ABSTRACT
The adverse effects of intake nitrite upon human health are well known. However, eating fruits and vegetables is one of the main pathways to absorb nitrite because of nitrogen assimilation in plants. This study demonstrated that during storage of tomato fruit, the production of endogenous hydrogen (H2) was decreased, in parallel with nitrite accumulation and the senescence rate. Furthermore, exogenously applied H2 could delay the decreased fruit H2 production and senescence, but importantly, nitrite accumulation was blocked. Consistently, the activities and transcripts of nitrate reductase (NR; catalyzing the synthesis of nitrite) and nitrite reductase (NiR; responsible for the reduction of nitrite to ammonium), were either inhibited or increased, respectively, by 0.585 mM H2. Decreased or increased nitrite synthesis was observed when tungstate (an inhibitor of NR) or 2,6-dichloroindophenol sodium salt (a putative inhibitor of H2 synthesis) were applied separately. Time-course analysis revealed that the decrease in vitamin C, a well-known nitrite scavenger, was blocked by H2. Overall, this study strongly revealed that nitrite accumulation during storage of tomato fruit was prevented by H2. This study describes potential applications for H2 in agriculture and food industry, especially in the preservation of fruit and vegetable products.
Abbreviations: Ar, argon; DCPIP, 2,6-dichloroindophenol sodium salt; GC, gas chromatograph; H2, hydrogen gas; HPLC, high-performance liquid chromatography; HRW, hydrogen-rich water; N, Newton; N2, nitrogen; NO, nitric oxide; NiR, nitrite reductase; NO2−, sodium nitrite; NO3−, sodium nitrate; NR, nitrate reductase; ONOO−, peroxynitrite anion; qPCR, Real-time quantitative reverse transcription-PCR; RNS, reactive nitrogen species; ROS, reactive oxygen species.
Introduction
Tomato (Solanum lycopersicum cv.) is one of the most important vegetable crops and is consumed widely throughout the world. It is a good source of folate, potassium, and vitamins A, C and E, as well as lycopene and phenolic compounds. There is ample evidence to show that the consumption of tomato fruit and related food products play positive roles in preventing human diseases, such as cardiovascular and chronic degeneration diseases, and age-related macular degeneration. Despite these valuable characteristics, tomato is a climacteric fruit, so that nitrite content may increase during storage which leads to some health adverse problems after human consumption.[Citation1,Citation2] Compared to leafy vegetables, the concentration of NO2− in tomato is typically too low to be problematic for adult health. However, taking into account the large-scale application of nitrogenous fertilizers in China (accounted for >30% of world’s total and exceeded the combined usage in both North America and the European Union in 2009), there has been an increasing threat of nitrate and nitrite accumulation in vegetable foods.[Citation3,Citation4] Additionally, since tomato can be normally stored in local open market or kitchen, and its genome has been sequenced,[Citation5] it has become a suitable plant model to investigate the molecular mechanism(s) relating to NO2− accumulation during storage and retail display.
Sodium and potassium nitrite, which are usually referred to as nitrite salts, have been widely used in meat production industry as preservatives for many centuries.[Citation6] However, nitrite has adverse effects upon human health. Firstly, nitrite can increase methemoglobin content by reacting with hemoglobin, transforming the ferrous iron to the ferric state and then arresting or decreasing the oxygenation of blood. This dangerous disease, which is called methemoglobinemia, is common in infants these days.[Citation7] Nitrite can also generate reactive nitrogen species (RNS), including nitric oxide (NO) and peroxynitrite anion (ONOO−), in both animals and plants.[Citation8,Citation9] Besides the signaling role of NO in the human body, these RNS can also react with body tissues and trigger enzyme inactivation, DNA lesions, lipid peroxidation, damage different organs, and can be considered as one of the most important human dietary carcinogenic factors.[Citation10–Citation12] Nitrite, in particular, can easily react with amides and amines to produce carcinogenic N-nitroso compounds.[Citation13–Citation17] According to some epidemiological and toxicological studies, N-nitroso compounds may potentially increase the risk of esophageal and stomach cancer.[Citation10,Citation18–Citation21] In fact, stomach cancer is globally the third most common cause of death in men, following lung and prostate cancer, and is fourth for women.[Citation18,Citation22]
Fruit and vegetables play an important role in human diet with respect to their provision of minerals, vitamins, protein, carbohydrate and other biologically active compounds.[Citation23] Furthermore, some antioxidant compounds that are found in food, for example, vitamin C, could inhibit nitrite accumulation.[Citation6,Citation24,Citation25] Because of nitrogen assimilation by plants due to nitrogen fertilization, eating fruit and vegetables is one of the main pathways to ingest nitrite.[Citation18,Citation20,Citation26] In addition, during storage after harvesting, nitrite content has been found to increase constantly.[Citation26,Citation27]
In plants, nitrogen assimilation is a basic physiological process, which is necessary for plant growth and development.[Citation28,Citation29] Nitrite is normally generated from nitrate catalyzed by nitrate reductase (NR), a cytosolic enzyme. In this process, NR is a key rate-limiting enzyme of nitrate-to-nitrite reduction process in the plant assimilation pathway, and it catalyzes the NAD(P)H-dependent reduction of nitrate to nitrite. As a result, the regulation of NR activity and its expression plays an important role in the formation of nitrite.[Citation30] After it is transferred to the chloroplast by specific systems, nitrite is reduced to ammonium catalyzed by nitrite reductase (NiR), which later becomes a part of plant organic components including proteins.[Citation31]
Hydrogen gas (H2), mainly catalyzed by hydrogenase and nitrogenase in plants, has recently been identified as a gasotransmitter.[Citation32] A large number of studies have shown that exogenous application of H2 (hydrogen-rich water; HRW) can influence multiple plant responses, including the alleviation of various abiotic stress and the promotion of root development.[Citation33–Citation36] In addition, the application of H2 has been observed to prolong the storage life of fruits and flowers.[Citation37–Citation39] Importantly, the positive effects of H2 involvement in these responses were partially mediated by modulating antioxidant enzyme gene expression and in turn their activities.[Citation34,Citation37] The interplay of H2 and other gasotransmitters has also been observed. For instance, exogenously applied H2-alleviated alfalfa root elogation inhibiton, caused by aluminum stress, was associated with the inhibition of NO generation, with the involvement of NR-mediated NO production being suggested.[Citation33] However, there is yet no information regarding the effect of endogenous H2 on nitrite accumulation in vegetables during storage.
To date, no information regarding the influence of H2 on the preservation of food products during storage by delaying the nitrite accumulation has been reported. The aim of this research was to investigate if and how endogenous H2 could help to prevent nitrite accumulation in tomato during storage. In this study, the effect of H2 on preventing nitrite accumulation was investigated by physicochemical, biochemical and molecular methodology. The results observed in our investigations provided some insights into the molecular mechanisms of H2 in food storage, which permitted the assessment of the potential application of HRW and H2 gas, as suitable approaches to preventing nitrite accumulation during vegetable fruit storage.
Materials and methods
Plant materials
Commercial tomato fruits (Solanum lycopersicum cv. Jiafen No. 2) were purchased from the Suguo market in Nanjing (Jiangsu Province, China) with uniform size and shape without diseases, defects and physical damages. Tomatoes at commercial maturity were transported to the laboratory within 1 h. Before various treatments were applied, the tomato fruit were surface-sterilized by sodium hypochlorite for 3 min, then thoroughly washed with double-distilled water and dried at ambient temperature (25°C). In order to verify H2 function, six other fruits and vegetables (eggplant, lettuce, daylily flower, spinach, apple and carrot) were also stored with the experimental conditions described below.
Preparation of HRW
Purified H2 produced by a H2 generator (SHC-300, Saikesaisi Hydrogen Energy Co., Ltd., Shandong, China) was bubbled into 4000 mL distilled water at a rate of 160 mL min−1 for at least 90 min at ambient temperature, to reach a saturation level (0.78 mM, as determined by gas chromatography, GC).[Citation35] Subsequently, the hydrogen-rich water (HRW) was immediately diluted to 0.195 mM and 0.585 mM to be used in further experiments. The half-life period (concentration) of HRW is 12 h as determined by GC.
Storage condition and phenotypic analysis
Fruits were immersed in a plastic box containing 4000 mL distilled water with or without 0.195, 0.585 and 0.78 mM H2, 200 μM vitamin C, 500 μM tungstate (an inhibitor of NR),[Citation28] 100 μM DCPIP (an artificial electron acceptor, which can interrupt the electron transfer to hydrogenase, then inhibits H2 production),[Citation39,Citation40] 1 mM sodium nitrite (NO2−), 1 mM sodium nitrate (NO3−), either alone or in combination with other components for 20 min at ambient temperature, and then air-dried for 30 min.
Afterwards, to simulate a constant normal or microthermal storage condition, the fruits were placed at 25.0 ± 0.2°C (room temperature; mimicking the temperature in the open market or kitchen) or 4.0 ± 0.2°C (mimicking the temperature in refrigerator) in the plastic container (21 L, Lock & Lock) for 16 d. During storage, samples were taken at the indicated time points for phenotypic, physiological and biochemical analyses.
In order to verify that the inhibition of nitrite production was caused by H2 gas rather than hypoxia, nitrogen (N2)- and argon (Ar)-rich water controls were used. According to the method, as described by the previous report,[Citation38] a H2 fumigation test was also conducted. The tomato fruit were fumigated with 4.5 μL L−1 H2 in a plastic container. The containers were placed in the same treatment condition for the indicated time points at room temperature (25.0 ± 0.2°C). Control group was placed in the same condition without H2.
Flesh firmness measurements
Flesh firmness was determined using a TA-XT2i texture analyzer (Stable Micro System Ltd., U.K.) with a 5 mm probe at a speed of 1 mm s−1. Measurements were performed on the opposite sides of each individual fruit tested. Flesh firmness of fruit was expressed as Newton (N). The higher firmness values meant that samples were more firm.
Determination of H2 content
According to the previous methods,[Citation38] about 0.5 g fresh weight of fruit tissues were homogenized, and then placed in a clean vial, followed by the addition of 5 mL distilled water and 0.2 mL of 5 mM sulphuric acid. Afterward, the inside air of vial was displaced with pure N2, and the vial was immediately capped. H2 content was then analyzed by using an Agilent 7820 model gas chromatograph (GC; Agilent Technologies Inc., USA) equipped with a column containing the Molecular Sieve 5 Å stationary phase and a thermal conductivity detector. The column was held isothermally at 60°C, and the detector and injection temperature regulated at 150°C and 70°C N2, respectively, was used as carrier gas, with the gas pressure being 0.2 MPa.
Detection of nitrite and nitrate contents by high-performance liquid chromatography (HPLC)
According to the method described in the previous report,[Citation18] the concentrations of nitrite and nitrate of fruit tissues were analyzed by HPLC (D-2000, Hitachi Ltd., Tokyo, Japan) with a ZORBAX Eclipse XDB-C18 (250 × 4.6 mm; 5 μm particle size; Fortis Technologies Ltd., Cheshire, UK). The mobile phase was KH2PO4-H3PO4 (0.03 M, pH 3.3), and flow rate was 1.0 mL min−1. After filtration through a 0.22 μm filter (Puradisc 25 NYL; Whatman, Maidstone, UK), all samples were injected into HPLC. The detection wavelength was 214 nm, and the volume of each sample injected into the HPLC was 20 μL. Total run time was 10 min, and detection time of nitrate and nitrite were 3.85 min and 5.95 min, respectively.
NR and NiR activity assay
According to the method described in a previous report,[Citation41] NR activity was assayed with some method modifications. About 5 g of fruit tissues were grinded with extraction buffer containing 50 mM HEPES-KOH (pH 7.5), 20 mM FAD, 2 mM EDTA, 1 mM leupeptin, 5 mM Na2MoO4, and 1% polyvinylpyrrolidone (v/v). After centrifuging at 10,000 g for 20 min at 4°C, the supernatant was obtained. To analyze NR activity, 1 mL of the supernatant was blended with 5 mL of reaction buffer (prewarmed at 25°C, containing 50 mM HEPES-KOH, pH 7.6, 5 mM KNO3 and 0.2 mM NADH), and the mixture was incubated at 25°C for 30 min. The reaction was stopped by adding 0.1 M zinc acetate. After 10 min, the mixture was centrifuged at 13,000 g for 5 min at 4°C. Afterward, supernatant was added with 1 mL of reaction reagent containing 58.1 mM sulfenilamide in 3 M HCl and 1 mL of 1.07 mM N-(1-naphthyl)-ethylenediamine. Finally, the nitrite production was analyzed by colorimetry at 540 nm.
The NiR activity was analyzed by following previous methods with some modifications.[Citation42] The extract was added to a reagent including 0.5 M Tris-HCl buffer (pH 8.0), 20 mM sodium nitrite, 5 mM methyl viologen, sodium dithionite solution (25 mg sodium dithionite dissolved in 1 mL of 0.29 M NaHCO3), and Griess reagent. The mixture was incubated at 30°C for 30 min, and the reaction was stopped by strenuous vortexing. The remaining nitrite content was analyzed by colorimetry at 540 nm.
RNA extraction and analysis of gene transcription
Total RNA was extracted from fruit tissues by homogenizing with pestle and mortar in liquid nitrogen until a fine powder appeared. The powder was transferred to clean tube with TransZol Up Kit (TransGen Biotech, Beijing, China), and then digested with NRase-free DNase. The quantification of RNA was performed by using a NanoDrop 2000 (Thermo Fisher Scientific, Wilmington, USA).
Total first-strand cDNA synthesis was conducted in a 20 μL reaction volume containing 1 μL oligo(dT) primers and 1 μL SuperScript™ reverse transcriptase (Invitrogen, Carlsbad, USA). Real-time quantitative reverse transcription-PCR (qPCR) was carried out by using Mastercycler® ep realplex real-time PCR system (Eppendorf, Hamburg, Germany) with TransStart Top Green qPCR SuperMix (TransGen Biotech, Beijing, China). The genes expression levels of relevant genes were presented as values compared to the control samples, after normalization with two reference genes SlActin and SlGAPDH. The sequences of the primers were given in Supplementary Table S1. The quantification of expression levels of the relative genes was examined by using the 2−ΔΔCT method.[Citation43]
Evaluation of vitamin c content
The determination of vitamin C content was carried out by using a previous method.[Citation44] Tomato fruit tissues were sampled and extracted with trichloroacetic acid solution (0.3 M). After derivatization with 1,2-o-phenylenediamine, quantification of vitamin C was performed by HPLC (D-2000, Hitachi Ltd., Tokyo, Japan). The mobile phase was 0.1 mol L−1 K2HPO4-0.08 mol L−1 KH2PO4-CH3OH (55/25/20, v/v/v), and flow rate was 1.5 mL min−1. Detection was performed by λ (excitation) = 350 nm and λ (emission) = 430 nm. The volume of each sample injected to HPLC was 20 μL. Total run time was 10 min. Quantification was carried out by external calibration with L-ascorbic acid.
Experimental design
According to previous methods with some minor modifications,[Citation39,Citation45] all experiments were arranged in a randomized complete block design. The experiment was designed with three replicates for each. For the determination of NO2− and NO3− content, 10 tomato fruits per replicate were used, and the total tomato fruits in triplicate was 30 (10 × 3). For the other parameters, including firmness, H2 concentration, enzyme (NR and NiR) activity and gene (SlNR and Slnii1) expression level, and vitamin C content, five tomato fruits per replicate were selected and/or sampled for the analyses, and the total tomato fruits in triplicate was 15 (5 × 3). To avoid decayed or overripe zone, all samples were from healthy tissues.
Statistical analysis
The data is presented as the means ± standard error (SE). Statistical analysis was performed using SPSS 18.0 software. The statistical analyses of two groups or multiple groups were performed using t-test or Duncan’s multiple range tests. In all cases, the confidence coefficient was set at 0.05.
Results and discussion
H2 not only delays senescence but also prevents nitrite accumulation during storage
Previous results have shown that a delay in kiwifruit ripening can be achieved by HRW and H2 gas individually.[Citation37,Citation38] In order to evaluate the role of H2 in nitrite accumulation, the contents of nitrite and nitrate in tomato fruit were analyzed by HPLC. Here, our results revealed that exogenous application with HRW not only delayed senescence of tomato fruit but also prevented nitrite accumulation during storage.
Initially, a pilot experiment revealed that pretreatment with HRW containing 0.195, 0.585 and 0.78 mM H2 of tomatoes differentially blocked nitrite accumulation, with contrasting reductions in nitrate contents (Supplementary Figure S1). Given that the responses to 0.585 mM H2 were maximal, HRW containing this concentration of H2 was used in the following experiments.
Since the concentration of H2 in HRW used can reduce the dissolved oxygen content in water (hypoxia),[Citation35] two controls with proportional saturation either argon- or nitrogen-rich water were assessed. As expected, hypoxia failed to alter endogenous nitrite contents during storage of tomato fruit (Supplementary Figure S2), confirming that the responses observed above to HRW were H2-specific. Above conclusion was also supported by the direct H2 fumigation test (Supplementary Figure 3a-b).
Time-course analysis further showed that the symptoms of fruit quality deterioration appeared after 12 d of storage, which was reduced by H2 treatment (). Meanwhile, the decreased firmness during tomato fruit storage was arrested by H2 (, Supplementary Figure S3c). Similar results were obtained when postharvest kiwifruit was treated with H2 gas.[Citation38] During the storage period, endogenous H2 production was progressively decreased (, Supplementary Figure S3d), indicating that H2 homeostasis was disrupted by senescence. Interestingly, the reestablishment of H2 homeostasis was observed when exogenous H2 was applied, indicating that maintenance of homeostatic H2 concentration was critical for delaying fruit ripening during storage.[Citation38]
Figure 1. Exogenously applied H2 not only delayed senescence (a, b), but also slowed down H2 production (c) and nitrate content (d), and nitrite accumulation (e) during storage of tomato fruit. Bar = 5 cm. Asterisk denotes significant difference at P < .05 according to t-test
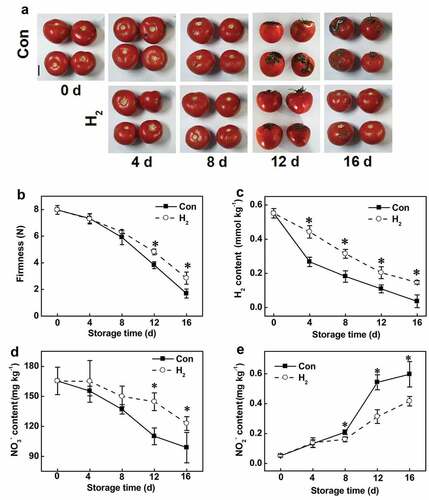
Normally, nitrite and nitrate contents in fruit and vegetables are changed during storage,[Citation26] and both are regarded as standards of freshness.[Citation27] In addition, nitrite has a potential clinical risk.[Citation11,Citation12,Citation23] Importantly, it was observed that H2-induced nitrate production and prevented nitrite accumulation in tomato fruit was in a time-dependent fashion (–). With eggplant, lettuce, daylily flower, spinach, apple and carrot as experimental materials, further time-course experiments showed that H2-prevented nitrite accumulation in these plant materials during storage, demonstrating that H2 was a universal preservation agent (Supplementary Figure S4).
Changes in NR and nir activities and gene expression in response to H2
In the process of nitrite metabolism, NR (catalyzing the synthesis of nitrite) and NiR (responsible for the reduction of nitrite to ammonium) were two of the key enzymes.[Citation28] To investigate whether NR and NiR were regulated by H2, corresponding enzymatic activities were assessed. The results showed that H2 treatment reduced relative NR activity by 42.4% in H2-treated fruit for 8 d, compared to the control fruit (). Conversely, H2 treatment for 4 d significantly increased NiR activity in tomato fruit (). The changes reported above were consistent with the changes of SlNR and Slnii1 transcripts, as analyzed by qPCR (-d). Combined with the changes in nitrite and nitrate contents (-e), the nitrite accumulation prevented by H2 was at least partially, associated with the decreased NR activity.
The inhibition of NR is involved in H2-prevented nitrite accumulation
To better characterize the contribution of NR-generated nitrite production in above H2 response, the effects of the inhibitor of NR (tungstate) on nitrite content and NR activity in the presence and absence of H2, were studied and compared.[Citation28,Citation33,Citation35] Meanwhile, NO3−, a substrate of NR enzyme and an inducer of its synthesis,[Citation30,Citation46] was used as a positive control. As shown in , mimicking the response of H2, nitrite content during storage was markedly reduced by the inhibition of NR activity with tungstate. Consistently, the inhibition of NR activity by H2 was previously reported in aluminum-treated alfalfa seedlings.[Citation33] An additive response was further observed when H2 was added together with tungstate. By contrast, the increased nitrite content triggered by the addition of sodium nitrate, which can increase NR activity and its synthesis,[Citation30] was impaired by H2 (). Similarly, these findings paralleled the changes in NR activity (). Above findings suggested that the inhibition of NR might be involved in H2-prevented nitrite accumulation. Certainly, the role of NiR activity might not be easily ruled out.
Contrasting responses were observed when DCPIP and H2 were applied individually
In order to investigate the possible role of endogenous H2 in preventing nitrite accumulation, a putative synthetic inhibitor of H2, DCPIP was applied.[Citation40] As shown in ,b, delayed or accelerated senescence were observed in tomato fruit when supplemented with H2 and DCPIP, respectively. Consistent with previous reports,[Citation36,Citation40] it was observed that exogenous H2 application could increase endogenous H2 content in tomato fruits, whereas DCPIP inhibited H2 accumulation (). It was also noted that nitrite contents were decreased by H2, but increased by DCPIP, in comparison with the chemical-free tomato fruit (), suggesting the critical role of endogenous H2 homeostasis. This conclusion was further supported by the subsequent results, showing that above H2 responses were sensitive to the addition of DCPIP. Collectively, it was concluded that alterations of endogenous H2 in fruit were closely associated with the senescence process.
Participation of vitamin c in H2-prevented nitrite accumulation
As a major source of antioxidant, vitamin C can react with many clinical health-risk factors, including nitrite.[Citation6,Citation24,Citation25] To investigate the possible role of vitamin C in the above observed H2-prevention of nitrite accumulation, a time-course analysis of vitamin C level during tomato fruit storage, was determined by HPLC after treatment in the presence or absence of H2. Similar to the reduction of endogenous H2 production (), decreased vitamin C levels were observed. In contrast, these changes were partially blocked by the administration of H2 (in particularly at 8 and 12 d; ). These findings were consistent with those reported previously, showing that the decreased vitamin C contents in mercury-stressed alfalfa seedlings were rescued by H2.[Citation47] Importantly, these changes were also negatively correlated to the biological response of nitrite content ().
Figure 5. Time-course analysis revealed that the decreases in vitamin C were slowed down by H2 administration. Asterisk denotes significant difference at P < .05 according to t-test
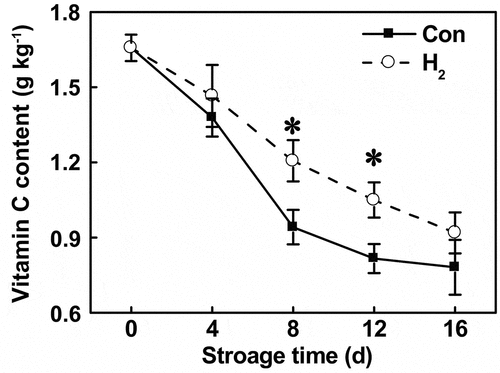
Previous studies suggested that intake of fresh fruit and vegetables containing vitamin C was negatively correlated with cancer of the stomach.[Citation24] Several approaches, including the addition of vitamin C or other antioxidants, have been applied to reduce nitrite content and nitrosamines formation.[Citation6,Citation24,Citation25,Citation48] Accordingly, our results imply a possible interaction between H2 and vitamin C governing NR-mediated nitrite accumulation. It has been reported that H2 could also be an antioxidant, not only selectively scavenging reactive oxygen species (ROS),[Citation49] but also interacting with or modulating vitamin C metabolism.[Citation34,Citation37,Citation50] Therefore, the interaction among H2, vitamin C and ROS with respect to nitrite metabolism during storage requires further verification.
During conditions of cold storage, nitrite level was observed to be lower because low temperature inhibits NR.[Citation46,Citation51] To confirm the role of vitamin C in H2-prevented nitrite accumulation, nitrite contents were determined in both room temperature (25°C) and cold storage conditions (4°C; mimicking the temperature in refrigerator). As shown in , tomato fruit stored at 4°C showed a significant decrease in nitrite content compared to room temperature, suggesting that low temperature decreased nitrite formation by inhibiting NR activity. A subsequent experiment showed that, nitrite accumulation was prevented by vitamin C as well as H2, but increased by exogenously applied NO2−, at either 25°C or 4°C. H2 inhibition of NO2–induced nitrite accumulation was also observed, regardless of the storage temperature. Combined with the previous results that the decreased vitamin C was partially rescued by H2 administration (), we further suggest that H2 may play a role in reducing nitrite accumulation by regulating the endogenous vitamin C level. We advocate that future studies should investigate the extent to which level of vitamin C synthetic gene expression during storage is dependent on H2 signaling pathway.
Conclusion
In summary, this research showed that endogenous H2-prevented nitrite accumulation was due to decreased NR activity and the maintenance of vitamin C content in stored tomato fruit. Considering the other advantages of H2, such as nontoxicity, absence of side effects and easy diffusion, these results identify potential uses of H2 in the agricultural and food industries with respect to the preservation and storage of fruit and vegetable products.
Supplemental Material
Download MS Word (3.1 MB)Acknowledgments
This work was supported by Foshan Agriculture Science and Technology Project (Foshan City Budget No. 140, 2019.). We would like to thank Dr Evan Evans (University of Tasmania; [email protected]) for the English editing of this paper.
Supplementary material
Supplemental data for this article can be accessed here.
Additional information
Funding
References
- Banihani, S. A.;. Tomato (solanum Lycopersicum L.) And Type 2 Diabetes. Int. J. Food Prop. 2018, 21(1), 99–105. DOI: 10.1080/10942912.2018.1439959.
- Santamaria, P.;. Nitrate in Vegetables: Toxicity, Content, Intake and EC Regulation. J. Sci. Food Agric. 2006, 86(1), 10–17. DOI: 10.1002/(ISSN)1097-0010.
- Zhong, W.; Hu, C.; Wang, M. Nitrate and Nitrite in Vegetables from North China: Content and Intake. Food Addit. Contam. 2002, 19(12), 1125–1129. DOI: 10.1080/0265203021000014806.
- Zhang, W. F.; Dou, Z. X.; He, P.; Ju, X.-T.; Powlson, D.; Chadwick, D.; Norse, D.; Lu, Y.-L.; Zhang, Y.; Wu, L.; et al. New Technologies Reduce Greenhouse Gas Emissions from Nitrogenous Fertilizer in China. Proc. Natl. Acad. Sci. U. S. A. 2013, 110(21), 8375–8380. DOI: 10.1073/pnas.1210447110.
- Tomato Genome Consortium. The Tomato Genome Sequence Provides Insights into Fleshy Fruit Evolution. Nature. 2012, 485, 635–641. DOI: 10.1038/nature11096.
- Shpaizer, A.; Nussinovich, A.; Kanner, J.; Tirosh, O. S-Nitroso-N-acetylcysteine Generates Less Carcinogenic N-Nitrosamines in Meat Products than Nitrite. J. Agric. Food Chem. 2018, 66(43), 11459–11467. DOI: 10.1021/acs.jafc.8b04549.
- Kohn, M. C.; Melnick, R. L.; Ye, F.; Portier, C. J. Pharmacokinetics of Sodium Nitrite-induced Methemoglobinemia in the Rat. Drug Metab. Dispos. 2002, 30(6), 676–683. DOI: 10.1124/dmd.30.6.676.
- Ghasemi, A.; Jeddi, S. Anti-obesity and Anti-diabetic Effects of Nitrate and Nitrite. Nitric Oxide. 2017, 70, 9–24. DOI: 10.1016/j.niox.2017.08.003.
- Pimková, K.; Chrastinová, L.; Suttnar, J.; Štikarová, J.; Kotlín, R.; Čermák, J.; Dyr, J. E. Plasma Levels of Aminothiols, Nitrite, Nitrate, and Malondialdehyde in Myelodysplastic Syndromes in the Context of Clinical Outcomes and as a Consequence of Iron Overload. Oxid. Med. Cell. Longev. 2014, 2014, 1–10. DOI: 10.1155/2014/416028.
- Sellimi, S.; Ksouda, G.; Benslima, A.; Nasri, R.; Rinaudo, M.; Nasri, M.; Hajji, M. Enhancing Colour and Oxidative Stabilities of Reduced-nitrite Turkey Meat Sausages during Refrigerated Storage Using Fucoxanthin Purified from the Tunisian Seaweed Cystoseira Barbata. Food Chem. Toxicol. 2017, 107, 620–629. DOI: 10.1016/j.fct.2017.04.001.
- Toyoizumi, T.; Sekiguchi, H.; Takabayashi, F.; Deguchi, Y.; Masuda, S.; Kinae, N. Induction Effect of Coadministration of Soybean Isoflavones and Sodium Nitrite on DNA Damage in Mouse Stomach. Food Chem. Toxicol. 2010, 48(10), 2585–2591. DOI: 10.1016/j.fct.2010.06.015.
- Villaverde, A.; Parra, V.; Estévez, M. Oxidative and Nitrosative Stress Induced in Myofibrillar Proteins by a Hydroxyl-radical-generating System: Impact of Nitrite and Ascorbate. J. Agric. Food Chem. 2014, 62(10), 2158–2164. DOI: 10.1021/jf405705t.
- Herraiz, T.; Galisteo, J. Nitrosative Deamination of 2ʹ-deoxyguanosine and DNA by Nitrite, and Antinitrosating Activity of β-carboline Alkaloids and Antioxidants. Food Chem. Toxicol. 2018, 112, 282–289. DOI: 10.1016/j.fct.2017.12.042.
- Kim, S. H.; Kang, K. H.; Kim, S. H.; Lee, S.; Lee, S.-H.; Ha, E.-S.; Sung, N.-J.; Kim, J. G.; Chung, M. J. Lactic Acid Bacteria Directly Degrade N-nitrosodimethylamine and Increase the Nitrite-scavenging Ability in Kimchi. Food Control. 2017, 71, 101–109. DOI: 10.1016/j.foodcont.2016.06.039.
- Mirvish, S. S.;. Effects of Vitamins C and E on N-nitroso Compound Formation, Carcinogenesis, and Cancer. Cancer. 1986, 58(S8), 1842–1850.
- Van Hecke, T.; Bussche, J. V.; Vanhaecke, L.; Vossen, E.; Van Camp, J.; De Smet, S. Nitrite Curing of Chicken, Pork, and Beef Inhibits Oxidation but Does Not Affect N-nitroso Compound (noc)-specific DNA Adduct Formation during in Vitro Digestion. J. Agric. Food Chem. 2014, 62(8), 1980–1988. DOI: 10.1021/jf4057583.
- Li, L.; Ji, H. Protective Effects of Epicatechin on the Oxidation and N-nitrosamine Formation of Oxidatively Stressed Myofibrillar Protein. Int. J. Food Prop. 2019, 22(1), 185–196. DOI: 10.1080/10942912.2019.1578792.
- Hsu, J.; Arcot, J.; Lee, N. A. Nitrate and Nitrite Quantification from Cured Meat and Vegetables and Their Estimated Dietary Intake in Australians. Food Chem. 2009, 115, 334–339. DOI: 10.1016/j.foodchem.2008.11.081.
- Mirvish, S. S.;. Role of N-nitroso Compounds (NOC) and N-nitrosation in Etiology of Gastric, Esophageal, Nasopharyngeal and Bladder Cancer and Contribution to Cancer of Known Exposures to NOC. Cancer Lett. 1995, 93(1), 17–48. DOI: 10.1016/0304-3835(95)03786-V.
- Prasad, S.; Chetty, A. A. Nitrate-N Determination in Leafy Vegetables: Study of the Effects of Cooking and Freezing. Food Chem. 2008, 106(2), 772–780. DOI: 10.1016/j.foodchem.2007.06.005.
- Rathod, K. S.; Velmurugan, S.; Ahluwalia, A. A ‘green’ Diet-based Approach to Cardiovascular Health? Is Inorganic Nitrate the Answer? Mol. Nutr. Food Res. 2016, 60(1), 185–202. DOI: 10.1002/mnfr.201500313.
- Zhang, P.; Lee, J.; Kang, G.; Li, Y.; Yang, D.; Pang, B.; Zhang, Y. Disparity of Nitrate and Nitrite in Vivo in Cancer Villages as Compared to Other Areas in Huai River Basin, China. Sci. Total Environ. 2018, 612, 966–974. DOI: 10.1016/j.scitotenv.2017.08.245.
- Cemek, M.; Akkaya, L.; Birdane, Y. O.; Seyrek, K.; Bulut, S.; Konuk, M. Nitrate and Nitrite Levels in Fruity and Natural Mineral Waters Marketed in Western Turkey. J. Food Compost. Anal. 2007, 20(3–4), 236–240. DOI: 10.1016/j.jfca.2006.12.003.
- Mirvish, S. S.; Wallcave, L.; Eagen, M.; Shubik, P. Ascorbate-nitrite Reaction: Possible Means of Blocking the Formation of Carcinogenic N-nitroso Compounds. Science. 1972, 177(4043), 65–68. DOI: 10.1126/science.177.4051.788.
- Ohshima, H.; Bartsch, H. Quantitative Estimation of Endogenous Nitrosation in Humans by Monitoring N-nitrosoproline Excreted in the Urine. Cancer Res. 1981, 41(9 part 1), 3658–3662.
- Tamme, T.; Reinik, M.; Püssa, T.; Roasto, M.; Meremäe, K.; Kiis, A.; Van Ree, R.; Giuffrida, M. G.; Conti, A.; Martelli, A. Dynamics of Nitrate and Nitrite Content during Storage of Home-made and Small-scale Industrially Produced Raw Vegetable Juices and Their Dietary Intake. Food Addit. Contam. 2010, 27(4), 487–495. DOI: 10.1080/02652030903225799.
- Phillips, W. E. J.;. Changes in the Nitrate and Nitrite Contents of Fresh and Processed Spinach during Storage. J. Agric. Food Chem. 1968, 16(1), 88–91. DOI: 10.1021/jf60155a012.
- Chamizo-Ampudia, A.; Sanz-Luque, E.; Llamas, A.; Galvan, A.; Fernandez, E. Nitrate Reductase Regulates Plant Nitric Oxide Homeostasis. Trends Plant Sci. 2017, 22(2), 163–174. DOI: 10.1016/j.tplants.2016.12.001.
- Forde, B. G.;. Local and Long-range Signaling Pathways Regulating Plant Responses to Nitrate. Annu. Rev. Plant Biol. 2002, 53(1), 203–224. DOI: 10.1146/annurev.arplant.53.100301.135256.
- Solomonson, L. P.; Barber, M. J. Assimilatory Nitrate Reductase-functional Properties and Regulation. Annu. Rev. Plant Biol. 1990, 41(1), 225–253. DOI: 10.1146/annurev.pp.41.060190.001301.
- Guilherme, E. A.; Carvalho, F. E. L.; Daloso, D. M.; Silveira, J. A. G. Increase in Assimilatory Nitrate Reduction and Photorespiration Enhances CO2 Assimilation under High Light-induced Photoinhibition in Cotton. Environ. Exp. Bot. 2019, 159, 66–74. DOI: 10.1016/j.envexpbot.2018.12.012.
- Li, C.; Gong, T.; Bian, B.; Liao, W. Roles of Hydrogen Gas in Plants: A Review. Funct. Plant Biol. 2018, 45(8), 783–792. DOI: 10.1071/FP17301.
- Chen, M.; Cui, W.; Zhu, K.; Xie, Y.; Zhang, C.; Shen, W. Hydrogen-rich Water Alleviates Aluminum-induced Inhibition of Root Elongation in Alfalfa via Decreasing Nitric Oxide Production. J. Hazard. Mater. 2014, 267, 40–47. DOI: 10.1016/j.jhazmat.2013.12.029.
- Jin, Q.; Zhu, K.; Cui, W.; Xie, Y.; Han, B.; Shen, W. Hydrogen Gas Acts as a Novel Bioactive Molecule in Enhancing Plant Tolerance to Paraquat-induced Oxidative Stress via the Modulation of Heme Oxygenase-1 Signalling System. Plant Cell Environ. 2013, 36(5), 956–969. DOI: 10.1111/pce.12029.
- Xie, Y.; Mao, Y.; Zhang, W.; Lai, D.; Wang, Q.; Shen, W. Reactive Oxygen Species-dependent Nitric Oxide Production Contributes to Hydrogen-promoted Stomatal Closure in Arabidopsis. Plant Physiol. 2014, 165(2), 759–773. DOI: 10.1104/pp.114.237925.
- Cao, Z.; Duan, X.; Yao, P.; Cui, W.; Cheng, D.; Zhang, J.; Jin, Q.; Chen, J.; Dai, T.; Shen, W. Hydrogen Gas Is Involved in Auxin-induced Lateral Root Formation by Modulating Nitric Oxide Synthesis. Int. J. Mol. Sci. 2017, 18(10), 2084. DOI: 10.3390/ijms18102084.
- Hu, H.; Li, P.; Wang, Y.; Gu, R. Hydrogen-rich Water Delays Postharvest Ripening and Senescence of Kiwifruit. Food Chem. 2014, 156, 100–109. DOI: 10.1016/j.foodchem.2014.01.067.
- Hu, H.; Zhao, S.; Li, P.; Shen, W. Hydrogen Gas Prolongs the Shelf Life of Kiwifruit by Decreasing Ethylene Biosynthesis. Postharvest. Biol. Technol. 2018, 135, 123–130. DOI: 10.1016/j.postharvbio.2017.09.008.
- Su, J.; Nie, Y.; Zhao, G.; Cheng, D.; Wang, R.; Chen, J.; Zhang, S.; Shen, W. Endogenous Hydrogen Gas Delays Petal Senescence and Extends the Vase Life of Lisianthus Cut Flowers. Postharvest. Biol. Technol. 2019, 147, 148–155. DOI: 10.1016/j.postharvbio.2018.09.018.
- Florin, L.; Tsokoglou, A.; Happe, T. A Novel Type of Iron Hydrogenase in the Green Alga Scenedesmus Obliquus Is Linked to the Photosynthetic Electron Transport Chain. J. Biol. Chem. 2001, 276(9), 6125–6132. DOI: 10.1074/jbc.M008470200.
- Zhao, M. G.; Chen, L.; Zhang, L. L.; Zhang, W. H. Nitric Reductase-dependent Nitric Oxide Production Is Involved in Cold Acclimation and Freezing Tolerance in Arabidopsis. Plant Physiol. 2009, 151(2), 755–767. DOI: 10.1104/pp.109.146589.
- Debouba, M.; Maâroufi-Dghimi, H.; Suzuki, A.; Ghorbel, M. H.; Gouia, H. Changes in Growth and Activity of Enzymes Involved in Nitrate Reduction and Ammonium Assimilation in Tomato Seedlings in Response to NaCl Stress. Ann. Bot. 2007, 99(6), 1143–1151. DOI: 10.1093/aob/mcm050.
- Livak, K. J.; Schmittgen, T. D. Analysis of Relative Gene Expression Data Using Real-time Quantitative PCR and the 2−ΔΔCT Method. Methods. 2001, 25(4), 402–408. DOI: 10.1006/meth.2001.1262.
- Georgé, S.; Tourniaire, F.; Gautier, H.; Goupy, P.; Rock, E.; Caris-Veyrat, C. Changes in the Contents of Carotenoids, Phenolic Compounds and Vitamin C during Technical Processing and Lyophilisation of Red and Yellow Tomatoes. Food Chem. 2011, 124(4), 1603–1611. DOI: 10.1016/j.foodchem.2010.08.024.
- In, B. C.; Ha, S. T. T.; Lee, Y. S.; Lim, J. H. Relationships between the Longevity, Water Relations, Ethylene Sensitivity, and Gene Expression of Cut Roses. Postharvest. Biol. Technol. 2017, 131, 74–83. DOI: 10.1016/j.postharvbio.2017.05.003.
- Nicholas, J. C.; Harper, J. E.; Hageman, R. H. Nitrate Reductase Activity in Soybeans (glycine Max [L.] Merr.) I. Effects of Light and Temperature. Plant Physiol. 1976, 58(6), 731–735. DOI: 10.1104/pp.58.6.731.
- Cui, W.; Fang, P.; Zhu, K.; Mao, Y.; Gao, C.; Xie, Y.; Wang, J.; Shen, W. Hydrogen-rich Water Confers Plant Tolerance to Mercury Toxicity in Alfalfa Seedlings. Ecotoxicol. Environ. Saf. 2014, 105, 103–111. DOI: 10.1016/j.ecoenv.2014.04.009.
- O’connor, H. J.; Schorah, C. J.; Habibzedah, N.; Axon, A. T.; Cockel, R. Vitamin C in the Human Stomach: Relation to Gastric pH, Gastroduodenal Disease, and Possible Sources. Gut. 1989, 30, 436–442. DOI: 10.1136/gut.30.4.436.
- Ohsawa, I.; Ishikawa, M.; Takahashi, K.; Watanabe, M.; Nishimaki, K.; Yamagata, K.; Katsura, K.-I.; Katayama, Y.; Asoh, S.; Ohta, S. Hydrogen Acts as a Therapeutic Antioxidant by Selectively Reducing Cytotoxic Oxygen Radicals. Nat. Med. 2007, 13(6), 688–694. DOI: 10.1038/nm1577.
- Davey, M. W.; Van Montagu, M.; Inzé, D.; Sanmartin, M.; Kanellis, A.; Smirnoff, N.; Benzie, I. J.; Strain, J. J.; Favell, D.; Fletcher, J. Plant L-ascorbic Acid: Chemistry, Function, Metabolism, Bioavailability and Effects of Processing. J. Sci. Food Agric. 2000, 80(7), 825–860. DOI: 10.1002/(SICI)1097-0010(20000515)80:7<825::AID-JSFA598>3.0.CO;2-6.
- Woodin, S. J.; Lee, J. A. The Effects of Nitrate, Ammonium and Temperature on Nitrate Reductase Activity in Sphagnum Species. New Phytol. 1987, 105(1), 103–115. DOI: 10.1111/nph.1987.105.issue-1.