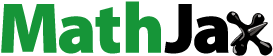
ABSTRACT
Paralytic shellfish poisoning (PSP) toxin is widely contained in seafood and its product. It brings great harm to human health when people eat it. During the past two decades, shellfish toxin quantitative analysis methods develop fast, such as mouse bioassay (MBA), chemical analysis method, immunoassay and cell-based assay (CBA). However, there are some disadvantages in these analytical methods such as time consuming, high cost. Therefore, a more suitable method is in demand. In this study, a novel method using high-frequency surface acoustic wave resonator (SAWR)-based sensor combined with living cells was developed for shellfish toxin continuous monitoring. The mouse tongue isolated taste bud (MTITB) cells cell lines were used as the sensing elements to establish the sensor device for PSP toxin carbamoyl toxin (CT) quantitative monitoring. The sensor device is in serial with SAWR. SAWR output frequency was recorded for detecting characterization. HEK293 cell lines were cultured on electrode and utilized as negative control. The results showed that MTITB cell-based sensor had selective responses to CT. This method is promising in PSP toxins rapid quantitative determination.
Introduction
Paralytic shellfish poisoning (PSP) toxin is a kind of dangerous food toxin. It can cause a series of health problems, such as muscle paralysis of the peripheral nerve by blocking the sodium channels in the cell, and leads to paralysis to the nervous system.[Citation1] PSP threaten public health and marine product safety greatly due to its wide distribution. News pertaining to that people is poisoned by shellfish toxin is frequently reported.[Citation2]
PSP is one of the organic highly toxic toxins, and its toxicity is the same with tetrodotoxin. PSP is stable under acid or high temperature environment. Current PSP quantitative analytical methods include animal testing, instrument analysis, immunoassay, and cell-based sensing techniques.[Citation3–Citation5] Animal testing method is the officially recommended method for PSP toxin detection in Europe, Japan, and other countries. Animal testing method is easy to follow, but frequently protested by animal protecting people and organizations. Instrument analysis method is commonly used under laboratory environment, presents high accuracy and low detecting limit. However, instrument analysis method needs standard reference substances and experienced operators. In addition, this method requires extensively expensive instrumentations. Immunoassay method is fast and accurate. But the cost of this technique is expensive, limiting its field applications in PSP quantitative detection. However, the current methods usually have bulky size, expensive prices, and fuzzy operation procedures. For marine toxin detection, small-sized and cheap instruments are greatly demanded. Furthermore, the operating procedure intends to be simplified so that the operators can easily handle it. These disadvantages greatly limit the development of novel methods or equipment that can be conveniently used for rapid field detection for toxins.
Cell-based sensing technique has attracted much attention of researchers around the world in the past two decades.[Citation6–Citation8] It utilizes living cells as sensing elements based on the interactions between living cells and the detecting chemicals. The chemicals present biochemical effects on the cells cultured on the detecting electrode. Finally, the relationship between chemical information and sensor responses is built for chemical quantitative detection. Cell-based sensors have some special properties, such as rapid response, good selectivity, and high sensitivity. This method has been successfully applied in abundant research fields, such as drug screening, medicine evaluation, and toxin detection.
Some studies on chemical detection using surface acoustic wave (SAW) devices have been proposed.[Citation9] SAW technique is sensitive to weak signals, and it offers a reliable, effective and simple way to obtain weak signal measurement data.[Citation10–Citation12] SAW technique presents microbial count measurement abilities for multiple samples. Yao et al (1995) proposed SAW resonator system and a pair of conductive electrodes in the feedback circuit with a RF amplifier.[Citation10] Surface acoustic wave resonator (SAWR) impedance measurements have also been widely applied in many research fields.[Citation13–Citation16]
In this paper, a high-frequency SAWR-based sensor combined with living cells was proposed for PSP toxin continuous monitoring. MTITB cell lines were used as the sensing elements to establish the sensor device for CT quantitative monitoring. Sensor responses to different CT solutions were recorded. CT quantitative determination model was built based on non-linear fitting regression. HEK293 cell lines were cultured on electrode and utilized for negative control experiments.
Materials and methods
Materials
PBS was purchased from Haotian Pharmacy (Hanzhou, China). Poly-l-ornithine, RPMI 1640, fetal calf serum and EDTA-trypsin were purchased from GIBCO (Grand Island, USA). Anhydrous alcohol was purchased from Kelong Co., Ltd (Chengdu, China). OA, Dimethyl sulfoxide (DMSO) were purchased from Sigma-Aldrich Chemical Co. (Milwaukee, WI, USA). CT was purchased from Amresco, USA. Screen printed carbon electrodes (Herui Bio, Shanghai) and electrochemical analyzer CHI-660C (CHI instruments, Inc., USA) were used in cell-based sensor assay. All chemicals were analytically pure grade. All the chemical reagents are tested by golden standard method to identify the accurate concentration of the measured solutions.
Cell culture
Esophageal carcinoma (MTITB) cell lines and human embryonic kidney 293 cell lines (HEK293) were purchased from the American Type Culture Collection and cultivated in 25cm2-volumed plastic flasks (Jinuo Co., Ltd, China) with 1640 containing 10% FCS and 1% penicillin and streptomycin in an atmosphere containing a humidified 5% CO2 at 37°C. HEK 293 cells are popular for their ease of growth and transfection, making them a common cell culture in cancer research. In addition, high transfection efficiency of HEK293 cells produces exogenous proteins or viruses for pharmaceutical and biomedical research purposes. HEK-293 cells are useful for many transfection experiments, particularly the propagation of adenoviral-based and retroviral-based vectors. The cell culture medium is renewed every second or third day. The cells are cultured on a 60mm diameter tissue culture dish in a humidified incubator at 37℃ with 5% CO2. 10μL of 106/mL cell suspension is seeded on the surface of CSPE coated with 100μg/mL poly-l-ornithine and 10g/mL laminin prior to cell-seeding procedure
SAWR detecting system
ST-X cut quartz substrate with operating frequency of 433MHz used in two-port SAWR fabrication. SAWR structure contains 30 pairs of electrodes for usage of input and output. 150 electrodes are involved in each reflective array. The electrode is made by high purity aluminum sheet with thickness of 2000Å. The precise photolithographic technique with negative masks has been applied to form SAWR pattern. A metal vacuum shell is utilized to make the SAWR device vacuum, which is shown in ). SAWR is utilized to perform basic working frequency for the designed sensor. Under vacuum condition, the device performs a more stable working frequency for the proposed sensor. Furthermore, vacuum packaging can also prevent the device from corrosion and perturbation, and prolong the service life of the proposed sensor. Screen-printed carbon electrode is purchased from Herui Bio (Shanghai) (see )). The potential values are referred to the screen-printed silver pseudo-reference electrode. This kind of electrode is widely used in biochemical analysis due to its convenient design, good stability, and low cost.
Model and principle and testing system
The cell-cultured screen-printed electrode is fixed in a flow-cell, and connected with the electrochemical working station. SAWR was used as frequency signal provider to form a positive feed oscillating circuit with other discrete components. The equivalent circuit of screen-printed electrode in serial with SAWR is displayed in ). C0 is the shunt static capacitance, and Ls, Cs and Rs are dynamic inductance, dynamic capacitance, and dynamic resistance, respectively. The cell-cultured is in serial with SAWR device. Ce and Re are equivalent capacity and resistor of the screen-printed electrode, respectively. The living cells in culture media modify Ce and Re of the electrode, altering the SAWR frequency finally. SAWR resonance frequency F is
where is the SAW basic working frequency.
is a phase parameter of the amplifier circuit.
is surface conductance of the screen-printed electrode. Interelectrode capacitance
, among which
is dielectric constant and
is the parasitic capacitance of connecting wires. SAWR is vacuum-packaged and its equivalent parameter remains stable during measurement. Resonance frequency of this design is determined by
and
. Thus the substances on electrode are more suitable. The viable cells on electrode induce changes in
and
. Changes in frequency
can be achieved.
Measurement system is displayed in ). A positive feedback oscillation circuit is fabricated to generate oscillator signals. The cell-cultured electrode is serial with SAWR via a compatible interface. The frequency signal is calculated by an electrical counter. The sensing system is fixed in a shielded box to avoid the electromagnetic interference.
Results and discussion
Cell culture results
The cells were used as sensing elements in this sensor. It is important to seed the cells on working electrode properly and keep them alive. Poly-l-ornithine and lamimin were used to improve the biocompatibility of the carbon material on the electrode. After being cultured for 2 days, the cells were deployed on electrode uniformly (see ). Experiments were conducted to study the viability of the cells. The cells off were washed off, and trypan blue dye (0.04%) was used to evaluate cell viability. The proportion of living MTITB cells reached about 90%. In all areas of the working electrode, the total cell number was over 2 × 104. In the same way, the number of living HEK cells in working electrode area was over 2 × 103 according to the SEM characterization results. Results demonstrated that the cell culture was successful. Differentiation of the cells cultured on electrode was studied. The electrode with cells was fixed in the flow-cell, and PBS was injected into the flow-cell for 2 h. EIS data had no obvious changes during measurement. Results demonstrated that cell differentiation had no effect on our experiments.
Experiments were conducted to study the cell differentiation on the carbon screen printed electrode. The electrode with the cells was fixed in the flow-cell, and PBS was injected into the flow-cell for 2 hours. The output frequency data was recorded and analyzed. During experimental procedure, the frequency data did not present obvious changes, which indicated that cell differentiation had no effect on our experiments.
CT quantitative analysis results
Responses of MTITB cell-based sensor to CT solution as the function of measurement time were displayed in ). The interaction between CT and cells fluctuate with the increase of measurement time. In section between a and b, SAWR frequency declined as time elapsed. It may be attributed to the concentration effects of CT to the cells. More cells gathered around the working electrode, leading to an increase in resistance. This increase caused the decline in SAWR frequency. In section between b and c, the frequency accelerated obviously due to the toxin action of CT to the cells. CT interacted with the protein within the cells, resulting in protein denaturation and the density decreases with the cells around the working electrode. The resistance of the electrode declined, which led to the increase in SAWR frequency. Section between c and d was the stable state of this sensor. In this stage, the cell responses to CT reach the saturation state. The electrode resistance was more stable than the other two sections. This was the stable signal reflecting the interactions between CT and the cells. The mean values of this section were selected as the characterization of this sensor. The cells were cultured on the working electrode. According to theoretical analysis, the Ce and Re of the electrode were modified. Because of the dielectric property of viable cells, the electrical current, between two electrodes, was altered by the cells. The larger cell concentration in culture media, the higher interelectrode resistance (Re) the electrode was presented. While interelectrode capacitance (Ce) (move this full names to the place when Re and Ce were first mentioned) change in this study can be ignored. According to the equivalent circuit, a higher Re causes increasing frequency to SAWR oscillator. This is a possible explanation for the obtained results.
Figure 3. Sensor frequency responses: (a) cell culture results, (b) original responses and (c) control experiments results
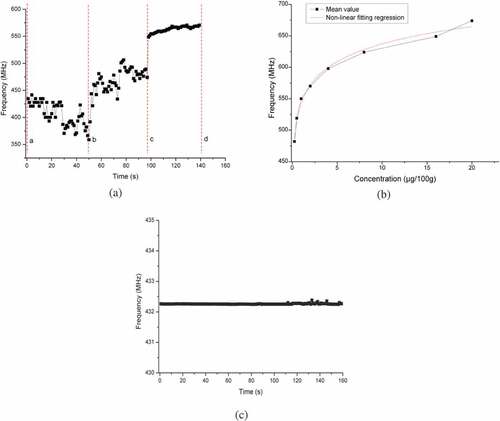
Non-linear fitting regression between SAWR responding frequency and CT concentrations were calculated, and the results are displayed in ) and EquationEquation (1)(1)
(1) . R = 0.9918 indicated that there was a good correlation between SAWR responding frequency and CT concentrations. After mathematical transform, EquationEquation (2)
(2)
(2) is used to characterize CT concentrations:
where x the is the CT concentration. The measurement units are μg/100g for concentration, and the MHz for frequency.
Control experiments results
Results of negative control experiments are displayed in ). The results demonstrated that HEK293 cell-based sensor presented no specific responses to CT solution. The output frequency was around 432.3 MHz. HEK293 cell-based sensor did not have specific responses to CT solutions. The results demonstrated that MTITB cell-based sensor could be selectively respond to CT solution.
Detecting accuracy of the proposed method
To validate the accuracy of the developed detecting system, validating experiments are performed. Additional 15 solutions with unknown CT concentration are prepared and used for validation. Frequency values are measured, and input into EquationEq. (2(2)
(2) ), so the forecasted CT concentration can be obtained. Results are shown in . The detecting accuracy is about 93.3%, indicating that this model is suitable for CT concentration detection. This method provides a rapid way for CT concentration determination.
Table 1. Validation experiments results (√ means right; x means wrong)
LOD
The detecting sensitivity of the sensor is defined as S = Δf/ΔM where Δf represents the shift of the sensor frequency in MHz, and ΔM the change of the shift of the CT concentration in μg/100g. Data analysis was conducted on to explore the LOD of the proposed sensor system. To link these results with the Limit of Detection (LOD) of our sensors, we measured the output frequency of the experimental setup according to changes in CT concentration. The LOD is defined as the minimum input quantity that can be distinguished with more than 99% fidelity, and can be calculated as LOD = 3∈/S, where ∈ is the output uncertainty. Using this formula, we measured a LOD of 3 × 10−2 μg/100g. The method proposed in this paper presented some advantages including rapid analysis, low cost, good accuracy comparing with instrument analysis or chemical examination methods, such as GC/MS, HPLC, etc. It is promising in toxin field rapid detection applications.
Conclusion
In this paper, a novel high-frequency SAWR-based sensor combined with living cells was investigated for shellfish toxin quantitative determination. MTITB cell lines were used as the sensing elements to establish the sensor system for CT quantitative analysis. The sensor device was in serial with SAWR. SAWR output frequency was recorded for further analysis. The CT quantitative determination model was
. Negative control experiments results demonstrated that HEK293 cell-based sensor had no specific responses to CT. The detecting accuracy of the proposed system is 93.3%. The proposed method is promising in CT detection. The LOD of this sensor is about 3 × 10−2 μg/100g.
Disclosure statement
The authors declare no conflict of interest.
Additional information
Funding
References
- Efsa, E. Marine Biotoxins in Shellfish–Okadaic Acid and Analogues. Scientific Opinion of the Panel on Contaminants in the Food Chain. Efsa. J. 2008, 589, 1–62.
- V.L. Trainer, L. Moore, B.D. Bill, N.G. Adams, N. Harrington, J. Borchert, D. da Silva, B.T Eberhart. Diarrhetic Shellfish Toxins and Other Lipophilic Toxins of Human Health Concern in Washington State. Mar. Drugs. 2013, 11, 1815–1835. DOI: 10.3390/md11061815.
- Sassolas, A.; Hayat, A.; Catanante, G.; Marty, J.-L. Detection of the Marine Toxin Okadaic Acid: Assessing Seafood Safety. Talanta. 2012, 105, 306–316. DOI: 10.1016/j.talanta.2012.10.049.
- Campbell, K.; Vilariño, N.; Botana, L. M.; Elliott, C. T. A European Perspective on Progress in Moving Away from the Mouse Bioassay for Marine-Toxin Analysis. TrAC. Trends. Anal. Chem. 2011, 30, 239–253. DOI: 10.1016/j.trac.2010.10.010.
- Luis, M.B.; Natalia, V.; Amparo, A.; Carmen, V.; Carmen, L.; Christopher, T.E.; Katrina, C.; Ana, M.B. The Problem of Toxicity Equivalent Factors in Developing Alternative Methods to Animal Bioassays for Marine-Toxin Detection. TrAC. Trends Anal. Chem. 2010, 29, 1316–1325. DOI: 10.1016/j.trac.2010.09.004.
- Abdelghani, A.; Abdelghani-Jacquin, C.; Hillebrandt, H.; Sackmann, E. A Cell Based Biosensors for Inflammatory Agents Detection. Mater. Sci. Eng C. 2002, 22, 67. DOI: 10.1016/S0928-4931(01)00344-7.
- Wang, P.; Xu, G. X.; Qin, L. F.; Xu, Y.; Li, Y.; Li, R. Cell-Based Biosensors and Its Application in Biomedicine. Sens. Actuators. B Chem. 2005, 108, 576. DOI: 10.1016/j.snb.2004.11.056.
- Gilchrist, K. H.; Giovangrandi, L.; Whittington, R. H.; Kovacs, T. T. A. Sensitivity of Cell-Based Biosensors to Environmental Variables. Biosens. Bioelectron. 2005, 20, 1397. DOI: 10.1016/j.bios.2004.06.007.
- Hoyt, A. E.; Ricco, A. J.; Bartholomew, J. W.; Osbourn, G. C. SAW Sensors for the Room-Temperature Measurement of CO2 and Relative Humidity. Anal. Chem. 1998, 70, 2137–2145. DOI: 10.1021/ac971095z.
- Ge, K.; Liu, D.; Chen, K.; Nie, L.; Yao, S. Assay of Pancreatic Lipase with the Surface Acoustic Wave Sensor System. Anal. Biochem. 1995, 226, 207–211.
- Shen, C. Y.; Huang, C. P.; Huang, W. T. Gas-Detecting Properties of Surface Acoustic Wave Ammonia Sensors. Sens. Actuators. 2004, 101, 1–7. DOI: 10.1016/j.snb.2003.07.016.
- Gardner, J. W.; Cole, M.; Dowson, C. G.; Newton, P.; Sehra, G. Smart Acoustic Sensor for the Detection of Bacteria in Milk. Proc. Int. Conf. Biomed. Eng. 2005, 539–542.
- Leidl, A.; Hartinger, R.; Ruth, M.; Endres, H. E. A New SO2 Sensor System with SAW and IDC Elements. Sens. Actuators. 1996, 34, 339–342. DOI: 10.1016/S0925-4005(96)01825-4.
- Du, X. S.; Ying, Z. H.; Jiang, Y. D.; Liu, Z. X.; Yang, T. J.; Xie, G. Z. Synthesis and Evaluation of a New Polysiloxane as SAW Sensor Coating for DMMP Detection. Sens. Actuators. 2008, 134, 409–413. DOI: 10.1016/j.snb.2008.05.016.
- Zinnai A, Sanmartin C, Taglieri I, G Andrich, F Venturi. Supercritical Fluid Extraction From Microalgae With High Content Of LC-PUFAs. A Case Of Study: Sc-CO 2 Oil Extraction From Schizochytrium Sp. J. Supercrit. Fluids. 2016. DOI:10.1016/j.supflu.2016.05.011.
- Venturi, F.; Sanmartin, C.; Taglieri, I.; Xiaogou, Y.; Andrich, G.; Zinnai, A. The Influence of Packaging on the Sensorial Evolution of White Wine as a Function of the Operating Conditions Adopted during Storage. Agrochimica. 2016a, 60(2), 150–160.