ABSTRACT
Mung bean starch slurries (75% moisture) were respectively treated by repeated annealing (RANN) at 50°C for eight repetitions (every repetition was treated for 12 h) and continuous annealing (CANN) at the same temperature for 24, 48, 72 and 96 h. After RANN and CANN modifications, variations in the physicochemical properties, structures as well as digestive characteristics of mung bean starch were investigated. Scanning electron microscopy observed the grooves on the surfaces of starch granules became deeper after RANN and CANN modifications, and the cracks in the granule centers were monitored by light microscopy. However, there was no distinct change detected by confocal laser scanning microscopy. RANN and CANN modifications increased the crystallinity and degree of crystalline order, but the crystalline patterns were not changed. Under the same annealing time, RANN samples presented higher crystallinity, gelatinization temperature, enthalpy, peak time, pasting temperature, resistant starch (RS), slowly digestible starch (SDS) and SDS+RS values, but lower solubility, swelling power, peak viscosity, breakdown and rapidly digestible starch (RDS) values than CANN samples. Therefore, RANN could exert conspicuous impacts compared to CANN, which illustrates the annealing impact on starch modification and it could be applied in food processing as an innovative approach.
Introduction
Mung beans are widely planted in many countries because it has been regarded as the inexpensive and nutritious food source. It is an essential ingredient in all kinds of savory and sweet Asian cuisines.[Citation1] Mung beans are widely consumed by koreans in many forms, such as mung bean sprout, soup, flour paste, and pancake.[Citation2] Starch makes up more than half of mung beans in the total weight and amylose content is about 40% of the total starch amount.[Citation3] Amylose content of mung bean starch is larger than those of other legume and cereal starches.[Citation4] High amylose mung bean starch has become the better choice for film-forming biopolymers and starch noodle.[Citation1,Citation5] Mung bean starch has excellent gelling capability and cohesiveness, but it has detrimental storage stability and severe syneresis due to the high amylose content.[Citation4]
As a vitally important physical modification technique, annealing can be applied to alter physicochemical properties and in vitro digestibility of starch on the premise of maintaining granule structure integrity. Starch is heated below the onset gelatinization temperature (To) within a restricted range, generally less than 5-15°C of To, in adequate water contents for a given amount of time that varies between 0.5 h and 240 h.[Citation6–Citation9] Annealing treatment implicates no use of biological and chemical reagents; hence annealed starches are significant ingredients to insert in food without any quantitative restrictions in healthy and safe food compared with chemically or enzymatically modified starch.
At present, there are many literatures describing the impacts of annealing on morphological characteristics, pasting viscosity, thermal properties and in vitro starch digestion of a variety of starches. The seemingly simple treatment could effectively eliminate the restrictions on the nature of starch, including poor paste sheen, low shear, high tendency to retrograde as well as poor adhesion.[Citation10–Citation12] Existing researches showed that some pores, fissures or indentations were detected in starch granules after annealing.[Citation13,Citation14] The treatment increased the thickness of crystalline lamella[Citation15], but the crystalline pattern of barley starch and rice starch remained unchanged.[Citation13,Citation16] Meantime, the molecular weights (Mw) of amylopectin of rice starch reduced but Mw of amylose had no significant change.[Citation16] Annealing could reduce swelling power, increase gelatinization temperature and shrink the gelatinization temperature range (Tc-To) of cassava, barley, wheat, kudzu, and maize starch.[Citation7,Citation13,Citation17–Citation19] Other changes such as in vitro enzyme hydrolysis, gelatinization enthalpy, peak viscosity, setback, and breakdown have not been acquired to form a unified conclusion, particularly for the pasting parameters.[Citation8,Citation20]
The content of rapidly digestible starch (RDS) increased and the levels of resistant starch (RS) and slowly digestible starch (SDS) of rice starch reduced.[Citation16] While the levels of RDS and SDS in annealed pea starch had a noticeable increase, its RS content had a decrease.[Citation21] The physiochemical and functional properties of annealed starch were hardly to have a coincident trend due to diverse starch sources and different annealing temperatures, treatment time and moisture contents.
Annealed starch with desirable properties has been widely applied to food products. The reduced retrogradation and increased thermal stability after annealing treatment are satisfactory properties of frozen and canned food.[Citation6] In addition, annealing-treated starch has been widely utilized as an ingredient in noodle manufacturing since its texture was improved (chewiness, tensile strength, and adhesiveness) due to higher shear and heat stability and lower amylose leaching and swelling power.[Citation22] Finally, annealing could elevate the RS content, so annealed starch is also used to elevate the dietary fiber content or applied as a fat mimetic.[Citation6]
As of now, it has been confirmed that the repeated annealing treatment is a more effective modification compared with the continuous one. The repeated annealed starches possessed the higher relative crystallinity, thermal stability, pasting temperature and SDS content of the potato starch compared with CANN ones.[Citation23] By contrast, CANN starches of the red adzuki bean had a higher relative crystallinity than RANN ones.[Citation24] The differences in these consequences mayresult from the difference in the structure of the starch, which has a great relationship with these factors such as amylose content, particle size, and starch sources. Therefore, the study aimed to investigate the influence on structural, physicochemical and digestibility properties of the mung bean starch after repeated versus continuous annealing and the difference between RANN and CANN. This could provide a creative insight for the physical denaturation of starch in food and non-food applications.
Materials and methods
Materials and reagents
Mung bean seeds without any impurities were owned from Shandong Jinnuo Seed Co., Ltd (Shandong, China). The chemical components of mung bean starch on a dry basis were: 98.55% of starch (starch purity), 36.21% of amylose, 0.25% of protein, 0.12% of lipid and 0.18% of ash, respectively. The analytical grade of the chemical reagents was higher than 99.7% in this experiment.
Isolation and preparation of RANN- and CANN-treated samples
Native mung bean starch was isolated from mung bean seeds by a previously used method.[Citation25] The continuous and repeated annealed starch samples were prepared using a similar method of Xu et al. (2018a)[Citation23] with slight modifications. Fifty grams of native mung bean starch (dry basis) and 150 mL deionized water (containing 0.02% sodium azide) were added into a 250 mL blue glass container. Repeated annealing treatments were carried out as the following procedure: one cycle was that the container was treated in a dry oven at 50°C for 12 h, then removed from the oven and left it at the temperature of 25 ± 2°C for 0.5 h. The samples experiencing the first cycle were treated for a total of eight repetitions. The obtained samples were coded as RANN-1, 2, 4, 6, and 8.
The procedure of continuous annealing treatment was as followed: the identical starch-water suspensions were treated at the same annealing temperature for continuous 24, 48, 72 and 96 h. The obtained samples were coded as CANN-24, 48, 72 and 96, respectively. After the treatments completed, mung bean samples were centrifuged and milled. It should be noted that the impacts of annealing on starch properties would be more prominent if the annealing temperature was set (close) to but below To (about 5-15°C below To)[Citation9], so the 50°C was chosen for annealing temperature of mung bean starch.
Structure analysis
Scanning electron microscopy (SEM)
Morphological characteristics of mung bean starch samples were observed by scanning electron microscope (S-3400N, Hitachi Int., Japan) at an accelerating voltage of 5.0 kV.
Light microscopy
The samples were suspended in a mixed solution of water-glycerol (1:1, V:V) and were examined under optical and polarized light using a DMBA 400 microscope (Motic China Group Co., Ltd., Guangzhou, China) with a 400x objective.
Confocal laser scanning microscopy (CLSM)
The CLSM of mung bean starch samples was conducted in a procedure described by Gong et al. (2017).[Citation12] The images were obtained by using CLSM (Nikon Co., Ltd., Tokyo Japan) equipped with 100 × plan apo/1.4011 oil UV.
X-ray diffraction (XRD)
XRD patterns and crystallinity of samples were demonstrated by an X-ray diffractometer (Rigaku D/MAX 2500 V, Rigaku Corporation, Japan) using CuKα radiation. A starch sample was scanned from 4° to 60°. The step time and step size were 0.2 s and 0.02 in the process. The samples were measured at a ray wavelength of 0.154178 nm. The relative crystallinity was quantitatively calculated from the ratio of diffraction peak area to total diffraction peak area using MDI-Jade 5.0 software (Material Date, Inc.Livermore, California, USA).
Fourier transform-infrared spectroscopy (FT-IR)
The mixture of anhydrous potassium bromide and starch in weight of 100:1 were compressed to form a pellet. The FT-IR spectra of samples were measured by a Vertex 70 (Bruker, Germany). The spectral resolution was 4 cm−1 over 64 scans. The spectra were deconvoluted from 4000 cm−1 to 400 cm−1. The prepared tablet of anhydrous potassium bromide was served as background scanning.
Viscosity analysis
The pasting viscosity of a sample was measured by a Rapid Viscosity Analyzer (RVA, TechMaster, Perten, Sweden). Samples (3.0 g, 14% moisture basis) and distilled water was added into an RVA canister to reach a total weight of 28.0 g. This procedure was carried out as previously described by Zhao et al. (2018).[Citation11]
Solubility and swelling power
Solubility and swelling power were measured based on the procedure described by Gong et al. (2017).[Citation12]
Thermal properties
A sample (3.0 mg) and distilled water (9 µL) were added into an aluminum pan, respectively. The prepared aluminum pans were sealed and placed in the refrigerator (4°C) for 12 h to reach equilibrium. The samples were heated in a differential scanning calorimeter (DSC, Q2000, TA Instruments, USA) against an empty reference pan from 20°C to 120°C at 10°C/min.
In vitro starch digestion
Starch samples were digested following the method described by Englyst, Kingman and Cumming (1992).[Citation26]
Statistical analysis
Values were expressed as the mean value ± standard deviation. The significance defined (p < .05) was analyzed by analysis of variance (ANOVA) with Minitab version 16.2.3 statistical software for Windows.
Results and discussion
Microscopic morphology
Mung bean starch granules presented round or kidney shapes with the diameter between 5 and 30 µm ( A1). Native starch granules were observed having smooth surfaces, and shallow grooves appeared on the surfaces of most of the granules ( A1). There were no apparent changes on the surfaces of RANN and CANN treated starch granules, except that the grooves on the granule surfaces became deeper after RANN and CANN treatments ( B1-J1). Likewise, the integrity of potato starch[Citation23] and finger millet starch[Citation26] were[Citation27] not altered appreciably. However, surface pores were observed in granules of high-amylose rice starch under treatment of 55°C for 16 h[Citation28], and the evidence of some cracks appeared along the grooves and indents on the pea starch granule surfaces treated with annealing at 45°C for 24 h.[Citation21] Moreover, starch agglomerates or aggregates were viewed in annealed pea and rice starch.[Citation21,Citation28]
Figure 1. Scanning electron micrographs (SEM) (×1000) (1), light micrographs (×400) (2), polarized light micrographs (PLM) (×400) (3) and confocal laser scanning micrographs (CLSM) (512 × 512 pixel resolution) (4) of native, RANN and CANN starch samples. A, Native; B, RANN-1; C, RANN-2; D, CANN-24; E, RANN-4; F, CANN-48; G, RANN-6; H, CANN-72; I, RANN-8; J, CANN-96
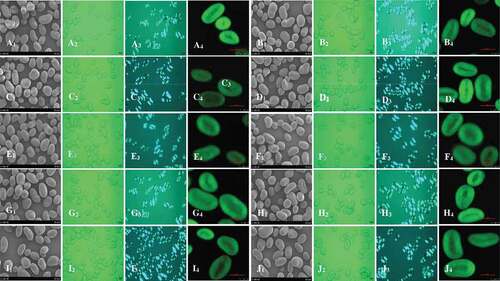
The micrographs observed via light microscopy were presented in (A2-J2). From the micrographs, cracks at the granule center were detected after RANN and CANN treatments, but this phenomenon was not found at the granule periphery. Perhaps starch chains at the granule center were loosely arranged than those at the periphery, and were therefore more liable to reorientation and rearrangement during annealing, leading to an increase in crystallinity. Native mung bean starch granules ( A3) showed characteristic birefringence patterns (“Maltese cross”). The intensity of birefringence depended on the relative crystallinity, overall size of granules, and microcrystalline orientation.[Citation29,Citation30] The intensity of “Maltese cross” of RANN- and CANN-treated mung bean starch had no detectable changes ( B3-J3). Similar phenomenon was also found in the potato starch and red adzuki bean starch after RANN and CANN.[Citation23,Citation24]
The appearance of internal cavities (hilum) and growth rings in mung bean starch granules could be clearly detected for native, RANN and CANN annealed starch by CLSM after fluorescence labeling with 8-amino-1,3,6-pyrenetrisulfonic acid (APTS). Native and annealed starch granules were not stained uniformly after fluorescence labeling with 8-amino-1, 3, 6-pyrenetrisulfonic acid (APTS) ( A4-J4). This was because amylopectin would be less heavily labeled with APTS than amylose, due to the fact that amylopectin possesses lower molar ratio of reducing ends per glucose residue than amylose.[Citation30] The CLSM images showed that the degrees of dying and area sizes of starch granules were not changed visually with the prolonged annealing time and increased number of repetitions ( B4-J4).
Recrystallization characteristics
Native mung bean starch exhibited a typical C-type pattern characterized by the peaks at 15°, 17° and 23° (), as observed in the previous study.[Citation5] The overall crystalline patterns of CANN and RANN treated mung bean starch remained essentially unchanged (). Similarly, the crystalline patterns of B-type potato starch and C-type red adzuki bean starch were not changed after repeated and continuous annealing.[Citation23,Citation24]
As the prolonged annealing time and increased number of repetitions, crystallinity of continuously and repeatedly annealed starch increased to 23.21% and 23.76%, respectively (). An increase in relative crystallinity after annealing treatment resulted from the amylopectin content, the orientation of the starch crystallites, crystallite perfection, enhanced order of the V-amylose–lipid complex or formation of amylose crystallites.[Citation6,Citation9,Citation13] As the number of repetitions and annealing time increased, the interaction force between starch chains may be enhanced gradually and the crystallites may become more perfect and ordered, so that the crystallinity increased progressively. This change trend was consistent with our previous research in potato starch and red adzuki bean starch.[Citation23,Citation24]
Table 1. X-ray diffraction and infrared spectroscopy ratios with 1043 cm−1/1022 cm−1 of native, RANN, and CANN starch samples
What is noteworthy is that RANN samples presented higher crystallinity than CANN ones under the same annealing time (), indicating more satisfactory crystal structures and ordered molecular orientation of RANN starch samples than CANN samples. It could be guessed that the intermittent phase of placing the sample at room temperature for 30 min could effectively strengthen the interaction between amylose-amylose and amylose-amylopectin.[Citation23] The intermittent phase may be more conducive to the motivation of water molecules, which led to an increase in the degree of interaction between chains to promote reorientation and rearrangement of starch molecules. The newly generated starch structure might be maintained during the intermediate phase between the repetitions. Therefore, the processing of the next RANN would be beneficial to this new structure, and it might benefit the consolidation of the already changed structure. However, CANN-treated red adzuki bean starch samples possessed higher crystallinity than the RANN ones under the same treatment time.[Citation24] The discordance in crystallinity between mung bean starch and red adzuki bean starch may mainly depend on the type of starch.
FT-IR spectroscopy
The FT-IR spectra and the ratio of 1043 cm−1/1022 cm−1 of mung bean starch samples were summarized in and . The intensity ratio of 1047 cm−1/1022 cm−1 was evaluated the crystallinity and the degree of crystalline order.[Citation31] So the RANN- and CANN-treated samples with higher ratios of 1043 cm−1/1022 cm−1 possessed higher crystallinity than native starch. It was attributed that the temperature at 25 ± 2°C during the cooling process was more beneficial to increase the degree of interaction between starch molecular chains, the starch granules treated by RANN had more ordered and stable granular structures than those treated by CANN.
No new absorption peaks were appeared or vanished after RANN and CANN treatments, indicating that no chemical group was newly introduced or destructed during RANN and CANN treatments. The absorbance ratio of 1043 cm−1/1022 cm−1 was elevated gradually with the increased annealing time and number of cycles (), revealing that both RANN and CANN could increase the degree of crystalline order and crystallinity of mung bean starch.
Solubility and swelling power
The swelling power and solubility of annealed starch samples displayed a downward trend compared to native starch (). A large number of literatures have also shown a consistent conclusion, demonstrating decreased swelling power and solubility compared to native starch.[Citation6,Citation7,Citation9,Citation13] The observed phenomenon in solubility and swelling power was in connection with increased interactions between starch chains or molecular organizations, which reduced the hydration degree in the amorphous regions and restrained the swelling and leaching of starch molecules.[Citation6,Citation23,Citation24]
Table 2. Solubility and swelling power of native, RANN, and CANN starch samples
Meanwhile, the swelling power and starch solubility decreased gradually with annealing time and number of repetitions increasing. The prolonged annealing time and increased number of repetitions caused the degree of interaction between starch chains to further extend, and then brought about further reduction in the diffusion level of amylose and amylopectin molecules.
Solubility and swelling power of RANN treated starch samples were almost remarkably lower than the corresponding part of CANN ones under the same treatment time. The rearrangement or reorientation of starch molecules in the cooling process during RANN was responsible for higher crystallinity compared to CANN so that the diffusion capacity of amylose and swelling extents of amylopectin molecules of RANN starch were weaker than that of CANN ones.
Pasting characteristics analysis
The effect of RANN and CANN treatments on the pasting properties of mung bean starch conducted by RVA was summarized in and . The peak viscosity of RANN- and CANN-treated samples had a general downward trend as well as breakdown values, whereas the trough viscosity, final viscosity, setback values, peak time, and pasting temperature of the samples had an upward trend compared to the native starch. Peak viscosity and breakdown values of the CANN- and RANN-treated mung bean starch showed a progressive decrease with the increasing processing time and number of cycles (). The peak viscosity was the maximum viscosity of swollen starch granules during heating, and the change trend of peak viscosity was in accord with the results of “swelling power” (). Breakdown refers to the fragility of starch granules and stability of the starch paste during shearing at high temperatures.[Citation32] The increased heat stability after RANN and CANN treatments was relevant to more ordered and compact crystal structures after annealing.
Table 3. Pasting parameters of native, RANN, and CANN starch samples
RANN- and CANN-treated samples showed higher setback viscosity than that of native starch, which indicated that the modified starches were less stable than the native starch during the process of cooling. This setback parameter of CANN starch increased in the first 72 h of annealing and then decreased, and the values of RANN samples had a tendency of ascending in the first four cycles and then descending in succession. Setback values were influenced by the contents of spilled amylose and long-chain amylopectin, structure of starch granules, and contents of non-gelatinized starch.[Citation30]
Notably, RANN samples presented significantly lower peak viscosity and breakdown viscosity than that of CANN ones under the same annealing time, and the final viscosity and setback values of RANN samples were greater than the CANN ones under the treatments of four cycles and 48 h, and then were less than the CANN ones afterward. These differences largely depended on the strengthened granular structures of starch and reinforced intra-molecular bonding compared to CANN samples.
Analysis of thermal transition properties
Gelatinization properties of mung bean starch samples were measured by DSC ( and ). The values of To, Tp, Tc, and ΔH increased with the increased annealing time and number of annealing times (). The transition temperatures increased resulted from the enhanced order of starch molecules. The increased gelatinization enthalpy indicated that the structure of the double helices or crystals was dramatically strengthened after annealing treatment.
Table 4. Thermal properties of native, RANN, and CANN starch samples
Moreover, RANN treatment was more beneficial to increase the values of gelatinization temperatures and enthalpy than CANN treatment. This may be caused by redistribution of water molecules and rearrangement of starch molecules by RANN modification, resulting in better crystal structures and higher gelatinization transition temperatures and ΔH. The analytical results were in general agreement with the pasting temperature of RVA ().
In vitro digestibility analysis
The digestive properties of native, RANN and CANN starches are displayed in . Both RANN starch and CANN starch possessed higher levels of RS, SDS as well as RS+SDS, but a lower level of RDS compared to native starch. The increased SDS and RS contents were ascribed to the interaction among the amylose and amylopectin molecules and crystallite perfection after annealing, which could cause the enzymatic susceptibility of mung bean starch decrease. Annealing also increased the RS and SDS contents and decreased the RDS content in potato starch and red adzuki bean starch after RANN and CANN treatments[Citation23,Citation24], while annealing decreased the RS level and increased the RDS levels in pea starch.[Citation21] The discrepant results may be relevant to the annealing conditions and the kind of starches.
Furthermore, mung bean starch samples after RANN treatment showed lower RDS content than the samples after CANN treatment, while the contents of RS, SDS, and RS+SDS in RANN samples presented higher values than CANN samples. It indicated that RANN was more beneficial to decrease in the enzymatic susceptibility of mung bean starch compared to CANN. Likewise, the rearrangement of starch molecules during RANN treatment was more conducive to form a steadier and more compact crystal structure than CANN treatment, which was inconsistent with the XRD results as mentioned above.
Conclusion
The study was designed to reveal the impact of repeated and continuous annealing modifications on microscopic morphology, digestive properties and physicochemical changes of mung bean starch. RANN treatment was more conducive to increase the relative crystallinity, degree of crystalline order and gelatinization enthalpy, and decrease in the enzymatic susceptibility of mung bean starch compared to CANN treatment. The rearrangement of RANN-treated starch molecules during a cooling process might strengthen and stabilize the starch structure to a greater extent. RANN treatment could produce excellent properties for mung bean starch, which suggested a desirable modification method when compared to CANN, so that it could tailor the functional performance of starch for specific industrial applications and then provide a prospective reference for further study of physical modification.
Acknowledgments
Authors thank the Fundamental Research Funds for the Natural Science Foundation of China (31301557) and the research and innovation team construction plan of Henan University of Animal Husbandry and Economy (2018KYTD17).
Additional information
Funding
References
- Rompothi, O.; Pradipasena, P.; Tananuwong, K.; Somwangthanaroj, A.; Janjarasskul, T. Development of Non-Water Soluble, Ductile Mung Bean Starch Based Edible Film with Oxygen Barrier and Heat Sealability. Carbohydr. Polym. 2017, 157, 748–756. DOI: 10.1016/j.carbpol.2016.09.007.
- Junhee, N.; Malshick, S. Textural Properties of Mung Bean Starch Gels Prepared from Whole Seeds. Food Sci. Biotechnol. 2016, 25, 729–734. DOI: 10.1007/s10068-016-0126-3.
- Li, S. L.; Ward, R.; Gao, Q. Y. Effect of Heat–Moisture Treatment on the Formation and Physicochemical Properties of Resistant Starch from Mung Bean (Phaseolus Radiatus) Starch. Food Hydrocoll. 2011, 25, 1702–1709. DOI: 10.1016/j.foodhyd.2011.03.009.
- Kim, S.; Lee, B.; Baik, M.; Joo, M.-H.; Yoo, S.-H. Chemical Structure and Physical Properties of Mung Bean Starches Isolated from 5 Domestic Cultivars. J. Food Sci. 2007, 72, 471–477. DOI: 10.1111/jfds.2007.72.issue-9.
- Li, W. H.; Guo, H. M.; Wang, P.; Tian, X.; Zhang, W.; Saleh, A. S. M.; Zheng, J.; Ouyang, S.; Luo, Q.; Zhang, G. Physicochemical Characteristics of High Pressure Gelatinized Mung Bean Starch during Recrystallization. Carbohydr. Poly. 2015, 131, 432–438. DOI: 10.1016/j.carbpol.2015.05.076.
- Jayakody, L.; Hoover, R. Effect of Annealing on the Molecular Structure and Physicochemical Properties of Starches From Different Botanical Origins-A Review. Carbohydr. Polym. 2008, 74, 691–703. DOI: 10.1016/j.carbpol.2008.04.032.
- Gomes, A. M. M.; Da Silva, C. E. M.; Ricardo, N. M. P. S. Effects of Annealing on the Physicochemical Properties of Fermented Cassava Starch (Polvilho Azedo). Carbohydr. Polym. 2005, 60, 1–6. DOI: 10.1016/j.carbpol.2004.11.016.
- Zavareze, E. D. R.; Dias, A. R. G. Impact of Heat-Moisture Treatment and Annealing in Starches: A Review. Carbohydr. Polym. 2011, 83, 317–328. DOI: 10.1016/j.carbpol.2010.08.064.
- Tester, R. F.; Debon, S. J. J. Annealing of Starch-A Review. Int. J. Biol. Macromol. 2000, 27, 1–12. DOI: 10.1016/S0141-8130(99)00121-X.
- BeMiller, J. N.;. Starch Modification: Challenges and Prospects. Starch-Stärke. 1997, 49, 127–131. DOI: 10.1002/(ISSN)1521-379X.
- Zhao, K.; Li, B.; Xu, M. J.; Jing, L.; Gou, M.; Yu, Z.; Zheng, J.; Li, W. Microwave Pretreated Esterification Improved the Substitution Degree, Structural and Physicochemical Properties of Potato Starch Esters. LWT-Food Sci. Technol. 2018, 90, 116–123. DOI: 10.1016/j.lwt.2017.12.021.
- Gong, B.; Xu, M. J.; Li, B.; Wu, H.; Liu, Y.; Zhang, G.; Ouyang, S.; Li, W. Repeated Heat-Moisture Treatment Exhibits Superiorities in Modification of Structural, Physicochemical and Digestibility Properties of Red Adzuki Bean Starch Compared to Continuous Heat-Moisture Way. Food Res. Int. 2017, 102, 776–784. DOI: 10.1016/j.foodres.2017.09.078.
- Waduge, R. N.; Hoover, R.; Vasanthan, T.; Gao, J.; Li, J. Effect of Annealing on the Structure and Physicochemical Properties of Barley Starches of Varying Amylose Content. Food Res. Int. 2006, 39, 59–77. DOI: 10.1016/j.foodres.2005.05.008.
- Kiseleva, V. I.; Krivandin, A. V.; Fornal, J.; Błaszczak, W.; Jeliński, T.; Yuryev, V. P. Annealing of Normal and Mutant Wheat Starches. LM, SEM, DSC and SAXS Studies. Carbohydr. Res. 2005, 340, 75–83. DOI: 10.1016/j.carres.2004.10.012.
- Kiseleva, V. I.; Genkina, N. K.; Teste, R.; Wasserman, L. A.; Popov, A. A.; Yuryev, V. P. Annealing of Normal, Low and High Amylose Starches Extracted from Barley Cultivars Grown under Different Environmental Conditions. Carbohydr. Polym. 2004, 56, 157–168. DOI: 10.1016/j.carbpol.2004.01.006.
- Bian, L.; Chuang, H. J. Molecular Structure and Physicochemical Properties of Starch Isolated from Hydrothermally Treated Brown Rice Flour. Food Hydrocoll. 2016, 60, 345–352. DOI: 10.1016/j.foodhyd.2016.04.008.
- Lan, H.; Hoover, R.; Jayakody, L.; Liu, Q.; Donner, E.; Baga, M.; Asare, E. K.; Hucl, P.; Chibbar, R. N. Impact of Annealing on the Molecular Structure and Physicochemical Properties of Normal, Waxy and High Amylose Bread Wheat Starches. Food Chem. 2008, 111, 663–675. DOI: 10.1016/j.foodchem.2008.04.055.
- Zhang, B.; Wu, C. S.; Li, H. Y.; Hu, X.; Jin, Z.; Tian, Y.; Xu, X. Long-Term Annealing of C-Type Kudzu Starch: Effect on Crystalline Type and Other Physicochemical Properties. Starch-Stärke. 2015, 67, 577–584. DOI: 10.1002/star.v67.7-8.
- Wang, S. J.; Wang, J. R.; Yu, J. L.; Wang, S. A Comparative Study of Annealing of Waxy, Normal and High-Amylose Maize Starches: The Role of Amylose Molecules. Food Chem. 2014, 164, 332–338. DOI: 10.1016/j.foodchem.2014.05.055.
- Wang, S. J.; Wang, J. R.; Wang, S. K.; Wang, S. Annealing Improves Paste Viscosity and Stability of Starch. Food Hydrocoll. 2017, 62, 203–211. DOI: 10.1016/j.foodhyd.2016.08.006.
- Wang, S. J.; Jin, F. M.; Yu, J. G. Pea Starch Annealing: New Insights. Food Bioprocess. Tech. 2013, 2013(6), 3564–3575. DOI: 10.1007/s11947-012-1010-7.
- Hormdok, R.; Noomhorm, A. Hydrothermal Treatments of Rice Starch for Improvement of Rice Noodle Quality. Food Bioprocess. Tech. 2007, 40, 1723–1731.
- Xu, M. J.; Saleh, A. S. M.; Gong, B.; Li, B.; Jing, L.; Gou, M.; Jiang, H.; Li, W. The Effect of Repeated Versus, Continuous Annealing on Structural, Physicochemical, and Digestive Properties of Potato Starch. Food Res. Int. 2018a, 111, 324–333. DOI: 10.1016/j.foodres.2018.05.052.
- Xu, M. J.; Saleh, A. S. M.; Liu, Y.; Jing, L. Z.; Zhao, K.; Wu, H.; Zhang, G. Q.; Ouyang, S. H.; Li, W. H. The Changes in Structural, Physicochemical, and Digestive Properties Of Red Adzuki Bean Starch after Repeated and Continuous Annealing Treatments. Starch-stärke. 2018b, 70. DOI: 10.1002/star.201700322.
- Jiang, B.; Li, W. H.; Hu, X. S.; Wu, J.; Shen, Q. Rheology of Mung Bean Starch Treated by High Hydrostatic Pressure. Int. J. Food Prop. 2015, 18, 81–92. DOI: 10.1080/10942912.2013.819363.
- Englyst, H. N.; Kingman, S. M.; Cummings, J. H. Classification and Measurement of Nutritionally Important Starch Fractions. Eur. J. Clin. Nutr. 1992, 46, S33–S50.
- Adebowale, K. O.; Afolabi, T. A.; Olu-Owolabi, B. I. Hydrothermal Treatments of Finger Millet (Eleusine Coracana) Starch. Food Hydrocoll. 2005, 19, 974–983. DOI: 10.1016/j.foodhyd.2004.12.007.
- Dias, A. R. G.; Zavareze, E. D. R.; Spier, F.; de Castro, L. A. S.; Gutkoski, L. C. Effects of Annealing on the Physicochemical Properties and Enzymatic Susceptibility of Rice Starches with Different Amylose Contents. Food Chem. 2010, 123, 711–719. DOI: 10.1016/j.foodchem.2010.05.040.
- Zhang, B. J.; Li, X. X.; Liu, J.; Xie, F.; Chen, L. Supramolecular Structure of A- and B-Type Granules of Wheat Starch. Food Hydrocoll. 2013, 31, 68–73. DOI: 10.1016/j.foodhyd.2012.10.006.
- Li, W. H.; Xiao, X. L.; Zhang, W. H.; Zheng, J.; Luo, Q.; Ouyang, S.; Zhang, G. Compositional, Morphological, Structural and Physicochemical Properties of Starches from Seven Naked Barley Cultivars Grown in China. Food Res. Int. 2014, 58, 7–14. DOI: 10.1016/j.foodres.2014.01.053.
- Hu, X. P.; Huang, T. T.; Mei, J. Q.; Jin, Z.-Y.; Xu, X.-M.; Chen, H.-Q. Effects of Continuous and Intermittent Retrogradation Treatments on in Vitro Digestibility and Structural Properties of Waxy Wheat Starch. Food Chem. 2015, 174, 31–36. DOI: 10.1016/j.foodchem.2014.11.026.
- Li, W. H.; Shan, Y. L.; Xiao, X. L.; Luo, Q.; Zheng, J.; Ouyang, S.; Zhang, G. Physicochemical Properties of A- and B-Starch Granules Isolated from Hard Red and Soft Red Winter Wheat. J. Agr. Food Chem. 2013, 61, 6477–6484. DOI: 10.1021/jf400943h.