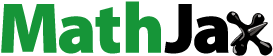
ABSTRACT
For the development of a natural antimicrobial additive based on natural compounds (AC) such as lemongrass essential oil and its active compound citral, encapsulating agents (EA) are required to incorporate it inside food matrixes. Therefore, the effects of type and concentration of EA (maltodextrin and modified-starch called Capsul®) on physical and antimicrobial properties of alginate-based and Lemongrass/citral emulsions were evaluated. Emulsions using different AC:EA ratios were prepared, measuring their antimicrobial (Escherichia coli and Listeria innocua) and physical properties. Antimicrobial activities were observed in all emulsions independently of AC:EA ratio and EA or AC types. However, color differences (ΔE2000) depended on AC:EA ratio and AC used. For both AC, samples with maltodextrin showed higher viscosity and physical stability than Capsul® samples, while the latter showed the highest oxidative stability. In conclusion, EA type affected both physical and oxidative stability of alginate-AC-emulsions; however, both EA could be used for the development of natural antimicrobial additives.
Introduction
Food preservation using natural additives is now requested by consumers, where natural antimicrobials such as essential oils are currently being studied.[Citation1,Citation2] Particularly, essential oils from medicinal plants have been extensively studied due to the benefits in both food preservation and human health.[Citation3] Lemongrass essential oil (LEM) has shown antimicrobial activity against some pathogens,[Citation4] principally attributed to its high concentration of citral, which is a natural mixture of geranial and neral.[Citation4,Citation5] Citral (3, 7-dimethyl-2, 6-octadienal) is the major component (>70%) present in LEM,[Citation6] which is a monoterpene that occurs naturally in herbs, plants, and citrus fruits.[Citation7] Both, LEM and citral, are regarded as Generally Recognized as Safe (GRAS) additives for human consumption by the Food and Drug Administration (FDA).[Citation8] The direct incorporation to food products is a technological challenge to industry due to their lipophilic characteristics, high volatility, and the possibility of drastic changes in flavor and aroma of foods.[Citation3] Thus, encapsulation technology is a simple strategy to incorporate these compounds in foods, both providing a physical barrier to protect them against oxidation or volatilization and ensuring the oil stability inside the food matrix.[Citation9]
For encapsulation, the selection of the encapsulating agent (EA) or surfactant type and its concentration to form the barrier are critical in the formation of emulsions.[Citation10,Citation11] Each surfactant or EA acts differently at the oil–water interface of emulsions depending on its molecular structure, since this determines the ability to adsorb to the oil–water interface and reduce interfacial tension.[Citation12] The incorporation of biopolymers, such as polysaccharides or proteins, increases the viscosity and can improve the emulsion stability by delaying collision between droplets.[Citation13] Currently, the most widely used biopolymers for encapsulation of active compounds (AC) are natural gums (principally alginates), proteins (gelatin, caseinates), carbohydrates (maltodextrins, modified starches), and mixtures thereof.[Citation14] Particularly, sodium alginate suspensions are widely used for stabilization of conventional and nanoemulsions based on essential oils.[Citation10,Citation11,Citation15] Moreover, carbohydrates are preferred for encapsulation because they have high solubility and they generate low viscosities at high solids content. However, a low encapsulation efficiency is observed because of their lack of interfacial properties.[Citation16] Thus, the effect of chemical modifications of carbohydrates, e.g., modified starches, was studied to enhance their hydrophobicity and interactions with oily compounds.[Citation17] Likewise, it is also necessary to consider that the processing method to obtain essential oil-loaded emulsions determines their functionality. The feasible technology for reducing the droplet size of emulsion up to nanometric scale, such as the ultrasound processing, increased the emulsion stability. However, this technology also diminished the antimicrobial potential of lemongrass essential oil–alginate nanoemulsions against E. coli in comparison with the coarse emulsion.[Citation1]
This research considered the final design of active additives in powder, where two types of carbohydrates commonly used to obtain powdered additives by spray drying were studied as encapsulating agents of LEM and citral in alginate-based emulsions. Maltodextrin is a starch obtained by partial hydrolysis, which has a neutral taste, is odorless, and is easily digestible by humans. As encapsulating agent has the ability to protect the core material, minimizing exposure to oxygen and creating a protective barrier on the surface of the particles.[Citation18] Maltodextrin has been used for the encapsulation of various compounds, such as ginger essential oil,[Citation18] among others. The second encapsulating agent is a chemically modified starch (octenyl succinil anhydride–OSA-starch) derived from waxy maize, which is commercially known as Capsul®. The chemical modification is the incorporation of a lipophilic component (octenyl succinate), which helps to obtain good retention of volatile compounds and excellent stability and emulsifier capacity, as in orange essential oil.[Citation19]
The objective of this research was to study the effect of Maltodextrin and Capsul® as EA of LEM and citral as AC on both the antimicrobial and physical properties of emulsions stabilized with alginate for further development of natural antimicrobial additives for spray drying. This study has possible industrial applications due to the actual consumer’s request for natural antimicrobial additives instead synthetic preservatives for food products. The selection of formulation and performance is critical to their effectiveness. Antimicrobial emulsions can be applied directly or to generate powders additives with active properties to increase the shelf life of food matrices. Therefore, both industry and consumers can be benefits by these results.
Materials and methods
Materials
The reagents used for the preparation of active emulsions were sodium alginate (Blumos S.A., Chile) as stabilizing matrix of continuous phase, maltodextrin (Dextrose Equivalent = 19.33) and Capsul® (Quimatic S.A., Chile) as EA, LEM, and citral (Sigma-Aldrich Co., USA) as AC. In addition, hydrochloric acid and sodium hydroxide (used for adjust pH of emulsions) were supplied by Winkler S.A. (Chile) and Arquimed S.A. (Chile), respectively. For antimicrobial measurements, bacteria used were Escherichia coli (ATCC 25922) and Listeria innocua (ATCC 33090), both obtained by Institute of Public Health (ISP, Santiago-Chile). Mueller-Hinton broth (Biokar Diagnostics, France) was used as culture media for microbial growth.
Preparation of emulsions
Active emulsions were prepared adding 1% (w/w) sodium alginate, 1% (w/w) LEM or citral (concentration experimentally determined in previous work), corresponding to twice the minimum inhibitory concentration (0.6% (w/w) for both essential oils). Maltodextrin or Capsul® were added in ratios of active compound to encapsulating agent (AC:EA) of 1:1, 1:2, 1:3, and 1:4. These emulsions were homogenized at 10000 rpm for 2 min using an ultraturrax homogenizer (Thristor Regler, TR50, Germany). pH was adjusted to 5.5 with hydrochloric acid (1 N), where interference from pH on stability of emulsions was not observed (data not shown), being monitored through a pH meter (Jenway, 3505, UK). Three control samples were thus prepared: sodium alginate, sodium alginate with LEM, and sodium alginate with citral.
Physical characterization of emulsions
Droplet size and polydispersity index
To determine average droplet size and its distribution, images of emulsions were captured by light microscope (Carl Zeiss, PrimoStar, Germany) at 100X magnification, using a digital camera (Canon, EOS Rebel T3, Japan). Average droplet sizes of emulsions were estimated from these images using the Motic Images Plus 2.0 software (Motic®, China). The software was previously calibrated. Its values were reported as surface average diameter of droplet, which can be represented by the Sauter diameter d32, following EquationEquation (1)(1)
(1) .
where di is droplet diameter and ni is the number of droplets of size i, respectively. Afterward, a cluster analysis was done using the XLSTAT software (Addinsoft©, Spain) to determine subgroups with maximum homogeneity. At least 1000 droplets were counted for each sample (by triplicate). Polydispersity index (PI) of droplet size distribution of active emulsions was calculated using EquationEquation (2)(2)
(2) , reported by Mabille, Leal-Calderon, Bibette, and Schmitt[Citation20]:
where di is droplet diameter of size i, and is average droplet diameter.
Physical stability and instability rate
Stability of emulsions was determined using an optical stability of dispersions and colloids analyzer (Turbiscan MA2000, Formulaction, France), which is based on the principle of multiple light scattering. For the measurements, emulsions were placed in glass tubes of 120 mm of length and backscattering profiles were analyzed as a function of sample height (total height of the sample in the tube was 65 mm). Emulsions were measured at different storage times (up to 48 h) at 4°C (refrigeration temperature) in a quiescent condition.
To study the instability phenomena of emulsions, the average percentage of backscattering between 7 and 10 mm of tube length was calculated for clarification, between 11 and 50 mm for flocculation/coalescence, and between 51 and 60 mm for creaming in the top of the tube, using MA2000 software (Turbisoft Application, France). Finally, instability kinetics were studied. Rate constants were determined using GraphPad Prism v5 software (GraphPad Prism Inc., USA), which were adjusted to zero-order kinetics model.
Optical properties (color difference and whiteness index)
Optical properties of active emulsions were determined using a calibrated computer vision system (VisionQLab, Chile), described by Matiacevich et al.[Citation21] Samples were prepared on glass plates, using 5 mL of volume to take photographs using a white background. The intensity of RGB digital characteristics was extracted using Adobe Photoshop 7.0 software (Adobe Systems Inc., USA). Finally, they were converted to the CIEL*a*b* color space, where L* indicates lightness, a*, red-green axis, and b*, yellow-blue axis. Color differences of emulsions in relation with control (sodium alginate) were calculated using CIEΔE2000 equation, described by Luo et al.,[Citation22] and the whiteness index (WI) following the equation described by Salvia-Trujillo et al.[Citation23]
Flow behavior
Flow behavior of emulsions was carried out in a rheometer (RheolabQC, Anton Paar GmbH, Austria) with concentric cylinder geometry (bob radius:13.33 mm; gap length:40.008 mm; cup radius:14.46 mm; cone angle:120°) (CC27, Anton Paar GmbH, Austria). Analysis was performed under constant temperature (25 ± 1°C), assisted by a Peltier heating system (Anton Paar, GmbH, Austria) for accurate control. Three batches of each emulsion were prepared. Each batch was measured in duplicate, using a fresh sample for each measurement. The flow curves were obtained by recording shear stress values, where the shear rate was increased linearly from 0 to 100 s−1. Experimental data from ascending flow curves of active emulsions were fitted to the Power law model using GraphPad Prism v5 software (GraphPad Prism Inc., USA).
Antimicrobial properties of emulsions
In order to evaluate the active character of studied emulsions, the growth behaviors of E. coli and L. innocua in the emulsions were studied. For this purpose, bacteria were grown in Mueller-Hinton broth at 37°C for 24 h, in an incubator with constant stirring (NB-205, N-Biotek, Korea). Bacteria concentration (colony forming units (CFU/mL)) was obtained by optical density at 625 nm through a spectrophotometer (UVmini-1240, Shimadzu, Japan), reaching a value of 108 CFU/mL according to the turbidity of McFarland scale.[Citation24] Then, bacterial suspensions were serially diluted until a final concentration of 103 CFU/mL was obtained.
The tests were performed for broth dilution. This was determined in a 96-well plate format. Bacteria (200 µL) were inoculated in concentrated broth 10X (200 µL) and the presence of different emulsions (600 µL), reaching a final bacterial suspension of 102 CFU/mL. Bacterial growth was evaluated at 37°C for 24 h in an automated microplate reader (Multiskan Go, Thermo Scientific, USA).
Oxidative stability of emulsions
The oxidative stability of emulsions was carried out in an oxidation stability tester (Rapidoxy, Anton Paar, Germany), which accelerates the oxidation process by an increase in oxygen pressure and temperature. This method helps to determine the oxidative stability without preliminary sample preparation. Five millliters of sample were placed in a 5 cm diameter plastic dish for measurements. Oxidation conditions were temperature of 120°C and oxygen pressure of 700 kPa. Induction time is recorded when a drop of 10% of oxygen pressure is detected.
Statistical analysis
All experiments were performed in triplicate. Results were reported as mean values and their corresponding standard deviations. Statistical analysis of results was evaluated by ANOVA (analysis of variance) and multiple comparison by Tukey test, with a significance level of 0.05 in order to assess the significance of differences among means, using Statgraphics Centurion XVI software (StatPoint Technologies Inc., USA).
Results and discussion
Droplet size and polydispersity index
The droplet sizes and polydispersity index on emulsions are critical physical parameter due to they are related to physical stability and appearance of the final emulsion.[Citation25] A polymodal behavior was obtained in all studied emulsions, where at least two droplet sizes population were recorded (data not shown). shows the corresponding average droplet sizes of the largest percentage populations, indicated in parenthesis. The droplet sizes of all active emulsions studied were in the range of conventional emulsions (0.75–1.15 µm). No significant differences (p > .05) were observed between them, regardless the type and concentration of EA and AC used. As expected, this result is in accordance to data reported by Klinkesorn et al.[Citation26] for tuna oil-maltodextrin emulsions, since maltodextrin concentration used in this study (4% (w/w)) is lower than the 20% (w/w) reported to obtain droplet size differences attributed to maltodextrin concentration. In the same way, no droplet size differences in samples containing Capsul® were observed, attributed to the adequate concentration used (maximum 4% (w/w)) to being absorbed at the interface and thus, successfully prevented forming larger drops or micelles by flocculation or coalescence.[Citation27] Although the droplet size of nanoemulsions normally decreases as the surfactant concentration increases,[Citation28] in conventional emulsions, this parameter depends on a critical concentration of surfactant of encapsulating agent used.[Citation29]
Table 1. Mean values and standard deviation of average droplet size and polydispersity index for active emulsions with different encapsulating agents and active compound:encapsulating agent ratios (AC:EA). Abbreviations: CIT: Citral; LEM: Lemongrass essential oil; CAP: Capsul®; MD: Maltodextrin
The polydispersity index (PI), which is indicative of the polymodal distribution, was also analyzed. The index allows to characterize the droplet size dispersion in the samples. PI values vary from 0 to 1, where lower values indicate more homogeneous droplets and better dispersion in the emulsion, contributing to reduce agglomeration tendency.[Citation30] As it can be seen in , no significant differences (p > .05) in PI values among samples were observed, but samples containing Capsul® presented a PI less than 0.5. These emulsions are expected to be more stable over time storage.
Physical stability and instability rate
Physical stability over time is an important parameter to determine the emulsion’s shelf life, maintaining its initial physical properties. A change on the backscattering over time is related to loss of stability of an emulsion. shows a representing example of the stability behavior of emulsions at AC:EA 1:4. Instability of emulsions was observed depending on the type of EA (p < .05) rather than their concentration (p > .05) or AC used (p > .05). show a typical creaming mechanism behavior: the droplets rise to the surface increasing the backscattering at the top of the test tube, while at the bottom of the emulsion no particles are observed, and clarification occurs. Flocculation/coalescence is also originated in the middle of the emulsion, where the droplets agglomerate (flocculation) or form larger droplets (coalescence).[Citation29] As observed in , the principal mechanism of destabilization was creaming for emulsions containing Capsul® (), and coalescence/flocculation mechanism was observed in all samples. Emulsions containing Capsul® were therefore less stable than the emulsions containing maltodextrin (), where instability of the former started from 19 h of storage, while the latter remained stable for 48 h, regardless of EA and AC concentration used. Likewise, control samples without any EA were also observed to remain stable throughout the storage time (48 h). The instability observed in emulsions containing Capsul® was not expected; firstly, the addition of EA must increase both the viscosity of samples and, the stability.[Citation31] Secondly, Capsul®-emulsions presented the lowest polidispersity index (), so that their modified structure with hydrophobic and hydrophilic groups must be strongly adsorbed at the oil–water interface, forming a stable system sustained by steric hindrance and electrostatic repulsive forces.[Citation32] However, it is important to note that the optimal degree of substitution (DS) of the commercial modified carbohydrate OSA starches (such as Capsul®) must be approximately 0.07 for stabilizing emulsions.[Citation33] Instead, the Capsul® used in this study has a DS equal to 0.02[Citation34], which is the maximum DS allowed to use in foods, regulated by the FDA. Consequently, at low DS as used in this study, the location of substitution in the middle sections of the starch chains are not available for interactions with oils.[Citation33] Therefore, emulsions physical instability was also reported.[Citation34] In contrast, samples with maltodextrin remained stable, since the critical concentration (20% (w/w)) of maltodextrin with DE20 was not exceeded.[Citation26] Finally, control emulsions also remained stable, this may be due to the presence of alginate, because they help to restrict the movement of the oil droplets, retarding the different mechanisms of destabilization.[Citation35]
Figure 1. Changes in backscattering profiles as function of tube length at different storage time at 4°C and relation active compound:encapsulating AC:AE agent 1:4 for: (a) CIT-CAP; (b) LEM-CAP; (c) CIT-MD; (d) LEM-MD; (e) Control CIT and (f) Control LEM. Abbreviations: CIT: Citral; LEM: Lemongrass essential oil; CAP: Capsul®; MD: Maltodextrin (







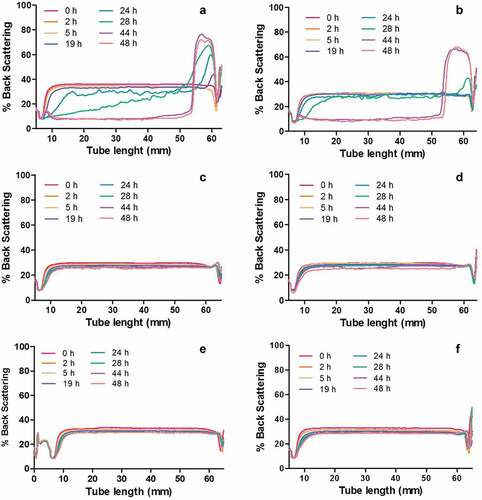
In order to compare the stability between systems with different encapsulating agents, instability rate constants of emulsions were analyzed for each instability mechanisms. In this regard, shows the different instability kinetics for the system with Capsul® and the system with maltodextrin. Here, clarification () and flocculation/coalescence () mechanisms were characterized by negative rate constants, observed by a decrease of backscattering (%BS) over time in the bottom (first 10 mm) and in the middle (between 11 and 50 mm) of the test tube, respectively, regardless of the EA or AC used. In contrast, creaming () mechanism was observed by an increasing of %BS over time in the top (between 51 and 60 mm) of test tube, showing a positive rate constant. On the one hand, the system with Capsul® as EA showed higher rate constants than those observed in the system with maltodextrin for all mechanisms, which was attributed to the destabilization effect caused by Capsul® with low DS. On the other hand, the system with maltodextrin showed rate constants similar (p < .05) to those observed in the control citral (data not shown). These results were correlated to the behaviors observed in , where the stabilizing capacity of these emulsions was attributed to alginate. Ong et al.[Citation35] found that at a relatively low concentration of alginate (1% (w/v)) and storage temperature of 25°C, the oil droplets could be more mobile and promote the agglomeration of the droplets, forming coalescence and possible phase separation, but in the control emulsions of this study, these phenomena were not observed when the emulsions were stored at 4°C, despite containing a similar alginate proportion. Their phenomena were attributed to thermal energy differences between both systems, where a lower thermal energy provides longer stability. Additionally, at lower temperatures, the emulsion viscosity increases, decreasing the molecular mobility and droplets collision rate,[Citation29] extending then the emulsion stability regarding the stability at higher temperatures.
Figure 2. Physical instability kinetics measured as %Backscattering normalized at initial time during storage time at 4°C for samples containing citral (CIT) at a concentration rate of 1:4 oil:encapsulating agent (CAP: Capsul® or MD: maltodextrin); (a) clarification, (b) floculation/coalescence and (c) creaming mechanisms. Bars indicate standard deviation of triplicate means. Lines indicate zero-order kinetics rate fitting and their corresponding equation
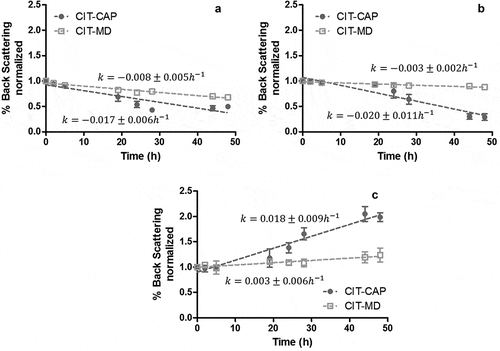
Optical properties: color difference and whiteness index
For many practical applications, the optical properties of emulsions are particularly important. Color differences measured as ΔE2000 were analyzed between control emulsions and emulsions containing different EA for each essential oil. shows that color differences in emulsions were observed depending on both AC and EA used. The emulsions with LEM showed higher color variation (ΔE2000) compared to emulsions with citral using a same EA. Similarly, the color variation increased as the concentration of EA did, regardless of the encapsulated essential oil used. The amount of EA affected then color perception by the human eye.[Citation36]
Figure 3. Color difference (ΔE2000) of active emulsions with lemongrass essential oil (LEM) or citral (CIT), as active compounds, and maltodextrin (MD) or Capsul® (CAP), as encapsulating agents, using alginate as reference. Bars indicate standard deviation of mean from triplicates






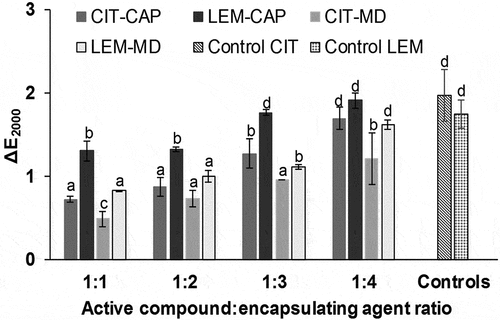
The WI of active emulsions, no significant differences (p > .05) among emulsions and controls were found, regardless of the essential oil or EA used, with an approximate value of 67% (data not shown). This relatively high level of whiteness was found because of the droplet size obtained for the emulsions, since larger particles scatter light more intensely than smaller particles, causing an increase in the WI and opacity of emulsions.[Citation13] In turn, transparent systems must be considered when the whiteness index is lower than 30%, due to the light scattering reduction caused by the small drop sizes.[Citation29] Other studies reported that the WI may also depend on the refractive indexes of continuous (n1) and dispersed phase (n2). Thus, when the ratio of the refractive index of the emulsion (n2/n1) tends to one as in this study (n2 = 1.481 ± 0.002; n1 = 1.33,[Citation37] the light scattering caused by droplets of dispersed lipid emulsion promotes increased opacity.[Citation38]
Flow behavior
The rheological flow properties were adjusted to the power law model. This model was chosen for the analysis of emulsions because it has been widely used to describe rheological behaviors of food emulsions as a function of the shear stress applied to the system.[Citation39] Furthermore, the correlation index (r2) showed its appropriateness when, in all studied emulsions, r2 ~ 1 was obtained (). Thus, the power law constants n (flow index) and K (consistency index) aid in understanding the rheological behavior of the emulsions analyzed.
Table 2. Mean values and standard deviation of flow parameters of active emulsions with different encapsulating agents and active compound:encapsulating agent ratios (AC:EA). Abbreviations: CIT: Citral; LEM: Lemongrass essential oil; CAP: Capsul®; MD: Maltodextrin
The constant n determines the behavior of a fluid, where three ranges of values were found for n < 1, corresponding to a pseudoplastic or reducing shear fluid, n = 1, to a Newtonian fluid, and n > 1, to a thickener shear fluid.[Citation27] summarizes the finding that all emulsions analyzed showed flow indexes (n) close to 1, where no significant differences (p > .05) among samples were found, regardless of which encapsulated oil, EA or concentration was used. This indicates that all emulsions showed a Newtonian behavior. The same behavior was observed for the K, with an overall value of ~0,11Pa sn, which reflects the apparent viscosity of a material to a shear rate of 1s−1.[Citation31] It is important to note that no significant differences (p > .05) were obtained in n and K parameters between emulsions and controls, indicating that flow behaviors of these emulsions are principally attributed to the presence of alginate more than encapsulating agents or essential oils.[Citation35]
The apparent viscosity of the samples with maltodextrin, either concentration or encapsulated essential oil, was nonetheless higher (>100mPa s) than the viscosity of the samples with Capsul® (<90mPa s). Considering that the rheological conditions to obtain a dried powder ingredient by spray drying is a maximum of 300 mPas (according to Büchi Labortechnik AG, Switzerland), the rheological parameters obtained in all emulsions (Newtonian behavior and low viscosity) indicate that all emulsions could be used to obtain dried powder using spray drying. In turn, it is important to consider that the lowest stability of emulsions containing Capsul®, where spray drying might require, It must be performed 19 h before the preparation of emulsions, where clear instability process can be observed ().
Antimicrobial activity
Citral is the active compound present in LEM in a proportion greater than 70%, which is the principal responsible for their antimicrobial activity. Essential oils have the ability to alter and penetrate the lipid structure of the bacterial cell wall. This process causes protein denaturation and subsequent destruction of the cell membrane. Afterward, these oils carry a cytoplasmic filtration and cell lysis, ultimately provoking death.[Citation5] This mechanism of action could be the antimicrobial mechanism of the compounds under study.
The antimicrobial activity of each emulsion was analyzed by growth bacterial kinetics against E. coli and L. innocua. All emulsions completely inhibited both bacterial growth, where no significant differences (p > .05) among samples were observed, regardless of the type of oil (LEM or citral) or EA as well as the AC:EA ratio used (data not shown). This result can be explained because emulsions may promote interactions between essential oil and microbial cell membranes.[Citation40] In addition, EA have an important role in the release of antimicrobial compounds (citral/LEM), which will affect the inhibition against microorganisms. Thus, it is important to choose a suitable EA.
The results showed that regardless of the encapsulating agents (Capsul® and maltodextrin) and the different concentrations analyzed, it was possible to interchangeably inhibit the growth of the studied microorganisms (E. coli and L. innocua). It is important to consider that these results were developed to the concentration of LEM and citral present in the emulsion, which was previously selected as the minimal inhibitory concentration (MIC) (0.6% (w/w)) for both bacteria, which is in accordance with literature reports.[Citation2,Citation41]
Although efficient antimicrobial emulsions were performed in this study, it is important to note that this activity could be lost when powder additives are obtained from these antimicrobial emulsions. More significantly, the antimicrobial efficacy of EO may also be influenced by encapsulating agent used, pH, fat content or water activity of the food matrix. Hence, plant-derived antimicrobials may bind to lipids, proteins, or carbohydrates in foodstuffs, requiring higher concentrations than those used in in vitro studies to achieve the same effect.[Citation42]
Oxidative stability
Oxidative emulsion stability is an important point for essential oil shelf-life. Defined as the resistance to oxidation under established conditions, the best oxidative stability for an oil sample is related to longer induction time. In the Rapidoxy test, the induction period is calculated when there is a decrease in pressure of 10%, which it is not more than the consumption of oxygen by the sample to perform autoxidation. shows the induction time for samples containing lemongrass essential oil, where similar results were observed in samples containing citral (p > .05). Induction time, of all emulsions containing AC (>20 h), was higher than each pure essential oil (~30 min). Similarly, significant differences were observed (p < .05) between control sample (alginate with each essential oil) (~20 h) and each emulsion with EA. As expected, both Capsul® and maltodextrin could protect the AC, improving its encapsulation with alginate and preventing its oxidation. The longest induction times (greater than 30 h) were observed in the samples containing Capsul® at 1:2; 1:3, and 1:4 of AC:EA. This result was attributed to the ability of this EA to interacts with EO due to its amphipathic nature. Here, the hydrophobic tail of Capsul® can be absorbed into oil phase, reducing surface tension of the oil–water interface,[Citation32] thus contributing to isolate the EO from oxygen. Likewise, the samples containing maltodextrin at 1:1; 1:2, and 1:3 of AC:EA showed longer induction times than the control sample, which was explained by an encapsulation improvement caused by this carbohydrate. However, although this EA cannot interact directly with the EO, maltodextrin can interact with alginate chains via hydrogen bonds between hydroxyl groups as sucrose and trehalose.[Citation43] This interaction possibly promotes an increase of wall density by filling voids between alginate chains, reducing oxygen permeation across the microcapsule.[Citation14] It is important to consider that the molecular weight profile of the carrier matrix is one of the key determinants for the oxidative stability of the encapsulated oils, since it affects the diffusion of small molecules such as oxygen into the particle wall.[Citation14]
Figure 4. Induction time or induction period of emulsions with lemongrass essential oil (LEM), and maltodextrin (MD) or Capsul® (CAP), as encapsulating agents, using LEM as reference. Bars indicate standard deviation of triplicates




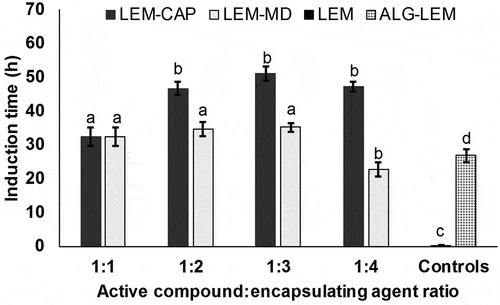
Conclusion
All studied emulsions presented inhibition of bacteria growth, regardless of the type and concentration of the components used (p > .05), indicating that antimicrobial emulsions were development in this study. This result was principally attributed to the high concentration (double of minimum inhibitory concentration) of antimicrobial compounds used (lemongrass essential oil and citral oil). However, some physical properties were affected, such as physical stability, color difference, and oxidative stability, regardless of the AC used. Here, samples with Capsul® showed better oxidative stability, but lower physical stability than samples with maltodextrin. Although some emulsion physical properties (droplet size, polydispersity and flow parameters) did not change by the type and concentration of encapsulating agents, a selection of the encapsulation agent is critical considering that physical properties are so important for a successful additive development. It is important to note that no significant differences (p > .05) on physical and antimicrobial properties were observed between LEM and citral oil. This indicates that the principal active compound of the lemongrass essential oil is responsible for antimicrobial activity. Therefore, both oils can be used interchangeably to obtain the same effect, but the cost of citral oil is higher than LEM, which must be considered in order to obtain a food additive. Finally, the studied emulsions could be used for the development of natural antimicrobial additives. This study has possible industrial applications due to the actual consumer’s request for natural antimicrobial additives instead of synthetic preservatives for food products. The selection of formulation and performance is critical to their effectiveness. Antimicrobial emulsions can be used to be applied directly or to generate powders additives with active properties to increase the shelf life of food matrices. Therefore, both industry and consumers can be beneficiaries from these results.
Acknowledgments
Authors acknowledge financial support by Conicyt Chile [Fondecyt Project 1160463 and Alarcón PhD Grant 21190697], Dicyt-VRIDEI from University of Santiago de Chile [Proyecto Fortalecimiento Usach USA1799_MS172228 and MECESUP USACH USA1899]. J. Alarcón-Moyano collected test data, interpreted the results, and drafted the manuscript. S. Matiacevich is the main researcher, responsible for financial support, design of the study, interpretation of results, and revision of the drafted manuscript. The authors declare that they have no conflict of interest.
Disclosure statement
No potential conflict of interest was reported by the authors.
Additional information
Funding
References
- Salvia-Trujillo, L.; Rojas-Graü, A.; Soliva-Fortuny, R.; Martín-Belloso, O. Impact of Microfluidization or Ultrasound Processing on the Antimicrobial Activity against Escherichia coli of Lemongrass Oil-loaded Nanoemulsions. Food Control. 2014, 37(1), 292–297. DOI: 10.1016/j.foodcont.2013.09.015.
- Chouhan, S.; Sharma, K.; Guleria, S. Antimicrobial Activity of Some Essential Oils—Present Status and Future Perspectives. Medicines. 2017, 4(3). DOI: 10.3390/medicines4030058.
- Moraes-Lovison, M.; Marostegan, L. F. P.; Peres, M. S.; Menezes, I. F.; Ghiraldi, M.; Rodrigues, R. A. F.; Fernandes, A. M.; Pinho, S. C. Nanoemulsions Encapsulating Oregano Essential Oil: Production, Stability, Antibacterial Activity and Incorporation in Chicken Pâté. LWT - Food Sci. Technol. 2017, 77, 233–240. DOI: 10.1016/j.lwt.2016.11.061.
- Adukwu, E. C.; Bowles, M.; Edwards-Jones, V.; Heather, B. Antimicrobial Activity, Cytotoxicity and Chemical Analysis of Lemongrass Essential Oil (Cymbopogon flexuosus) and Pure Citral. Appl. Microbiol. Biotechnol. 2016, 100(22), 9619–9627. DOI: 10.1007/s00253-016-7807-y.
- Saddiq, A. A.; Khayyat, S. A. Chemical and Antimicrobial Studies of Monoterpene: Citral. Pestic. Biochem. Physiol. 2010, 98(1), 89–93. DOI: 10.1016/j.pestbp.2010.05.004.
- Paranagama, P. A.; Abeysekera, K. H. T.; Abeywickrama, K.; Nugaliyadde, L. Fungicidal and Anti-aflatoxigenic Effects of the Essential Oil of Cymbopogon citratus (DC.) Stapf (Lemongrass) against Aspergillus flavus. Lett Appl. Microbiol. 2003, 37(1), 86–90. DOI: 10.1046/j.1472-765X.2003.01351.x.
- Lu, W. C.; Huang, D. W.; Wang, C. C.; Yeh, C. H.; Tsai, J. C.; Huang, Y. T.; Li, P. H. Preparation, Characterization, and Antimicrobial Activity of Nanoemulsions Incorporating Citral Essential Oil. J. Food Drug Anal. 2018, 26(1), 82–89. DOI: 10.1016/j.jfda.2016.12.018.
- Food and Drug Administration. Electronic Code of Federal Regulations Food and Drugs. Substances Generally Recognized as Safe. Chapter 1, Title 21, Subchapter B. Part 182, 2018. https://www.ecfr.gov/cgi-bin/text-idx?SID=e956d645a8b4e6b3e34e4e5d1b690209&mc=true&node=pt21.3.182&rgn=div5 (accessed Aug 29, 2018).
- Prakash, B.; Kujur, A.; Yadav, A.; Kumar, A.; Singh, P. P.; Dubey, N. K. Nanoencapsulation: An Efficient Technology to Boost the Antimicrobial Potential of Plant Essential Oils in Food System. Food Control. 2018, 89, 1–11. DOI: 10.1016/j.foodcont.2018.01.018.
- Arancibia, C.; Navarro-Lisboa, R.; Zúñiga, R. N.; Matiacevich, S. Application of CMC as Thickener on Nanoemulsions Based on Olive Oil: Physical Properties and Stability. Int. J. Polym. Sci. 2016, 2016, 1–10. DOI: 10.1155/2016/6280581.
- Alarcón-Moyano, J. K.; Bustos, R.; Herrera, M. L.; Matiacevich, S. Alginate Edible Films Containing Microencapsulated Lemongrass Oil or Citral: Effect of Encapsulating Agent and Storage Time on Physical and Antimicrobial Properties. J. Food Sci. Technol. 2017, 54(9), 2878–2889. DOI: 10.1007/s13197-017-2726-1.
- Acevedo-Fani, A.; Soliva-Fortuny, R.; Martín-Belloso, O. Nanostructured Emulsions and nanolaminates for Delivery of Active Ingredients: Improving Food Safety and Functionality. Trends Food Sci. Technol. 2017, 60, 12–22. DOI: 10.1016/j.tifs.2016.10.027.
- McClements, D. J.; Rao, J. Food-grade Nanoemulsions: Formulation, Fabrication, Properties, Performance, Biological Fate, and Potential Toxicity. Crit. Rev. Food Sci. Nutr. 2011, 51(4), 285–330. DOI: 10.1080/10408398.2011.559558.
- Carneiro, H. C. F.; Tonon, R. V.; Grosso, C. R. F.; Hubinger, M. D. Encapsulation Efficiency and Oxidative Stability of Flaxseed Oil Microencapsulated by Spray Drying Using Different Combinations of Wall Materials. J. Food Eng. 2013, 115(4), 443–451. DOI: 10.1016/j.jfoodeng.2012.03.033.
- Purwanti, N.; Zehn, A. S.; Pusfitasari, E. D.; Khalid, N.; Febrianto, E. Y.; Sutrisno-Suro, A.; Kobayashi, I. Emulsion Stability of Clove Oil in Chitosan and Sodium Alginate Matrix. Int. J. Food Prop. 2018, 21(1), 566–581. DOI: 10.1080/10942912.2018.1454946.
- Jafari, S. M.; Assadpoor, E.; He, Y.; Bhandari, B. Encapsulation Efficiency of Food Flavours and Oils during Spray Drying. J. Drying Technol. 2008, 26(7), 816–835. DOI: 10.1080/07373930802135972.
- Fathi, M.; Martín, A.; McClements, D. J. Nanoencapsulation of Food Ingredients Using Carbohydrate Based Delivery Systems. Trends Food Sci. Technol. 2014, 39(1), 18–39. DOI: 10.1016/j.tifs.2014.06.007.
- Fernandes, R. V. B.; Silva, E. K.; Borges, S. V.; de Oliveira, C. R.; Yoshida, M. I.; da Silva, Y. F.; Lourenço, E.; Machado, V.; Alvarenga, D. Proposing Novel Encapsulating Matrices for Spray-dried Ginger Essential Oil from the Whey Protein Isolate-inulin/maltodextrin Blends. Food Bioprocess. Technol. 2017, 10(1), 115–121. DOI: 10.1007/s11947-016-1803-1.
- Aburto, L.; Tavares, D.; Martucci, E. Microencapsulação De Óleo Essencial de Laranja. Ciência E Tecnologia De Alimentos. 1998, 18(1), 45–48. DOI: 10.1590/S0101-20611998000100010.
- Mabille, C.; Leal-Calderon, F.; Bibette, J.; Schmitt, V. Monodisperse Fragmentation in Emulsions: Mechanisms and Kinetics. Europhys. Lett. 2003, 61(5), 708–714. DOI: 10.1209/epl/i2003-00133-6.
- Matiacevich, S.; Silva, P.; Osorio, F.; Enrione, J. Color in Food: Technological and Psychophysical Aspects. In Evaluation of Blueberry Color during Storage Using Image Analysis; Caivano, J. L., Buera, M. P., Eds.; CRC Press: Boca Raton, 2012; pp 211–218.
- Luo, M. R.; Cui, G.; Rigg, B. The Development of the CIE 2000 Colour Difference Formula: CIEDE2000. Color Res. Appl. 2001, 26(5), 340–350. DOI: 10.1002/col.1049.
- Salvia-Trujillo, L.; Rojas-Graü, M. A.; Soliva-Fortuny, R.; Martin-Belloso, O. Effect of Processing Parameters on Physicochemical Characteristics of Microfluidized Lemongrass Essential Oil-alginate Nanoemulsions. Food Hydrocolloids. 2013, 30(1), 401–407. DOI: 10.1016/j.foodhyd.2012.07.004.
- Center for Disease Control and Prevention (CDCP), & World Health Organization (WHO). Manual for the Laboratory Identification and Antimicrobial Susceptibility Testing of Bacterial Pathogens of Public Health Importance in the Developing World; World Health Organization: Atlanta GA, 2003; pp 209–214.
- Acosta, E. Bioavailability of Nanoparticles in Nutrient and Nutraceutical Delivery. Curr. Opin. Colloid Interface Sci. 2009, 14(1), 3–15. DOI: 10.1016/j.cocis.2008.01.002.
- Klinkesorn, U.; Sophanodora, P.; Chinachoti, P.; Decker, E. A.; McClements, D. J. Encapsulation of Emulsified Tuna Oil in Two-layered Interfacial Membranes Prepared Using Electrostatic Layer-by-layer Deposition. Food Hydrocolloids. 2005, 19(6), 1044–1053. DOI: 10.1016/j.foodhyd.2005.01.006.
- Sharif, H. R.; Williams, P. A.; Sharif, M. K.; Khan, M. A.; Majeed, H.; Safdar, W.; Shamoon, M.; Shoaib, M.; Haider, J.; Zhong, F. Influence of OSA-starch on the Physico Chemical Characteristics of Flax Seed Oil-eugenol Nanoemulsions. Food Hydrocolloids. 2017, 66, 365–377. DOI: 10.1016/j.foodhyd.2016.12.002.
- Silva, H. D.; Cerqueira, M. A.; Vicente, A. A. Nanoemulsions for Food Applications: Development and Characterization. Food Bioprocess. Technol. 2012, 5(3), 854–867. DOI: 10.1007/s11947-013-1094-8.
- McClements, D. J. Food Emulsions. Principles, Practices and Techniques, 3rd ed.; CRC Press: Boca Raton, 2016.
- Li, Y.; Ch., W.; Wu, T.; Wang, L.; Chen, S.; Ding, T.; Hu, Y. Preparation and Characterization of Citrus Essential Oils Loaded in Chitosan Microcapsules by Using Different Emulsifiers. J. Food Eng. 2018, 217, 108–114. DOI: 10.1016/j.jfoodeng.2017.08.026.
- Dokić, L.; Krstonošić, V.; Nikolić, I. Physicochemical Characteristics and Stability of Oil-in-water Emulsions Stabilized by OSA Starch. Food Hydrocolloids. 2012, 29(1), 185–192. DOI: 10.1016/j.foodhyd.2012.02.008.
- Li, D.; Li, L.; Xiao, N.; Li, M.; Xie, X. Physical Properties of Oil-in-water Nanoemulsions Stabilized by OSA-modified Starch for the Encapsulation of Lycopene. Colloids Surf. A. 2018, 552, 59–66. DOI: 10.1016/j.colsurfa.2018.04.055.
- Sweedman, M. C.; Tizzotti, M. J.; Schäfer, C.; Gilbert, R. G. Structure and Physicochemical Properties of Octenyl Succinic Anhydride Modified Starches: A Review. Carbohydr. Polym. 2013, 92(1), 905–920. DOI: 10.1016/j.carbpol.2012.09.040.
- Miao, M.; Li, R.; Jiang, B.; Cui, S. W.; Zhang, T.; Jin, Z. Structure and Physicochemical Properties of Octenyl Succinic Esters of Sugary Maize Soluble Starch and Waxy maize starch. Food Chem. 2014, 151, 154–160. DOI: 10.1016/j.foodchem.2013.11.043.
- Ong, W. D.; Tey, B. T.; Quek, S. Y.; Tang, S. Y.; Chan, E. S. Alginate-based Emulsion Template Containing High Oil Loading Stabilized by Nonionic Surfactants. J. Food Sci. 2015, 80(1), 93–100. DOI: 10.1111/1750-3841.12729.
- Ravichandran, K.; Palaniraj, R.; Saw, N. M. M. T.; Gabr, A. M. M.; Ahmed, A. R.; Knorr, D.; Smetanska, I. Effects of Different Encapsulation Agents and Drying Process on Stability of Betalains Extract. J. Food Sci. Technol. 2014, 51(9), 2216–2221. DOI: 10.1007/s13197-012-0728-6.
- Idrees, M.; Nasir, S.; Naeem, M.; Aftab, T.; Masroor, M.; Khan, A.; Varshney, M. L. Gamma Irradiated Sodium Alginate Induced Modulation of Phosphoenolpyruvate Carboxylase and Production of Essential Oil and Citral Content of Lemongrass. Ind. Crops Prod. 2012, 40, 62–68. DOI: 10.1016/j.indcrop.2012.02.024.
- McClements, D. J.;. Colloidal Basis of Emulsion Color. Curr. Opin. Colloid Interface Sci. 2002, 7(5–6), 451–455. DOI: 10.1016/S1359-0294(02)00075-4.
- Camino, N. A.; Pilosof, A. M. R. Hydroxypropylmethylcellulose at the Oil-water Interface. Part II. Submicron-emulsions as Affected by pH. Food Hydrocolloids. 2011, 25(5), 1051–1062. DOI: 10.1016/j.foodhyd.2010.09.026.
- Chang, Y.; McLandsborough, L.; McClements, D. J. Fabrication, Stability and Efficacy of Dual-component Antimicrobial Nanoemulsions; Essential Oil (Thyme Oil) and Cationic Surfactant (Lauric Arginate). Food Chem. 2015, 172, 298–304. DOI: 10.1016/j.foodchem.2014.09.081.
- Burt, S. Essential Oils: Their Antibacterial Properties and Potential Applications in Foods-a Review. Int. J. Food Microbiol. 2004, 94(3), 223–253. DOI: 10.1016/j.ijfoodmicro.2004.03.022.
- Weiss, J.; Loeffler, M.; Terjung, N. The Antimicrobial Paradox: Why Preservatives Lose Activity in Foods. Curr. Opin. Food Sci. 2015, 4, 69–75. DOI: 10.1016/j.cofs.2015.05.008.
- Russ, N.; Zielbauer, B. I.; Vilgis, T. A. Impact of Sucrose and Trehalose on Different Agarose-hydrocolloid Systems. Food Hydrocolloids. 2014, 41, 44–52. DOI: 10.1016/j.foodhyd.2014.03.020.