ABSTRACT
The current research was designed to determine the effect of various concentrations (0%, 25%, 40%, and 55%) of fructose, sorbitol and urea plasticizers in cornstarch-based films, with the aim of achieving a new polymer for the application of biodegradable materials. Casting technique was used to prepare the films. The physical, morphological, thermal, and mechanical properties of produced films were evaluated. The results showed that the thickness, moisture content, and water solubility increased with the addition of plasticizer concentration. While the glass transition temperatures showed an insignificant effect with high plasticizer content. Regardless of plasticizer sort, the tensile stress and Young’s modulus of plasticized films decreased as the plasticizer concentrations were raised beyond 25%. Likewise, the relative crystallinity decreased by increasing the plasticizer content from 0% to 25%, but it began to grow once the concentration increased above 25%. The fructose-plasticized films presented consistent and more coherent surfaces compared to sorbitol and urea counterparts, which appeared less homogeneous surfaces with microcracks. In summary, the plasticizers types and concentrations are affected significantly on the properties and performance of the cornstarch-based film. Film plasticized with 25% fructose appeared the finest set of features and achieved the highest mechanical performance among the plasticizers used in this study.
Introduction
The concerns over current environmental issues have forced scientists and engineers to find solutions to ensure a sustainable green environment. Unlike biodegradable wastes, non-biodegradable materials cannot be disposed of easily. Non-biodegradable wastes are those who cannot be decayed or decomposed by natural agents. They last for many years without any degradation.[Citation1] A prominent example of this is synthetic plastic, which is a common source of environmental contamination used in almost every area of life. It is estimated that worldwide production of synthetic plastic is about 140 million tons per year, with an increasing rate of 2.2% per year.[Citation2] In this regard, the production of environmentally friendly materials to be used as an alternative to nonbiodegradable is inevitable.[Citation3–Citation5] Thus, biopolymers such as thermoplastic starches derived from natural sources are one of the most promising alternatives to mitigate the above-mentioned issues. Starches are carbohydrate polymers consisted mainly of a combination of polysaccharide amylose and branched polysaccharide amylopectin with different ratios depends on the botanical source of the starch.[Citation6] Starch is the most biopolymer that is used to produce films with high biodegradability because of its ability to act as linking matrix between fillers. Moreover, it is available, renewable, and cost-effective source.[Citation7] Corn plant is the predominant sources of native starches commercially available. Almost, more than 85% of starch production in the world is extracted from corn tree. The other minor plant sources of native starch are rice, cassava, potato, and wheat.[Citation8] The individual granule of corn contains 70% semi-crystalline starch, and the rest is crude oil, protein, sugar, and ash.[Citation6,Citation9]
Despite their multiple advantages such as biodegradability, availability, recyclability and low cost, the starch-based materials are also known to have several disadvantages like high water sensitivity (hydrophilic character) and less mechanical performance compared to traditional industrial polymers.[Citation10] Therefore, it is necessary to incorporate reinforcement materials like plasticizers to enhance the mechanical performance of these biomasses. The primary role of the plasticizers is reducing the strong attraction of hydrogen bonds within amylose and amylopectin molecules in the starch network, as well as facilitate the mobility rate of the polymer macromolecular chain, this, in turn, minimizes the glass transition temperature and then improves the flexibility and stiffness of starch-based plasticized materials.[Citation11,Citation12]
Recently, a considerable literature has grown up about the use of plasticizers to reinforce the biopolymers products. For instance, urea[Citation13,Citation14], glycerol[Citation15,Citation16], sorbitol[Citation17,Citation18], xylitol[Citation19] fructose, glucose and sucrose[Citation20,Citation21], as well as tri-ethanolamine and glycol[Citation14,Citation22] were used as plasticizers of biodegradable films. This research, therefore, sets out to assess the effect of different plasticizers, namely, fructose (F), sorbitol (S) and Urea (U) with concentrations (0%, 25%, 40%, and 55%) on the physical, morphological, and thermal characteristics of cornstarch (CS) based films as well as the mechanical performance.
Materials and methods
Materials
CS was isolated from granules of fresh corn ear based on the method described by.[Citation23] The CS chemical composition was analyzed in dry form following the procedures reported in.[Citation24] The CS contained 0.26% ash, 7.13% lipid, and 7.7% crude protein. The amylose and amylopectin content of starch was found to be 24.64 g/100g and 75.36 g/100g, respectively, as were measured using the standard in house method (food analytical chemistry) revealed in previous work.[Citation25] The particles of CS varied from polyhedral to a spherical shape, and the majority of distributed particles (89%) had sizes less than 40 μm. Whereas the starch moisture content was 10.45g/100g and the density was 1.4029 g/cm3. The Fructose, Sorbitol, and Urea plasticizers were supplied by Evergreen Engineering & Resources SDN-BHD, Malaysia. According to previous work,[Citation26] almost all plasticizers are compatible with polymers. In practice, the type of plasticizer to be used with polymers is usually selected experimentally through trial and error. Selection criteria depend on the required thermal and mechanical properties as well as the barrier and the rheological properties. However, the use of fructose, sorbitol, and urea for plasticizing starch-based filmmaking was indicated in the introduction section.
Preparation of films
The cornstarch-based films were manufactured by conventional casting procedure. Aqueous solution 100 ml distilled water contained 5 g of pure CS was heated to 85 ± 2°C for 20 min with constant stirring in a thermal water bath. The objective of this step is to provide a homogenous forming suspension. Afterward, the three types of plasticizers were added individually in the forming solution at concentrations 0%, 25%, 40%, or 55% (w/w, dry starch basis). The heating of the solution continued for an additional 20 min at the same temperature. After that, the slurry was left to cool before casting it in a thermal casting dish. The casted mixture was then dehydrated in an air circulation oven at 45°C for 24 h. The dehydrated films were peeled off from the casting dishes and kept in plastic bags at room temperature for a week before characterization processes. Films produced according to the type of plasticizers and concentration were encoded as follows: F25%, F40%, F55% for Fructose, S25%, S40%, S55% for sorbitol, U25%, U40% and U55% for Urea, and CS for none-plasticized CS film (control).
Characterization
Films thickness and density
An electronic caliper (Mitutoyo-Co, Japan) with ± 0.001-inch accuracy was used to calculate the film thickness. The average value of five random measurements for each film was determined to be the exact film thickness. The density of films was measured directly from their volume (v) and weight (m), where the volume of each film was computed from the resultant of the film suggested dimensions (10mmx30mm) times the obtained thickness from the previous step. Therefore, film density (ρ) was calculated via the following equation:
Film moisture content (MC)
The moisture content of the material is defined as the amount of water that could be removed from the material without changing the chemical composition in relation to the weight of the material.[Citation27] MC of the films was measured according to the method introduced by.[Citation28] A known weight film was kept in the oven for 24 h at 105ºC. The weight differences before (w1) and after (w2) heating were used to obtain the MC for each sample indicated by percentage or gram/100g.
Film water solubility (WS)
WS is the amount of chemical substances that can melt in the water at a certain temperature, preventing the loss of plasticizer.[Citation15] Three samples of each film (1x3mm2) were placed in a lab oven for 24 h at 105°C and then weighed (winitial) dry matter. The dry weighted samples were soaked up in a sealed beaker contains 50 ml distilled water for 24 h at ambient temperature. Afterward, the residues of the film were removed from the beaker and were dried for 24 h at 105°C and then reweighed (wfinal) dry matter. WS of each film was measured by:
Thermal gravimetric analyzer (TGA)
An analyzer type (Q500 V20.13 Build 39) was used to perform TGA and DTG tests. Film size 10 mm2 was placed in platinum crucibles under a Nitrogen atmosphere and exposed to heat from ambient temperature to 450°C at a constant rate of 10°C/min. This method of thermal analysis evaluates the thermal stability of samples and measures the mass loss over time as a function of temperature.
Scanning electron microscopy (SEM)
An instrument type Hitachi S-3400N was used to investigate the surface morphological structure of samples. The sample was coated with a thin golden layer before applying an acceleration voltage of 20 kv through a high vacuum. High-resolution images at different magnification factors resulted from this test.
Fourier transform infrared spectroscopy (FTIR)
The FT-IR test was performed by an infrared spectrometer (Bruker vector 22). The spectral frequency range was set between 4000 and 400 cm−1 over a spectral resolution of 4 cm−1. The preparation of specimens was handled by KBr-disk technique.
X-ray diffraction (XRD)
The X-ray diffraction analysis was conducted using 2500 X-ray diffractometer (Rigaku-Tokyo, Japan). The device was managed by 0.02 (θ) s−1 scattering speed within 5◦ to 60◦ (2θ) angular range. Under operating voltage and current of 40 kV and 35 mA, respectively. The crystallinity index (Ci) was measured based on the calculus of crystallinity area (Ac) and amorphous area (Aa) in diffractogram using the equation:
Tensile properties
The mechanical performances of the films were obtained by using 5KN INSTRON tensile machine with D882 (ASTM, 2002) standard. The preparation of film samples and settings of the tensile machine was carried out according to the method reported by.[Citation29,Citation30] Film strip of 10 × 70 mm dimension was fixed between tensile grips and then was dragged out with a crosshead speed of 2 mm/min and grip separation of 30 mm. The variation in the crosshead speed between 0.5 and 10 mm/min does not appear to affect the tensile properties of composite materials.[Citation31] Five replicates were performed for each specimen to find out tensile strength and elastic modulus.
Statistical analyses
The statistical analyses of the experimental data were carried out using Microsoft Excel 2016, and the resulting curves and graphs were plotted using Tecplot 9.0 software.
Results and discussion
Thickness and density
From the data in , it was found that the thickness of the CS-based films increased insignificantly with the addition of the plasticizer concentration. Hence, the films containing 40% and 55% of plasticizers were thicker than films containing 25% plasticizers. These observations may be relevant to the plasticizer function in reconstructing the molecular chain of the polymer film, where the higher plasticizer content generates more spaces, resulting in thickening of the film thickness. A similar explanation about the effect of plasticizers on the film thickness was reported by.[Citation15,Citation32–Citation35] Regarding the film’s density, the type and concentration of plasticizes appeared different influences on films densities. The increase of fructose concentration decreased the density of starch film from 1.559 to 0.988 g/cm3, this consistent with results of Edhirej et al.[Citation29] where they studied the effect of fructose on cassava starch films. Meanwhile, the addition of sorbitol and urea concentration significantly increased the density of films. However, the film plasticized with F55% recorded the lowest value of density (0.988 g/cm3) while, the highest density (5.454 g/cm3) was obtained from U55%-plasticized film.
Table 1. Physical properties of CS-films incorporated with various plasticizers type and concentration
Moisture content and water solubility
Regardless of plasticizer type, the addition of plasticizer concentration from 25% to 40% and then to 55% to some extent increases the water retention of the plasticized films that depend on the plasticizers holding water capacity.[Citation36] However, the effect of urea on the moisture content of CS-films was more noticeable than that of fructose and sorbitol-plasticized films. The difference in water retention of plasticized films was attributed to the resemblance of glucose units in the molecular structure of the plasticizers where the lower resemblance of glucose units causing a weak molecular interaction between the plasticizer and the intermolecular of biopolymer chains. Consequently, the chance of plasticizer to interact with water molecules became greater.[Citation37]
The water solubility of the starch-based film is a key feature property for the applications that may require water insolubility to enhance water resistance as well as product integrity.[Citation38–Citation40] Therefore, due to the hydrophilic nature of the plasticizers, both the type and concentration of plasticizer showed a strong influence on the water solubility of CS-films. The addition of plasticizers reduces the interactions between polymer molecules, and that creates greater free space in the structure of the polymer chain, this, in turn, allows for the penetration of water fragments in the film matrix, thus, maximizing the plasticized film solubility.[Citation29,Citation37]
Thermal gravimetric analyzer (TGA)
CS-plasticized films were thermogravimetrically examined to compare their thermal stability and decomposition characteristics at different concentrations. TGA is an essential analysis used to investigate the degradation and thermal behavior of starch-based materials that were used for industrial and scientific perspectives for biocomposites development.[Citation41–Citation43] presents the thermogravimetric (TGA) and derivative thermogravimetric (DTG) curves of plasticized samples. Apparently, all curves displayed a similar trend, showing that the thermal degradation and mass loss of the CS-based films occurred in three distinctive phases – each phase associated with a peak in the DTG curve, which represents a particular event during heating. The first thermal action occurred at temperatures below 100◦C caused the initial mass loss; this was mainly due to the elimination of moisture and water fragments by evaporation.[Citation44,Citation45] Accordingly, F and U films showed a significant weight loss as compared to S films. This observation is likely to be attributed to the substantial moisture content of F and U films as shown in . Thus, the higher the moisture content, the higher mass loss in this phase. Further heating led to the second weight loss at temperatures between 150°C and 250°C. This loss in mass was mostly ascribed to the volatilization of plasticizer molecules together with water remains. Similar findings were previously reported by.[Citation16,Citation46] The authors stated that most plasticizers begin to evaporate at 150°C. The last mass loss of film’s degradation occurred at temperatures higher than 270◦C. The weight loss in this phase was related to the depolymerization and degradation of carbon chains in starch structure. It is well known that the beginning of the thermal reactions of starches usually occurs at about 300°C.[Citation47,Citation48] From the data in , all films exhibited close decomposition temperatures varied between 277◦C and 296◦C, close to the degradation temperature of the native starch film (control) which recorded its maximum decomposition at 287◦C. These evidences that the incorporation of plasticizers did not change the thermal stability of CS-based films. Such results are consistent with the data obtained in[Citation49], who also studied the effect of different plasticizers (glycerol, sorbitol, and polyvinyl alcohol) on cornstarch-based films, and declared that the rate of degradation of films ranged from 290.9◦C to 295.4◦C.
Table 2. Degradation temperatures and percentage of residue of CS-films incorporated with various plasticizers type and concentration
Figure 1. TGA & DTG curves of CS-plasticized films with various plasticizers type and concentration. (a,b) fructose. (c,d) sorbitol, and (e,f) urea
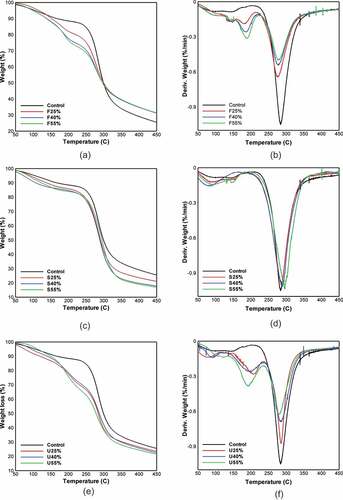
However, the addition of plasticizer concentration affected significantly on the thermal degradation of films, which reflects on the amount of residue after the last decomposition as revealed in . For instance, the percentage of mass residue decreased with the addition of sorbitol as well as with the urea addition, while it increased with the addition of fructose as compared to the control film (24%). This phenomenon confirmed that F-plasticized films are more thermally stable than the S and U-plasticized films at a temperature above 300◦C. These observations are in line with those of previous studies by[Citation29] the researchers investigated the effect of various plasticizers (fructose, urea, tri-ethylene glycol, and triethanolamine) on cassava-based films and stated that fructose films achieved the best thermal stability.
Scanning electron microscopy (SEM)
provides the (SEM) images of none plasticized (control) film along with plasticized films. The micrograph of none plasticized control film showed a uniform and relatively smooth surface with the presence of some non-dissolved starch particles, which is reflecting the morphological structure of CS. The addition of the different plasticizers by 25% to pure starch film showed a little disturbance on film surfaces caused by the high temperature and continuous stirring during preparation. The addition of F25% showed a relatively smooth and coherent surface with no pores, while the surfaces of the S25% and U25% were coarse and covered with some impurities and agglomerates of none melt starch. The films plasticized by 40% and 55% of U and S plasticizers exhibited less consistent surfaces with large porosity as well as microcracks were observed in the structure, unlike the F-films counterpart which revealed better surface integrity compared to the S and U films at the same concentration. However, the appropriate addition of plasticizer concentration to starch-based films supports dissolving of whole starch molecules; improves the structural integrity and coherence of the film surface.[Citation50] Accordingly, the highest proportion of plasticizers used in the manufacturing of starch-films is 60% (w/w dry basis), the exceeding of this limit the produced film appeared to be weak, incoherent and hard to peel off from the casting container. In contrast, the prepared film with less than 15% (w/w dry basis) plasticizer content appeared to be brittle, sticky, and difficult to remove from casting container. Consequently, the evaluation of their properties has become impossible. These observations are in good accord with those mentioned in previous literature.[Citation15,Citation29] For all different plasticizer concentrations in the current study, F-plasticized films evidenced to be rather smooth, coherent and more homogeneous.
Fourier transform infrared (FT-IR) spectroscopy
To investigate the potential interactions between CS and the various plasticizers successfully, the FT-IR spectrum curve has been divided into four main regions as follows: the first region below 800 cm−1 wavenumbers, the second region between 800 and 1500 cm−1 wavenumbers, the third region ranged from 2800 to 3000 cm−1, and the last region above 3000 cm−1 wavenumbers.[Citation51] As seen in the FTIR spectra curves were similar because the elemental composition of the plasticized films was based on the starch structure. The broad overlapping peaks in the first and second regions at 758.02 cm−1 and 933.92 cm−1 for the CS-control film were attributed to the vibrations of glucose pyranose units and C-O vibrational stretching of glucose unit, respectively[Citation51] Likewise, the existence of C-O-H group in CS structure showed up the peak at 1076.33 cm−1, whereas, the coupling of C-C and C-O stretching mode caused the appearance of the sharp band at 1149.01 cm−1. In the same area, the bending mode of CH2 produced a peak at 1367.04 cm−1.[Citation52] For the region between 2800 and 3000 cm−1, the oscillations of water fragments led to the emergence of the wide infrared band at the peak of 1636.44 cm−1.[Citation53,Citation54] Similarly, the occurrence of C-H vibrational stretching resulted in a sharp peak at 2925.27 cm−1. Last, in the fourth area, the O–H group vibrational stretching generated extreme band at 3266.20 cm−1.[Citation55]
Figure 3. FTIR curves of CS-films with various plasticizers type and concentration. (a) fructose, (b) sorbitol, and (c) urea
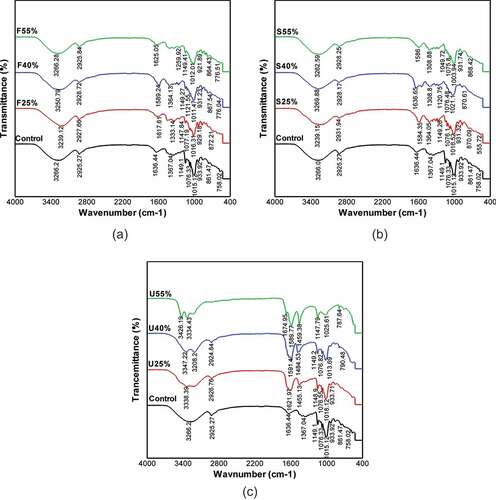
As a result, to plasticizers addition, it was noticed that the FT-IR spectra of F and S films presented identical spectra peaks compared to control film. The only difference, for instance, the sharp peaks which resulted from stretching of the O–H group were shifted slightly to lower wavenumbers for both F and S films. While in the case of U films these peaks have been turned into higher wavenumbers than that in control film. The authors[Citation56,Citation57] in earlier studies have attributed this behavior of U films to its substantial moisture content which affected the hydroxyl groups in starch. Furthermore, the U films offered additional double peaks in the high wavenumber area rather than a single peak for the CS-film and F-S films as well. The prominence of these peaks was ascribed to the stability of hydrogen bonds together with O molecules of both O–C glucose ring and C–O–H group within starch structure.[Citation4] The results mentioned above demonstrated that all films displayed absorption peaks within the same regions, regardless of plasticizer sort and content, this indicates that the plasticizers have similar functional groups, and they are all categorized as polyols.[Citation37] Moreover, FTIR analysis proved that the addition of plasticizers to CS-films did not alter the chemical composition of CS. This phenomenon showed that no chemical reaction occurred and that the chemical structure of the resulting CS-films was completely stable.
X-ray diffraction (XRD)
The X-ray diffraction structures of CS-based samples are introduced in . The results indicated that the majority of CS particles have been gelatinized and retrograded and thus the crystalline framework of the starch was collapsed. The retrograded CS-film owned diffraction peaks located at 15.14°, 17.4°, 18.6°, 20.11°, and 22.8°; Such peaks are effectively compatible with those presented by[Citation58] – however, the addition of plasticizer concentration regardless of the plasticizer type affected on the crystalline structure of films. The F-plasticized films showed a similar pattern to control film, except that the intensity of the diffraction peak at 9.5° increased gradually with increasing fructose concentration from 0% to 55%. Meanwhile, the X-ray diffraction pattern of S-plasticized films revealed a slight increase in the intensity of the peak at 9.4° when the concentration was raised from 0% to 25%, but the intensity of the peaks at 14.4°, 17.4°, and 25.4° turned out to be more pronounced by increasing sorbitol concentration from 25% to 55%. Besides, the crystallinity degree of CS-films is strongly affected by the addition of sorbitol concentration (0–55%) which is reflected in their sharp and clearly defined peaks that associated with the non-crystalline region. Based on that, S40% and S55%-plasticized films were classified as highly crystalline films compared to S25% counterparts; which displays lesser crystallinity. For the U-plasticized films, the XRD pattern shows sharp peaks between 2θ diffraction angles 20◦ and 30◦, which do not exist on control film. The appearance of these peaks was attributed to the typical diffraction pattern C-type model.[Citation59]
Figure 4. XRD of CS-films with various plasticizers type and concentration. (a) fructose, (b) sorbitol, and (c) urea
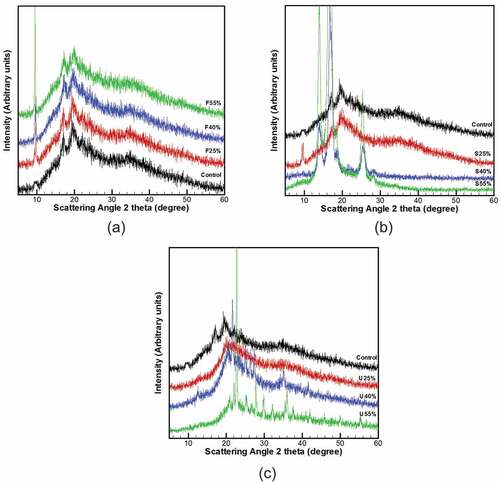
In addition, presents the crystallinity degree of the samples. A significant reduction in all films relative crystallinity was noticed once the plasticizer concentration has risen to 25%. While the further increase in the concentration (25% to 55%) led to increasing the degree of crystallinity. However, the comparison of the plasticized films demonstrated that the S-films possessed a higher crystallinity degree than the U and F-films counterparts. According to[Citation60–Citation62] the increase in crystallinity of starch-based films is strongly associated with the reduced moisture content of the film. Therefore, the increase in crystallinity of S-plasticized films as observed () is compatible with the low moisture content of S-films obtained in this study.
Table 3. Crystallinity index of CS-films incorporated with various plasticizers type and concentration
Mechanical properties
This test is conducted to measure particularly, tensile stress at yield and Young’s modulus for the CS-base films, which plasticized with different plasticizer types and concentrations. From the finding, it is clear that the tensile strength of the tested films decreased as the plasticizer concentration increased from 25% to 55% irrespective to plasticizer type. This conclusion is entirely consistent with that in a previous study conducted by.[Citation12,Citation29]
The film with 25% fructose recorded the highest tensile stress (6.8 MPa) which is higher than that recorded with 25% sorbitol (4.52 MPa) and 25% urea (0.62 MPa) counterparts. The expected interpretation of the high tensile stress at low plasticizer content is related to hydrogen bonds, which formed between starch and plasticizer molecules, these bonds are strongly dominated at lower plasticizer content, and become weaker as content increased.[Citation63,Citation64] Therefore, the tensile strength of F-plasticized films reduced from 6.8 to 3.8 MPa, and that of S-plasticized films decreased from 4.52 to 3.04 MPa as plasticizer percentage increased to 40% and then to 55%. Meanwhile, U-plasticized films recorded the lowest values of tensile stress; the observed reduction was from 0.62 to 0.0448 MPa corresponding to the same range of plasticizer percentage. Many authors have detected the reduction in tensile strength of starch-based films due to increased concentration of plasticizers.[Citation63,Citation65–Citation69]
The tensile strength for fructose films in the current study was higher than those found by[Citation29] the researchers fabricated plasticized films by mixing cassava starch with 30% fructose and achieved 4.7 MPa. Similarly, the same authors incorporated cassava starch with 30% urea and obtained 0.68 MPa which is closer to the current study finding. In general, the tensile strength values for CS-fructose-plasticized films were higher than the previously reported values for cornstarch films plasticized by glycerol[Citation70], cornstarch with stearic acid and glycerol[Citation71], and cornstarch with xylitol and glycerol.[Citation72] On the other hand, the values of tensile strength for CS films in this study were lower than those were made of oxidized and acetylated cornstarch and glycerol[Citation73], and plasticized cornstarch in the presence of glycerol, sorbitol, or PVA.[Citation49]
In relation to elastic modulus (Young’s modulus) which determines the stiffness of materials. The higher value of elastic modulus indicates the optimum stiffness. From , it can be noticed that the effect of plasticizer content (25%–55%) on Young’s modulus of CS-plasticized films has the same behavior compared with their correspondent tensile stress. The increase of plasticizer concentration from 25% to 55% recorded a noticeable decrease in films stiffness: from 61.15 to 29.91 MPa for F-plasticized films, 32.49–15.91 MPa S-plasticized films and 1.67–0.363 MPa for U-plasticized films. This behavior can be explained through the role of plasticizers in modifying the structure of the starch network. When plasticizers integrated into starch chains, they promote the development of hydrogen bonds between the molecules and weakening the solid intra-molecular attraction within the starch matrix. Thus, Young’s modulus of CS-plasticized films is reduced and then became less rigid.[Citation12,Citation29] In short, the mechanical performance of biopolymers based on thermoplastic starches is strongly affected by several parameters such as the botanical origin of starch (amylose/amylopectin ratio), the ambient circumstances (temperature and humidity), processing method as well as plasticizer type and concentration.[Citation74,Citation75]
Conclusion
The effect of different plasticizers types with different concentrations on the physical, morphological, mechanical and thermal characteristics of cornstarch-based films was investigated. The results of the investigation showed that the gradual loading of plasticizers concentration (25%, 40%, 55%) enhanced the performance of the films according to the plasticizer type. The addition of plasticizer concentration increased the thickness, moisture content and water solubility of all films, irrespective of the plasticizer type. However, sorbitol-plasticized films revealed less moisture content than fructose and urea-plasticized films which made sorbitol films achieved the highest degree of relative crystallinity. On the other hand, fructose films recorded lower film thickness and density compared to S and U-plasticized films. Therefore, fructose-plasticized films presented the best performance in terms of physical properties. The study of the morphological structure showed that the fructose-plasticized films provided a homogeneous structure without porosity, which is considerable evidence of their network integrity. Based on that, fructose films obtained the best tensile strength and elastic modulus recording 6.8 and 61.15 MPa, respectively, for the 25% fructose film. In terms of thermal stability, the transition temperatures of all films decreased by increasing plasticizer concentration and moisture content of films as well. While the mass residue at above 350°C increased in case of fructose addition from 24.02% to 29.63%, while it decreased from 24.02% to 16.06 with the addition of sorbitol and from 24.02% to 20.33% with the addition of urea. Hence, the fructose plasticizer evidently enhanced films thermal stability. Overall, the influence of the selected plasticizers at different concentrations on CS base films was verified. The F-plasticized films particularly, 25% fructose film offered the best combination of characteristics make it has the potential to be used for the application and development of biopolymer films.
Additional information
Funding
References
- Razali, N.; Salit, M. S.; Jawaid, M.; Ishak, M. R.; Lazim, Y. A Study on Chemical Composition, Physical, Tensile, Morphological, and Thermal Properties of Roselle Fibre: Effect of Fibre Maturity. Bio. Resources. 2015, 10(1), 1803–1824.
- Shimao, M.;. Biodegradation of Plastics. Curr. Opin. Biotechnol. 2001, 12(3), 242–247.
- Myllärinen, P.; Partanen, R.; Seppälä, J.; Forssell, P. Effect of Glycerol on the Behavior of Amylose and Amylopectin Films. Carbohydr. Polym. 2002, 50(4), 355–361. DOI: 10.1016/S0144-8617(02)00042-5.
- Ma, X. F.; Yu, J.; Wan, J. Urea and Ethanolamine as a Mixed Plasticizer for Thermoplastic Starch. Carbohydr. Polym. 2006, 64(2), 267–273. DOI: 10.1016/j.carbpol.2005.11.042.
- Edhirej, A.; Sapuan, S. M.; Jawaid, M.; Zahari, N. I. Cassava: Its Polymer, Fiber, Composite, and Application. Polym. Compos. 2017, 38(3), 555–570. DOI: 10.1002/pc.v38.3.
- Bertoft, E.;. Understanding Starch Structure: Recent Progress. Agronomy. 2017, 7(3), 56. DOI: 10.3390/agronomy7030056.
- Ghanbarzadeh, B.; Almasi, H.; Entezami, A. A. Improving the Barrier and Mechanical Properties of Corn Starch-Based Edible Films: Effect of Citric Acid and Carboxymethyl Cellulose. Ind. Crops Prod. 2011, 33(1), 229–235. DOI: 10.1016/j.indcrop.2010.10.016.
- Waterschoot, J.; Gomand, S. V.; Fierens, E.; Delcour, J. A. Production, Structure, Physicochemical and Functional Properties of Maize, Cassava, Wheat, Potato and Rice Starches. Starch‐Stärke. 2015, 67(1–2), 14–29. DOI: 10.1002/star.v67.1-2.
- McAloon, A.; Taylor, F.; Yee, W.; Ibsen, K.; Wooley, R. Determining the Cost of Producing Ethanol from Corn Starch and Lignocellulosic Feedstocks, National Renewable Energy Laboratory Report (2000).
- Averous, L.; Boquillon, N. Biocomposites Based on Plasticized Starch: Thermal and Mechanical Behaviours. Carbohydr. Polym. 2004, 56(2), 111–122. DOI: 10.1016/j.carbpol.2003.11.015.
- Sanyang, M.; Sapuan, S.; Jawaid, M.; Ishak, M.; Sahari, J. Effect Of Plasticizer Type And Concentration On Dynamic Mechanical Properties Of Sugar Palm Starch–Based Films. Int. J. Polym. Anal. Charact. 2015, 20(7), 627–636. DOI: 10.1080/1023666X.2015.1054107.
- Sanyang, M. L.; Sapuan, S. M.; Jawaid, M.; Ishak, M. R.; Sahari, J. Effect of Plasticizer Type and Concentration on Tensile, Thermal and Barrier Properties of Biodegradable Films Based on Sugar Palm (Arenga Pinnata) Starch. Polymers. 2015, 7(6), 1106–1124. DOI: 10.3390/polym7061106.
- Wang, J. L.; Cheng, F.; Zhu, P. X. Structure and Properties of Urea-Plasticized Starch Films with Different Urea Contents. Carbohydr. Polym. 2014, 101, 1109–1115. DOI: 10.1016/j.carbpol.2013.10.050.
- Cao, N.; Yang, X.; Fu, Y. Effects of Various Plasticizers on Mechanical and Water Vapor Barrier Properties of Gelatin Films. Food Hydrocolloids. 2009, 23(3), 729–735. DOI: 10.1016/j.foodhyd.2008.07.017.
- Jouki, M.; Khazaei, N.; Ghasemlou, M.; HadiNezhad, M. Effect of Glycerol Concentration on Edible Film Production from Cress Seed Carbohydrate Gum. Carbohydr. Polym. 2013, 96(1), 39–46. DOI: 10.1016/j.carbpol.2013.03.077.
- Versino, F.; López, O. V.; García, M. A. Sustainable Use of Cassava (Manihot Esculenta) Roots as Raw Material for Biocomposites Development. Ind. Crops Prod. 2015, 65, 79–89. DOI: 10.1016/j.indcrop.2014.11.054.
- Mantzari, G.; Raphaelides, S. N.; Exarhopoulos, S. Effect of Sorbitol Addition on the Physicochemical Characteristics of Starch–Fatty Acid Systems. Carbohydr. Polym. 2010, 79(1), 154–163. DOI: 10.1016/j.carbpol.2009.07.043.
- Müller, C. M.; Yamashita, F.; Laurindo, J. B. Evaluation of the Effects of Glycerol and Sorbitol Concentration and Water Activity on the Water Barrier Properties of Cassava Starch Films through a Solubility Approach. Carbohydr. Polym. 2008, 72(1), 82–87. DOI: 10.1016/j.carbpol.2007.07.026.
- Fishman, M.; Coffin, D.; Konstance, R.; Onwulata, C. Extrusion of Pectin/Starch Blends Plasticized with Glycerol. Carbohydr. Polym. 2000, 41(4), 317–325. DOI: 10.1016/S0144-8617(99)00117-4.
- Veiga-Santos, P.; Oliveira, L.; Cereda, M.; Scamparini, A. R. P. Sucrose and Inverted Sugar as Plasticizer. Effect on Cassava Starch–Gelatin Film Mechanical Properties, Hydrophilicity and Water Activity. Food Chem. 2007, 103(2), 255–262. DOI: 10.1016/j.foodchem.2006.07.048.
- Galdeano, M. C.; Mali, S.; Grossmann, M. V. E.; Yamashita, F.; García, M. A. Effects of Plasticizers on the Properties of Oat Starch Films. Mater. Sci. Eng C. 2009, 29(2), 532–538. DOI: 10.1016/j.msec.2008.09.034.
- Audic, J.-L.; Chaufer, B. Influence of Plasticizers and Crosslinking on the Properties of Biodegradable Films Made from Sodium Caseinate. Eur. Polym. J. 2005, 41(8), 1934–1942. DOI: 10.1016/j.eurpolymj.2005.02.023.
- Ali, A.; Wani, T. A.; Wani, I. A.; Masoodi, F. A. Comparative Study of the Physico-Chemical Properties of Rice and Corn Starches Grown in Indian Temperate Climate. J. Saudi Soc. Agric. Sci. 2016, 15(1), 75–82. DOI: 10.1016/j.jssas.2014.04.002.
- Alzorqi, I.; Sudheer, S.; Lu, T. J.; Manickam, S. Ultrasonically Extracted β-d-glucan From Artificially Cultivated Mushroom, Characteristic Properties And Antioxidant Activity. Ultrason. Sonochem. 2017, 35, 531–540. DOI: 10.1016/j.ultsonch.2016.04.017.
- James, C. S.. Analytical Chemistry of Foods; Springer Science & Business Media B. V: Dordrecht, Netherlands, 2013.
- Petrie, E. M. Plasticizers for Adhesives and Sealants. Select Plasticizers according to Base Polymer. SpecialChem. [Online] 2019. https://adhesives.specialchem.com/selection-guide/plasticizers-for-adhesives-and-sealants/according-to-your-base-polymer
- Siebenmorgen, T. J.; Jindal, V. K. Airflow Resistance of Rough Rice as Affected by Moisture Content, Fines Concentration and Bulk Density. Trans. ASAE. 1987, 30(4), 1138–1143. DOI: 10.13031/2013.30533.
- Shogren, R. L.;. Effect of Moisture Content on the Melting and Subsequent Physical Aging of Cornstarch. Carbohydr. Polym. 1992, 19(2), 83–90. DOI: 10.1016/0144-8617(92)90117-9.
- Edhirej, A.; Sapuan, S. M.; Jawaid, M.; Zahari, N. I. Effect of Various Plasticizers and Concentration on the Physical, Thermal, Mechanical, and Structural Properties of Cassava‐Starch‐Based Films. Starch‐Stärke. 2017, 69(1–2), 1500366. DOI: 10.1002/star.v69.1-2.
- Sanyang, M.; Sapuan, S.; Jawaid, M.; Ishak, M.; Sahari, J. Effect of Plasticizer Type and Concentration on Tensile, Thermal and Barrier Properties of Biodegradable Films Based on Sugar Palm (Arenga Pinnata) Starch. Polymers. 2015, 7(6), 1106–1124. DOI: 10.3390/polym7061106.
- Sood, A.; Ramarao, S.; Carounanidy, U. Influence of Different Crosshead Speeds on Diametral Tensile Strength of a Methacrylate Based Resin Composite: An In-Vitro Study. J. Conservative Dent. 2015, 18(3), 214. DOI: 10.4103/0972-0707.157253.
- Ahmadi, R.; Kalbasi-Ashtari, A.; Oromiehie, A.; Yarmand, M.-S.; Jahandideh, F. Development and Characterization of a Novel Biodegradable Edible Film Obtained from Psyllium Seed (Plantago Ovata Forsk). J. Food Eng. 2012, 109(4), 745–751. DOI: 10.1016/j.jfoodeng.2011.11.010.
- Razavi, S. M. A.; Amini, A. M.; Zahedi, Y. Characterisation of a New Biodegradable Edible Film Based on Sage Seed Gum: Influence of Plasticiser Type and Concentration. Food Hydrocolloids. 2015, 43, 290–298. DOI: 10.1016/j.foodhyd.2014.05.028.
- Imran, M.; El-Fahmy, S.; Revol-Junelles, A.-M.; Desobry, S. Cellulose Derivative Based Active Coatings: Effects of Nisin and Plasticizer on Physico-Chemical and Antimicrobial Properties of Hydroxypropyl Methylcellulose Films. Carbohydr. Polym. 2010, 81(2), 219–225. DOI: 10.1016/j.carbpol.2010.02.021.
- Ghasemlou, M.; Khodaiyan, F.; Oromiehie, A. Physical, Mechanical, Barrier, and Thermal Properties of Polyol-Plasticized Biodegradable Edible Film Made from Kefiran. Carbohydr. Polym. 2011, 84(1), 477–483. DOI: 10.1016/j.carbpol.2010.12.010.
- Cerqueira, M. A.; Souza, B. W.; Teixeira, J. A.; Vicente, A. A. Effect Of Glycerol And Corn Oil On Physicochemical Properties Of Polysaccharide films–A Comparative Study. Food Hydrocolloids. 2012, 27(1), 175–184. DOI: 10.1016/j.foodhyd.2011.07.007.
- Sanyang, M. L.; Sapuan, S. M.; Jawaid, M.; Ishak, M. R.; Sahari, J. Effect of Plasticizer Type and Concentration on Physical Properties of Biodegradable Films Based on Sugar Palm (Arenga Pinnata) Starch for Food Packaging. J. Food Sci. Technol. 2016, 53(1), 326–336. DOI: 10.1007/s13197-015-2009-7.
- Bertuzzi, M.; Armada, M.; Gottifredi, J. Physicochemical Characterization of Starch Based Films. J. Food Eng. 2007, 82(1), 17–25. DOI: 10.1016/j.jfoodeng.2006.12.016.
- Perez‐Gago, M.; Krochta, J. Denaturation Time and Temperature Effects on Solubility, Tensile Properties, and Oxygen Permeability of Whey Protein Edible Films. J. Food Sci. 2001, 66(5), 705–710. DOI: 10.1111/jfds.2001.66.issue-5.
- Yin, S.-W.; Tang, C.-H.; Wen, Q. B.; Yang, X.-Q. Properties of Cast Films from Hemp (Cannabis Sativa L.) And Soy Protein Isolates. A Comparative Study. J. Agric. Food Chem. 2007, 55(18), 7399–7404. DOI: 10.1021/jf071117a.
- Liu, X.; Wang, Y.; Yu, L.; Tong, Z.; Chen, L.; Liu, H.; Li, X. Thermal Degradation and Stability of Starch under Different Processing Conditions. Starch‐Stärke. 2013, 65(1‐2), 48–60. DOI: 10.1002/star.v65.1/2.
- Guinesi, L. S.; Da Róz, A. L.; Corradini, E.; Mattoso, L. H. C.; Teixeira, E. D. M.; Curvelo, A. A. D. S. Kinetics of Thermal Degradation Applied to Starches from Different Botanical Origins by Non-Isothermal Procedures. Thermochim. Acta. 2006, 447(2), 190–196. DOI: 10.1016/j.tca.2006.06.002.
- Soares, R.; Lima, A.; Oliveira, R.; Pires, A.; Soldi, V. Thermal Degradation of Biodegradable Edible Films Based on Xanthan and Starches from Different Sources. Polym. Degrad. Stab. 2005, 90(3), 449–454. DOI: 10.1016/j.polymdegradstab.2005.04.007.
- Dang, K. M.; Yoksan, R. Development of Thermoplastic Starch Blown Film by Incorporating Plasticized Chitosan. Carbohydr. Polym. 2015, 115, 575–581. DOI: 10.1016/j.carbpol.2014.09.005.
- Suppakul, P.; Chalernsook, B.; Ratisuthawat, B.; Prapasitthi, S.; Munchukangwan, N. Empirical Modeling of Moisture Sorption Characteristics and Mechanical and Barrier Properties of Cassava Flour Film and Their Relation to Plasticizing–Antiplasticizing Effects. LWT Food Sci. Technol. 2013, 50(1), 290–297. DOI: 10.1016/j.lwt.2012.05.013.
- Vega, D.; Villar, M. A.; Failla, M. D.; Vallés, E. M. Thermogravimetric Analysis of Starch-Based Biodegradable Blends. Polym. Bull. 1996, 37(2), 229–235. DOI: 10.1007/BF00294126.
- Nascimento, T.; Calado, V.; Carvalho, C. Development and Characterization of Flexible Film Based on Starch and Passion Fruit Mesocarp Flour with Nanoparticles. Food Res. Int. 2012, 49(1), 588–595. DOI: 10.1016/j.foodres.2012.07.051.
- Mano, J.; Koniarova, D.; Reis, R. Thermal Properties of Thermoplastic Starch/Synthetic Polymer Blends with Potential Biomedical Applicability. J. Mater. Sci.: Mater. Med. 2003, 14(2), 127–135.
- Isotton, F.; Bernardo, G.; Baldasso, C.; Rosa, L.; Zeni, M. The Plasticizer Effect on Preparation and Properties of Etherified Corn Starchs Films. Ind. Crops Prod. 2015, 76, 717–724. DOI: 10.1016/j.indcrop.2015.04.005.
- Dai, H.; Yu, J.; Geng, F.; Ma, X. Preparation and Properties of Starch-Based Film Using N-(2-Hydroxyethyl) Formamide as a New Plasticizer. Polym.-Plast. Technol. Eng. 2009, 48(8), 866–870. DOI: 10.1080/03602550902994938.
- Kizil, R.; Irudayaraj, J.; Seetharaman, K. Characterization Of Irradiated Starches By Using FT-Raman And Ftir Spectroscopy. J. Agric. Food Chem. 2002, 50(14), 3912–3918.
- Cael, J. J.; Koenig, J. L.; Blackwell, J. Infrared and Raman Spectroscopy of Carbohydrates. Part VI: Normal Coordinate Analysis of V‐Amylose. Biopolymers. 1975, 14(9), 1885–1903. DOI: 10.1002/(ISSN)1097-0282.
- Santha, N.; Sudha, K.; Vijayakumari, K.; Nayar, V.; Moorthy, S. Raman and Infrared Spectra of Starch Samples of Sweet Potato and Cassava. J. Chem. Sci. 1990, 102(5), 705–712. DOI: 10.1007/BF03040801.
- Wilson, R.; Goodfellow, B.; Belton, P.; Osborne, B.; Oliver, G.; Russell, P. Comparison of Fourier Transform Mid Infrared Spectroscopy and near Infrared Reflectance Spectroscopy with Differential Scanning Calorimetry for the Study of the Staling of Bread. J. Sci. Food Agric. 1991, 54(3), 471–483. DOI: 10.1002/(ISSN)1097-0010.
- Pi-Xin, W.; Xiu-Li, W.; Xue, D. H.; Xu, K.; Tan, Y.; Du, X.-B.; Li, W.-B. Preparation And Characterization Of Cationic Corn Starch With A High Degree Of Substitution In dioxane–THF–Water Media. Carbohydr. Res. 2009, 344(7), 851–855. DOI: 10.1016/j.carres.2009.02.023.
- Sahari, J.; Sapuan, S.; Zainudin, E.; Maleque, M. Mechanical and Thermal Properties of Environmentally Friendly Composites Derived from Sugar Palm Tree. Mater. Des. 2013, 49, 285–289. DOI: 10.1016/j.matdes.2013.01.048.
- Sakina, H.; Sarani, Z.; Khairul, D. Oil Palm Biomass: Opportunities and Challenges in Commercial Exploitation. Symposium on Utilisation of Oil Palm Tree Kuala Lumpur 2000.
- Paraginski, R. T.; Vanier, N. L.; Moomand, K.; de Oliveira, M.; Da Rosa Zavareze, E.; E Silva, R. M.; Ferreira, C. D.; Elias, M. C. Characteristics of Starch Isolated from Maize as a Function of Grain Storage Temperature. Carbohydr. Polym. 2014, 102, 88–94. DOI: 10.1016/j.carbpol.2013.11.019.
- Debiagi, F.; Marim, B. M.; Mali, S. Properties of Cassava Bagasse and Polyvinyl Alcohol Biodegradable Foams. J. Polym. Environ. 2015, 23(2), 269–276. DOI: 10.1007/s10924-014-0705-4.
- Gutiérrez, T. J.; Tapia, M. S.; Pérez, E.; Famá, L. Structural and Mechanical Properties of Edible Films Made from Native and Modified Cush-Cush Yam and Cassava Starch. Food Hydrocolloids. 2015, 45, 211–217. DOI: 10.1016/j.foodhyd.2014.11.017.
- Hu, G.; Chen, J.; Gao, J. Preparation and Characteristics of Oxidized Potato Starch Films. Carbohydr. Polym. 2009, 76(2), 291–298. DOI: 10.1016/j.carbpol.2008.10.032.
- Fama, L.; Rojas, A. M.; Goyanes, S.; Gerschenson, L. Mechanical Properties of Tapioca-Starch Edible Films Containing Sorbates. LWT Food Sci. Technol. 2005, 38(6), 631–639. DOI: 10.1016/j.lwt.2004.07.024.
- Muscat, D.; Adhikari, B.; Adhikari, R.; Chaudhary, D. Comparative Study of Film Forming Behaviour of Low and High Amylose Starches Using Glycerol and Xylitol as Plasticizers. J. Food Eng. 2012, 109(2), 189–201. DOI: 10.1016/j.jfoodeng.2011.10.019.
- Adhikari, B.; Chaudhary, D.; Clerfeuille, E. Effect of Plasticizers on the Moisture Migration Behavior of Low-Amylose Starch Films during Drying. Drying Technol. 2010, 28(4), 468–480. DOI: 10.1080/07373931003613593.
- López, O. V.; Lecot, C. J.; Zaritzky, N. E.; García, M. A. Biodegradable Packages Development from Starch Based Heat Sealable Films. J. Food Eng. 2011, 105(2), 254–263. DOI: 10.1016/j.jfoodeng.2011.02.029.
- Dias, A. B.; Müller, C. M.; Larotonda, F. D.; Laurindo, J. B. Biodegradable Films Based on Rice Starch and Rice Flour. J. Cereal Sci. 2010, 51(2), 213–219. DOI: 10.1016/j.jcs.2009.11.014.
- Smits, A.; Kruiskamp, P.; Van Soest, J.; Vliegenthart, J. Interaction between Dry Starch and Plasticisers Glycerol or Ethylene Glycol, Measured by Differential Scanning Calorimetry and Solid State NMR Spectroscopy. Carbohydr. Polym. 2003, 53(4), 409–416. DOI: 10.1016/S0144-8617(03)00119-X.
- Mikkonen, K. S.; Heikkinen, S.; Soovre, A.; Peura, M.; Serimaa, R.; Talja, R. A.; Helén, H.; Hyvönen, L.; Tenkanen, M. Films from Oat Spelt Arabinoxylan Plasticized with Glycerol and Sorbitol. J. Appl. Polym. Sci. 2009, 114(1), 457–466. DOI: 10.1002/app.v114:1.
- Turhan, K. N.; Şahbaz, F. Water Vapor Permeability, Tensile Properties and Solubility of Methylcellulose-Based Edible Films. J. Food Eng. 2004, 61(3), 459–466. DOI: 10.1016/S0260-8774(03)00155-9.
- Dai, H.; Chang, P. R.; Yu, J.; Ma, X.; N‐Bis, N. (2‐Hydroxyethyl) Formamide as a New Plasticizer for Thermoplastic Starch. Starch‐Stärke. 2008, 60(12), 676–684. DOI: 10.1002/star.200800017.
- Pushpadass, H. A.; Bhandari, P.; Hanna, M. A. Effects of LDPE and Glycerol Contents and Compounding on the Microstructure and Properties of Starch Composite Films. Carbohydr. Polym. 2010, 82(4), 1082–1089. DOI: 10.1016/j.carbpol.2010.06.032.
- Fu, Z.-Q.; Wang, L.-J.; Li, D.; Wei, Q.; Adhikari, B. Effects of High-Pressure Homogenization on the Properties of Starch-Plasticizer Dispersions and Their Films. Carbohydr. Polym. 2011, 86(1), 202–207. DOI: 10.1016/j.carbpol.2011.04.032.
- Yan, Q.; Hou, H.; Guo, P.; Dong, H. Effects of Extrusion and Glycerol Content on Properties of Oxidized and Acetylated Corn Starch-Based Films. Carbohydr. Polym. 2012, 87(1), 707–712. DOI: 10.1016/j.carbpol.2011.08.048.
- López, O. V.; Versino, F.; Villar, M. A.; Garcia, M. A. Agro-Industrial Residue from Starch Extraction of Pachyrhizus Ahipa as Filler of Thermoplastic Corn Starch Films. Carbohydr. Polym. 2015, 134, 324–332. DOI: 10.1016/j.carbpol.2015.07.081.
- Yu, L.; Dean, K.; Li, L. Polymer Blends and Composites from Renewable Resources. Prog. Polym. Sci. 2006, 31(6), 576–602. DOI: 10.1016/j.progpolymsci.2006.03.002.