, Arumugam S, Subramaniyan N, Muthuvel SK, Kumpati P, Rajamani B, Chinnasamy T. Anticancer Potential of ZnO Nanoparticle-Ferulic Acid Conjugate on Huh-7 and HepG2 Cells and Diethyl Nitrosamine Induced Hepatocellular Cancer on Wistar Albino Rat. Nanomedicine Nanotechnology. Biol. Med. 2017, 14, 415–428. DOI: 10.1016/j.nano.2017.11.003.