ABSTRACT
Rice bean (Vigna umbellata) is consumed as a common vegetable in Southeast Asia. Methyl jasmonate (MeJA) functions as an endogenous/exogenous stress signal to elicit the production of specific metabolites during sprouting. To elucidate the changes in metabolites and the effects of MeJA on rice bean sprouting, non-targeted ultra-performance liquid chromatography-quadrupole-time of flight mass spectrometry along with principal component analysis and supervised orthogonal projection to latent structure-discriminant analysis was performed to analyze the dissimilar profiles among the sprouts at different stages. Analysis of the abundance of biomarkers, such as sugars, free fatty acids, amino acids, flavonoids, benzoic acid derivatives, triglycerides, and lipids, showed the main effects of MeJA involved in the significant metabolite pathways. Our study elucidated the changes in rice bean composition and metabolites associated with various stages of sprouting and the roles of MeJA in sprout physiology. Our results can help improve this economically important grain crop.
Introduction
Rice bean (Vigna umbellata), also called as climbing-mountain bean and oriental bean, is native to Southeast Asia and is popular in China. It is consumed as a vegetable or an original food with medicinal usages.[Citation1] During the germination process, primary metabolites of diverse structure and abundance are produced, which play important roles in seedling growth. Furthermore, the hydrolysis or decomposition of some metabolites during sprouting leads to the generation of low molecular-weight chemical constituents, which are of high value to human beings and livestock.[Citation1] For instance, germination could lead to an increase in the level of monosaccharides, amino acids, and organic acids, and decrease in the level of polysaccharides, polyphenols, and long chain fatty acids.[Citation2] Little is known about the changes in primary metabolites during rice bean sprouting.
Studies have evaluated the changes in major metabolites during the germination of edible seeds. During mung bean seed germination, there is continuous accumulation of H2O2, synthesis of nutrient antioxidants, and increase in antioxidant enzyme activities. Reactive oxygen species (ROS) are maintained at a relatively low level via synergistic action of antioxidants and enzymes. Exogenous adenosine triphosphate (ATP) and 2, 4-dinitrophenol (DNP) increased and decreased the intracellular ATP content, respectively.[Citation3] The germination of mung bean is associated with the metabolic pathways of nitrogen, sugar, and amino acids. Oleic acid, linoleic acid, and succinic acid are associated with seeds; while other metabolites including sucrose, maltose, and glucose are closely related to sprouts.[Citation4] Exogenous ATP can maintain the quality of mung bean sprouts by reducing polyphenol oxidase activity, and delaying the browning and softening of mung bean sprouts.[Citation5] Chen et al. found that exogenous ATP supply could further promote the germination of broccoli buds and prevent energy consumption by maintaining the expression level of BoSnRK2 and related genes at a low level. DNP reduced the ATP content, activated the BoSnRK2 pathway, and significantly inhibited energy expenditure activities, including broccoli sprout germination, sprout growth, and secondary metabolic synthesis.[Citation6] The organic broccoli buds were treated with lactic acid (LA, 2% v/v) and low concentration sodium hypochlorite (SH, 4 mg/L). The results showed that aerobic bacteria, yeasts, and mold were effectively reduced and the storage quality of organic broccoli buds was not negatively affected.[Citation7]
Methyl jasmonate (MeJA) is used as an endogenous/exogenous stress signal that accumulates via the octadecanoid pathway to act as a major wounding signal transducer.[Citation8] For sprouting, MeJA treatment can be considered a useful biochemical tool to elicit the production of metabolites in relation to plant defense responses. Metabolites in MeJA-treated rice bean showed that the addition of MeJA during germination significantly increased total flavonoids and polyphenol content and that MeJA had a significant effect on the production of secondary metabolites in the shoots between 4 and 6 h, thereby inducing increased antioxidant activity.[Citation9,Citation10] Methyl jasmonate protects Vigna mungo infected with mungbean yellow mosaic India virus by restoring membrane stability and maintaining ROS homeostasis.[Citation11] Methyl jasmonate can significantly improve the growth and pigmentation of broad bean due to freezing. It could maintain the optimal levels of abscisic acid and salicylic acid, thereby coordinating the defense mechanism to prevent low temperature stress.[Citation12] Oliveira et al. found that MeJA induced systemic defense in dried soybean plants by increasing the level of transcripts encoding pathogenicity-related proteins. The application of exogenous MeJA basically protects leguminous plants from the influence of the necrotizing pathogen Sclerotinia sclerotiorum and reduces the number and size of lesions, consequently reducing the incidence of diseases.[Citation13] Among different elicitors, MeJA is considered to be an effective inducer for the synthesis of secondary metabolites in plant cell/organ culture.[Citation14] Zare-Hassani et al. applied different concentrations of MeJA to stem culture of Ziziphora persica and found that the content of volatile compounds increased.[Citation15] Therefore, MeJA could also be a signal molecule released when a plant is attacked by insects or has a physical wound. The use of MeJA could mimic the defense response of broccoli to insect damage and enhance the production of glucosinolates.[Citation16] In addition, MeJA can be seen as an alternative to improve the quality and health benefits of fruits and vegetables. Preharvest treatment with MeJA can effectively increase pomegranate crop yield, fruit quality, and bioactive compounds during harvest and storage.[Citation17]
In recent years, plant metabolomics has been applied to elucidate and quantify primary metabolites by using various analytical techniques; for example, through liquid chromatography coupled with mass spectrometry (LC/MS).[Citation18,Citation19] It can concurrently identify several primary metabolites and analyze the differences and similarities among samples through principal component analysis (PCA) and supervised orthogonal projection to latent structure discriminant analysis (OPLS-DA). The non-targeted approach has been used to obtain information on the metabolic variability in germplasm banks and differentiate the plant species.[Citation20,Citation21] Metabolomics has also been used as a means to analyze the whole stasis of an organism or changing profile of animal and human internal environment after medical intervention. In addition, nuclear magnetic resonance (NMR) is also a useful method to analyze metabolomics. Chen et al. identified the key metabolites in energy-regulated germination and germination of mung bean by NMR.[Citation4] Furthermore, NMR can be used to characterize the metabolomics characteristics in bacteria. Liu et al. used NMR to analyze Escherichia coli cell metabolite information after electrolyzed water stress.[Citation22] Information on cellular metabolites obtained by NMR could be used as an effective method for identifying the stress effects induced by electrolyzed water.[Citation23]
In the present study, non-targeted metabolites were analyzed using ultra performance liquid chromatography-triple-time-of-flight (UPLC-Triple-TOF) coupled with mass spectroscopy (MS) to investigate the changes in metabolites during rice bean sprouting and the effects of MeJA on the phytochemical profiles of rice bean sprouts during the first 48 h of growth.
Materials and methods
Chemicals and regents
Rice bean (V. umbellata) seeds produced in Jinlin Province, China, were purchased from Yonghui Supermarket. MeJA was purchased from Sigma (St. Louis, MO, USA.). HPLC-grade methanol was purchased from Merck (Germany). Ultra-pure water (18.2 MΩ) was prepared using the Milli-Q water purification system (Millipore, Bedford, MA, USA).
Rice bean sprout cultivation with MeJA induction
Rice bean seeds (15 g) were sterilized with 0.1% NaOCl and soaked in distilled water at 25°C for 6 h (liquid ratio 1:5). The seeds were rinsed with deionized water three times and placed in a dry culture dish. The sprouts were grown in an incubator for 48 h at 25°C; water was automatically sprayed at 1 h intervals. MeJA was dissolved in pure ethanol to prepare stock solutions, which were then diluted to 100 μmol/L and used to treat the seeds during sprouting for metabolic profiling.
Sample extraction
Seed samples (1), 24 h sprouts (2), 48 h sprouts (3), MeJA-treated 24 h sprouts (4), and MeJA-treated 48 h sprouts (5) were ground in liquid nitrogen. Each sample (80 mg) was vortexed, mixed, and extracted twice by ultrasonication in 1 mL of methanol/acetonitrile/water (2:2:1, v/v) for 30 min at 4°C. After precipitation of proteins for 1 h at −20°C, the extracts were centrifuged at 13,000 rpm for 15 min at 4°C. The supernatants were freeze dried and analyzed. In addition, a certain amount of each sample was mixed and used as quality control (QC) sample for LC/MS analysis.
Metabolomics using LC-MS/MS
Metabolomics profile was analyzed using an UHPLC system (1290 Infinity LC; Agilent Technologies) connected to a quadrupole time-of-flight (AB Sciex Triple TOF 6600). The extracted samples were separated using ACQUITY UPLC BEH Amide (1.7 μm, 2.1 mm × 100 mm; Waters Corp., Milford, MA, USA). The mobile phase included A (aqueous 25 mM NH4CH3CO2 and 25 mM NH3) and B (CH3CN). The gradient elution of solutions A and B was as follows: 85% B at 0–1 min, 85%–65% B at 1–12 min, 65%–40% B at 12–12.1 min, 40% B at 12.1–15 min, 40%–85% B at 15–15.1 min, 85% B at 15.1–20 min. The flow rate was 0.3 mL/min. The ESI source conditions were set as follows: ion source gas 1 (Gas1), 60 psi; ion source gas 2 (Gas2), 60 psi; curtain gas (CUR), 30 psi; source temperature, 600°C; ion spray voltage floating (ISVF), 5500 V in the positive mode and −5500 V in the negative mode. The TOF MS scan m/z range was set as 60–1000D and the product ion scan m/z range was set as 25–1000 Da with the instrument accumulation time of 0.05 s/spectra. The product ion scan was acquired using information dependent acquisition (IDA) with the high-sensitivity mode. Collision energy (CE) was fixed at 30 V with ± 15 eV. Declustering potential (DP) was set as ± 60 V. The stability of the established method was confirmed by analyzing QC samples repeatedly injected every 2 h.
Peak identification and data analysis
The original mass spectrometry data were converted into mzml files using protein wizard. The XCMS program was used for feature detection, retention time correction, alignment, and peak area extraction. The detected molecules were matched with accurate mass (< 25 ppm) and the fragmentation pattern of MS spectrum according to the standards database. In the extracted ion features, only the variables having more than 50% of the nonzero measurement values in one group were retained. MetaboAnalyst (www.metaboanalyst.ca) web-based system was used for the multivariate statistical analysis. After Pareto scaling, principal component analysis (PCA) and supervised orthogonal projection to latent structure discriminant analysis (OPLS-DA) were performed to elucidate the metabolite differences among the tested samples. Significantly different metabolites were determined based on a statistically significant threshold of variable influence on projection (VIP) values calculated using the OPLS-DA model and Student’s t-test (p value) on the raw data. Among the obtained metabolites, VIP values larger than 1.0 and results with p values less than 0.1 indicated differences among the sprout samples.
Statistical analysis
All data presented are mean ± SD of six experiments. The data were analyzed using the one-way ANOVA and Student’s t-test with SPSS (version 17.0). Further statistical analyzes of the data obtained by XCMS were conducted using SIMCA-P (version 14.1 Umetrics, Umea, Sweden). PCA and OPLS-DA were run to obtain a general overview of the intrinsic variance of metabolites. Good separation of groups was observed in the analyzed model.
Results and discussion
Effects of MeJA on the growth of rice bean sprouts
Germinating rice-bean seeds were subjected to MeJA treatment. Normal sprouting is accompanied by morphological changes including the growth of cotyledons, roots, hypocotyl, and shoots. In rice bean, the initial morphological changes occur within 48 h. In this stage, the sprouts grow continuously, and their appearance is normal. After this stage, the roots gradually lengthen, which limits sprouts enlargement. In the present study, the MeJA-treated group did not show apparent morphological differences compared with those of the control group. While the mean weight of sprouts in the MeJA-treated group increased consistently during the first stage of sprouting (up to 48 h), the second stage showed a continuous decreasing trend until 96 h during the germination process. Furthermore, at 96 h, the sprouts showed an obvious root enlargement. MeJA (100 μmol/L) had a more significant effect on germination than that at 1.0 mmol/L or 1 μmol/L. The results showed that 100 μmol/L MeJA could promote the metabolite profile changes within 48 h of sprouting initiation.
Metabolite profiling by UHPLC-Q-TOF MS
Comparison of the UHPLC-Q-TOF MS total ion chromatograms (TIC) of QC samples and test samples showed that the retention time and peak intensity were almost overlapping. The results indicated that the variation caused by instrumental error was under control during the experiment. Furthermore, 4746 peaks in the positive ion mode and 5495 peaks in the negative ion mode were extracted from the chromatogram by XCMS. To reveal the differential profile of the developing sprouts of rice beans and the MeJA effects on the metabolites, sprouts at five distinct stages (seeds, 24 h, 48 h, MeJA-24 h, and MeJA-48 h sprouts) were sampled for analysis by UPLC-Q-TOF MS, using six biological replicates for each stage. A heat map was obtained based on the top 200 peaks, which provided the profiles of all samples. Obvious differences in the complex metabolic data among the samples could be observed in the heat map. Moreover, 24 and 48 h sprouts showed differences compared with the seeds ().
Multivariate statistical analysis of metabolite changes during sprouting
The seed and sprout samples at different stages were clearly separated by the unsupervised PCA score plot. All samples were accurately divided by cluster analysis into independent groups, showing differences among seeds and 24 and 48 h sprouts () and those among seeds and MeJA-24 h and MeJA-48 h sprouts () (SIMCA-P and Pareto-scaling). During the sprouting process from seeds to 24 and 48 h sprouts, 139 significant different metabolites in the positive mode were determined. Moreover, 144 significant different metabolites from the seeds and MeJA-24 h and MeJA-48 h sprouts were identified. These were differences in sugars, amino acids, free fatty acids, flavonoids, benzoic acid derivatives, triglycerides, lipids, and amines.
MeJA effects on metabolite profiles during sprouting
The effect of MeJA on the sprouting process was identified using the OPLS-DA model with the profiling data sets. Metabolites at various developmental stages treated with MeJA showed separate clustering for 24 h and MeJA-24 h, and for 48 h and MeJA-48 h, respectively. The OPLS-DA model explained more than 98% (R2) and predicted more than 50% (Q2) of the total variance. The data were very robust as evidenced by the close clustering observed in the six biological replicates within each tested sample. The results showed that the metabolites were significantly affected by MeJA treatment (). An overview of MeJA treatment and the normal group at 24 and 48 h of sprouting was obtained by a heat map based on the complex metabolic data subjected to cluster analysis (). The potential biomarkers were identified by filtering with the variables important in the projection VIP and P values in the statistical analysis. Metabolites with VIP > 1 and P value < .05 were determined as biomarkers with significant differences, and VIP > 1 and 0.05 < P value < .1 were determined as biomarkers with difference.
Figure 3. (a) OPLS-DA plots of 24 h and MeJA-24 h sprouts; (b) OPLS-DA plots of 48 h and MeJA-48 h sprouts
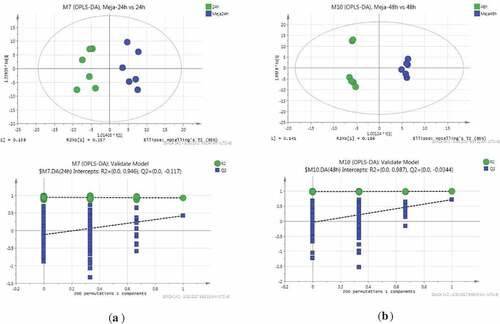
Figure 4. (a) Heat maps of the metabolites in 24 h and MeJA-24 h sprouts; (b) Heat maps of the metabolites in 48 h and MeJA-48h sprouts
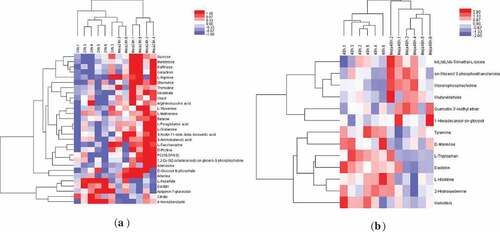
Twenty-nine signature metabolites playing a significant role between groups MeJA-24 h and 24 h were identified by OPLS-DA clustering. The main compounds were amino acids, amines, sugars, sugar alcohols, flavonoid glycosides, organic acids, and fatty acids. On the contrary, only 13 signature metabolites were identified to play a significant role between groups MeJA-48 h and 48 h by OPLS-DA clustering. The main compounds were amino acids, amines, isoflavonones, flavones, monosaccharides, and fatty alcohols. Their relative abundance pattern at each stage was examined (). As shown in , a significant increase in flavonoids daidzein, genistein, quercetin 3′-methyl ether, apigenin 7-glucoside, and daidzin was observed from the seed stage to the sprouting stage. This indicated that sprouting might promote the synthesis of flavonoids. Moreover, sprouts in the MeJA-treated group showed an increase in isoflavone, aglycone, daidzein, and genistein at 24 h, and a decrease in flavone glycosides, apigenin 7-glucoside, and daidzin at 24 h of sprouting, compared with those in the MeJA-untreated group. We concluded that MeJA might promote glycosidic bond cleavage of flavonoids before 24 h after sprouting initiation. This effect by MeJA was short lived and the flavone aglycones decreased at 48 h from sprouting initiation. Among sugars, d-mannose and maltotriose increased significantly, whereas stachyose and sucrose decreased significantly from the seed stage to 24 and 48 h from sprouting initiation. The MeJA-treated seeds showed higher d-mannose and maltotriose levels than the MeJA-untreated seeds at 24 h. The decline in stachyose and sucrose content might be due to the hydrolysis for energy supply. A significant reduction in galactinol was observed in the sprouts at 24 and 48 h, compared with that in the intact seeds. Lipid PC (16:0/16:0) was found to be predominantly decreased in the sprouts. A similar phenomenon of significant decrease in lipid content was observed during the mung beans sprouting process.[Citation2] This indicated that galactinol and lipids act as large energy sources for the developing embryo. Fatty acid methyl esters, such as 1-hexadecanoyl-sn-glycerol, 1,2-di-(9Z-octadecenoyl)-sn-glycero-3-phosphocholine, and glycerol phosphocholine, increased after germination. The overall level of organic acids, such as citrate and benzoic acid derivatives (4-aminobenzoate, argininosuccinic acid, and 3-aminobutanoic acid), decreased during sprouting. A significant increase in the levels of most amino acids (N6,N6,N6-trimethyl-l-lysine, d-proline, l-histidine, l-threonine, l-aspartate, l-arginine, and l-methionine) was observes. Increased content of free amino acids N6,N6,N6-trimethyl-l-lysine, d-proline, l-arginine, and l-threonine in MeJA-treated germinating mung beans and lentils has been reported. The results showed that MeJA could promote the cleavage of proteins and increase the levels of amino acids during germination.[Citation2]
Conclusion
Using a non-targeted metabolomic method, in the present study, for the first time, we describe the changes occurring in the profile of low-molecular-weight metabolites and the effects of MeJA on these profiles in rice beans during sprouting. Metabolites identified as biomarkers during sprouting of rice beans and their induction by MeJA treatment are discussed. The results obtained provide valuable and reliable evidence for further studies, including the analysis of metabolic pathways. The establishment of correlations among metabolite profiles of rice bean sprouts will allow the identification of valuable indicators (biomarkers) that will help to predict the sprouting process and to elaborate the quality of the final food.
Conflict of interest
The authors declare no conflict of interest
Additional information
Funding
References
- Saikia, P.; Sarkar, C. R.; Borua, I. Chemical Composition, Antinutritional Factors and Effect of Cooking on Nutritional Quality of Rice Bean [Vigna umbellata (Thunb; Ohwi and Ohashi)]. Food Chem. 1999, 67(4), 347–352. DOI: 10.1016/S0308-8146(98)00206-4.
- Na Jom, K.; Frank, T.; Engel, K. A Metabolite Profiling Approach to Follow the Sprouting Process of Mung Beans (Vigna radiata). Metabolomics. 2011, 7(1), 102–117. DOI: 10.1007/s11306-010-0236-5.
- Chen, L.; Tan, J. T. G.; Zhao, X.; Yang, D.; Yang, H. Energy Regulated Enzyme and Non-enzyme-based Antioxidant Properties of Harvested Organic Mung Bean Sprouts (Vigna radiata). LWT - Food Sci. Technol. 2019, 107, 228–235. DOI: 10.1016/j.lwt.2019.03.023.
- Chen, L.; Wu, J.; Li, Z.; Liu, Q.; Zhao, X.; Yang, H. Metabolomic Analysis of Energy Regulated Germination and Sprouting of Organic Mung Bean (Vigna radiata) Using NMR Spectroscopy. Food Chem. 2019, 286, 87–97. DOI: 10.1016/j.foodchem.2019.01.183.
- Chen, L.; Zhou, Y.; He, Z.; Liu, Q.; Lai, S.; Yang, H. Effect of Exogenous ATP on the Postharvest Properties and Pectin Degradation of Mung Bean Sprouts (Vigna radiata). Food Chem. 2018, 251, 9–17. DOI: 10.1016/j.foodchem.2018.01.061.
- Chen, L.; Tan, G. J. T.; Pang, X.; Yuan, W.; Lai, S.; Yang, H. Energy Regulated Nutritive and Antioxidant Properties during the Germination and Sprouting of Broccoli Sprouts (Brassica oleracea Var.italica). J. Agr. Food Chem. 2018, 66(27), 6975–6985. DOI: 10.1021/acs.jafc.8b00466.
- Chen, L.; Zhang, H.; Liu, Q.; Pang, X.; Zhao, X.; Yang, H. Sanitising Efficacy of Lactic Acid Combined with Low-concentration Sodium Hypochlorite on Listeria Innocua in Organic Broccoli Sprouts. Int. J. Food Microbiol. 2019, 295, 41–48. DOI: 10.1016/j.ijfoodmicro.2019.02.014.
- Moore, B. S.; Carter, G. T.; Brönstrup, M. Editorial: Are Natural Products the Solution to Antimicrobial Resistance? Nat. Prod. Rep. 2017, 34(7), 685–686. DOI: 10.1039/c7np90026k.
- Li, L.; Gong, X.; Ren, H.; Wang, X.; He, Y.; Dong, Y. Increased Polyphenols and Antioxidant Activity of Rice Bean (vigna Umbellata L.) Sprouts Induced by Methyl Jasmonate: The Promotion Effect of Methyl Jasmonate on Rice Bean Sprouts. Food Sci. Tech-Brazil 2019, 39(suppl 1), 98–104. DOI: 10.1590/fst.36717.
- Li, L.; Dong, Y.; Ren, H.; Xue, Y.; Meng, H.; LI, M. Increased Antioxidant Activity and Polyphenol Metabolites in Methyl Jasmonate Treated Mung Bean (Vigna radiata) Sprouts. Food Sci. Tech-Brazil 2017, 37(3), 411–417. DOI: 10.1590/1678-457x.15716.
- Chakraborty, N.; Basak, J. Exogenous Application of Methyl Jasmonate Induces Defense Response and Develops Tolerance against Mungbean Yellow Mosaic India Virus in Vigna Mungo. Funct. Plant Biol. 2019, 46(1), 69. DOI: 10.1071/FP18168.
- Abd Allah, E. F.; Alqarawi, A. A.; Al-Rashed, S. A.; Hashem, A.; Al-Huqail, A. A.; Aldosari, N. S. Modulation of Adverse Impact of Chilling in Vicia Faba L. By Methyl Jasmonate Involves Changes in Antioxidant Metabolism and Metabolites. Pak. J. Bot. 2016, 5(48), 1915–1923. DOI: 10.1186/s40064-016-3550-1.
- Oliveira, M. B.; Junior, M. L.; Grossi-de-Sá, M. F.; Petrofeza, S. Exogenous Application of Methyl Jasmonate Induces a Defense Response and Resistance against Sclerotinia Sclerotiorum in Dry Bean Plants. J. Plant Physiol. 2015, 182, 13–22. DOI: 10.1016/j.jplph.2015.04.006.
- Yu, K.; Gao, W.; Hahn, E.; Paek, K.-Y. Jasmonic Acid Improves Ginsenoside Accumulation in Adventitious Root Culture of Panax Ginseng C.A. Meyer. Biochem. Eng. J. 2002, 11, 211–215. DOI: 10.1016/S1369-703X(02)00029-3.
- Zare-Hassani, E.; Motafakkerazad, R.; Razeghi, J.; Kosari-Nasab, M. The Effects of Methyl Jasmonate and Salicylic Acid on the Production of Secondary Metabolites in Organ Culture of Ziziphora Persica. Plant Cell Tiss. Org. Culture (PCTOC) 2019, 138(3), 437–444. DOI: 10.1007/s11240-019-01639-x.
- Chiu, Y.; Matak, K.; Ku, K. Methyl Jasmonate Treated Broccoli: Impact on the Production of Glucosinolates and Consumer Preferences. Food Chem. 2019, 299, 125099. DOI: 10.1016/j.foodchem.2019.125132.
- García Pastor, M. E.; Serrano, M.; Guillén, F.; Giménez, M. J.; Martínez‐Romero, D.; Valero, D.; Zapata, P. J. Preharvest Application of Methyl Jasmonate Increases Crop Yield, Fruit Quality and Bioactive Compounds in Pomegranate ‘mollar De Elche’ at Harvest and during Postharvest Storage. J. Sci. Food Agr. 2019. DOI: 10.1002/jsfa.10007.
- Fiehn, O.;. Metabolomics–The Link between Genotypes and Phenotypes. Plant Mol. Biol. 2002, 48(1–2), 155–171.
- Schauer, N.; Zamir, D.; Fernie, A. R. Metabolic Profiling of Leaves and Fruit of Wild Species Tomato: A Survey of the Solanum Lycopersicum Complex. J. Exp. Bot. 2004, 56(410), 297–307. DOI: 10.1093/jxb/eri057.
- Cevallos-Cevallos, J. M.; Reyes-De-Corcuera, J. I.; Etxeberria, E.; Danyluk, M. D.; Rodrick, G. E. Metabolomic Analysis in Food Science: A Review. Trends Food Sci. Tech. 2009, 20(11–12), 557–566. DOI: 10.1016/j.tifs.2009.07.002.
- Oms-Oliu, G.; Odriozola-Serrano, I.; In-Belloso, O. M. Metabolomics for Assessing Safety and Quality of Plant-derived Food. Food Res. Int. 2013, 54(1), 1172–1183. DOI: 10.1016/j.foodres.2013.04.005.
- Liu, Q.; Wu, J.; Lim, Z. Y.; Aggarwal, A.; Yang, H.; Wang, S. Evaluation of the Metabolic Response of Escherichia Coli to Electrolysed Water by 1H NMR Spectroscopy. LWT - Food Sci. Technol. 2017, 79, 428–436. DOI: 10.1016/j.lwt.2017.01.066.
- Liu, Q.; Wu, J.; Lim, Z. Y.; Lai, S.; Lee, N.; Yang, H. Metabolite Profiling of Listeria Innocua for Unravelling the Inactivation Mechanism of Electrolysed Water by Nuclear Magnetic Resonance Spectroscopy. Int. J. Food Microbiol. 2018, 271, 24–32. DOI: 10.1016/j.ijfoodmicro.2018.02.014.