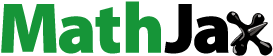
ABSTRACT
Sulfur dioxide (SO2) is an efficient additive that is used during winemaking to prevent microbial contamination as well as the oxidation and color changes caused by enzymatic and nonenzymatic reactions. However, this compound can cause allergic reactions to humans. In this study, α-pinene, a monomeric compound derived from fruits, was used to be an alternative for sulfur dioxide in red wine fermentation. Three concentrations of α-pinene, 0.125%, 0.25%, or 0.5%, were used. The red wine without additive or SO2 was used as controls. No significant differences were found in total soluble solids, pH values, titratable acidity, and ethanol content (n = 3, p < .05) though lower ethanol content was observed in the group at 0.5% α-pinene. The antibacterial results were the same between adding α-pinene and SO2. In the α-pinene group, significantly higher L and a values as well as transmittance were obtained than in the control and SO2 groups. Higher free radical scavenging ability was also obtained in the groups of α-pinene. However, the group with the highest concentration, 0.5%, showed negative effects to ethanol production and sensory performance. Better physicochemical and sensory characteristics were obtained in the groups at 0.125% or 0.25% of α-pinene, particularly, when 0.125% of α-pinene was added. Thus, α-pinene at 0.125% should be the optimal concentration for a potential alternative for SO2 in winemaking.
Introduction
Wine fermentation is a complex biochemical process based on the microbial conversion of sugar to ethanol. Carbon dioxide, fermentation by-products, and various microbes found on the surface of grape skin and the microbiota associated with winery surfaces participate in natural wine fermentation.[Citation1] The involvement of microorganisms is essential for the winemaking process and for determining wine quality. Sulfur dioxide (SO2) is a highly versatile and efficient additive included during winemaking to prevent microbial contamination, oxidation, and color changes related to undesirable enzymatic and nonenzymatic reactions.[Citation2] Although this compound inhibits the development of yeast, lactic acid bacteria, and acetic acid bacteria during winemaking, it has been found to be associated with allergic reactions or intolerance in some consumers. Most nonasthmatic individuals can tolerate up to 5 ppm, whereas sulfite-sensitive individuals react negatively to its ingestion and may experience a range of symptoms, including dermatitis, urticaria, angioedema, abdominal pain, diarrhea, bronchoconstriction, and anaphylaxis[Citation3], which have been associated with the presence of sulfite in wine. Forbidding the use of SO2 as an antimicrobial agent without an alternative would increase the risk of wine spoilage by yeast and bacteria.[Citation4] Therefore, there is a great deal of interest in finding alternatives to SO2 in winemaking.
Raposo et al.[Citation5] demonstrated the use of a grapevine shoot extract as a sustainable alternative to SO2 and were able to preserve wine composition without loss of quality. Additionally, the use of lysozyme has been proposed to control malolactic fermentation in winemaking to support sulfur dioxide substitution.[Citation5] Recently, some emerging technologies, also called green technologies, have been proposed as alternatives to SO2. Pulsed electric field, ultrasound, high pressure, and ultraviolet light have been tested in wine.[Citation6] Santos et al.[Citation7] demonstrated that moderate high hydrostatic pressure treatments, i.e., 425 and 500 MPa, for 5 min affect the long-term physiochemical characteristics of red and white wines, namely increasing orange-red color, reducing antioxidant activity, and enhancing total phenolic content. These studies have demonstrated the importance of preparing SO2-free wine for the winemaking industry.
According to Zacchino et al.[Citation8], of 100 papers reviewed on natural products that showed the antibacterial inhibitory capacity of adjuvants, approximately 73% dealt with polyphenols (48%) and terpenoids (25%). Terpenes are a class of compounds found as constituents of essential oils; these compounds are mostly hydrocarbons, which are classified according to the number of isoprene units.[Citation9] The simplest terpenes are monoterpenes that contain two isoprene molecules. Monoterpenes, such as ocimene, α-pinene, and limonene, are volatile molecules that evaporate quickly and are called top notes by the perfume industry. Some monoterpenes, such as α-pinene and limonene, exhibit antitumor properties.[Citation10,Citation11] The same amount of α-pinene with different enantiomeric compositions can have diverse antimicrobial potential owing to different specific interactions with other chemical compounds of essential oils.[Citation12] Moreover, grape skin contains a large number of terpenoids, which are extracted during fermentation.[Citation13] Among the specific aroma sources, terpenes are the main components of wine-specific aromas owing to their high concentration and low aroma threshold.[Citation14] Terpenes, a source of volatile aromas in wine, are present in the form of free volatiles or in combination, usually in the form of glycosides[Citation15], whereas in grapes, they are synthesized during ripening and are subject to the qualitative and quantitative effects of variety, soil, climate, and viticulture, strongly affecting the aromatic content of the wine.
Comprehensive studies have demonstrated the development of alternatives to SO2; however, these studies have not revealed other methods to effectively replace SO2 in winemaking. α-Pinene is one of the main components in the terpene family and is widely present in a variety of plant aroma compounds, including grapes and blueberries. Moreover, α-pinene shows antibacterial and antioxidant properties.[Citation16] Therefore, in this study, we aimed to evaluate the applicability of additional commercial α-pinene as an alternative to SO2 in winemaking, which was studied using three different doses of α-pinene. Quality and sensory parameters were also analyzed.
Materials and methods
Chemicals
Black queen grapes (Vitis vinifera × V. labrusca) harvested in 2018 and the yeast, Saccharomyces cerevisiae (BC103), were supplied by Song Heh Farm Produce (Taiwan). Ethanol (95%) and isopropanol were supplied by Echo Chemical Co., Ltd. (Taiwan). Acetone was supplied by Seedchem (Australia). H2SO4 was supplied by Aencore (Australia). Plate Count Agar (PCA) was supplied by Oxoid (UK). Sucrose and K2S2O5 were supplied by Wang Laichzng Enterprise Co., Ltd. (Taiwan). α-Pinene (≥99%), Folin-Ciocalteu phenol reagent, and ascorbic acid were supplied by Sigma (USA). 1,1-Diphenyl-2-picrylhydrazyl radical 2,2-diphenyl-1-(2,4,6-trinitrophenyl) hydrazyl was supplied by Alfa Aesar (USA). NaCl, Na2HPO4, NaOH, sodium tetraborate, and Na2CO3 were supplied by Showa Chemical Industry Co., Ltd. (Japan). KH2PO4 was supplied by Katayama Chemical (Japan).
Winemaking
Fresh black queen grapes grown in the Houli area (Longitude: 120E42’00”, Latitude:24N18’00”) (Taichung city, Taiwan) were harvested manually in July 2018 by Song Hen Farm Produce (Taichung, Taiwan) and transported to laboratory on the same day of harvest. Based on the protocols of Song Hen Farm Produce, around 25 kg of black queen grapes were harvested, destemmed, and crushed. The initial Brix was ~12 degree, then sucrose was added to the grape juice to increase reach ~25 °Brix. The yeast, Saccharomyces cerevisiae (BC103, France), supplied by Song Hen Farm Produce was activated by adding into 25 mL warm water at 40°C to 45°C at the concentration of 0.25 g/L. After activation for 15 min, the yeast suspension was added into the juice and fermented at 25 ± 2°C for 14 d. There are five different groups: (1) control group, without added SO2; (2) SO2 group, (SO2 was added at 55 ppm); (3) 0.125% α-pinene group; (4) 0.25% α-pinene group; and (5) 0.5% α-pinene group. Three bottles were used for each group and each bottle contained 1 L. After fermentation, the wine was filtered. Suitable amount for different tests was sampled and centrifuged at 13,000 g for 20 min at 4°C to determine the characteristics in physicochemical properties.
Microbial analysis
Since antibacterial effects were confirmed by the previous study (Wang et al., 2019)[Citation16], only the standard total plate count (TPC) was conducted to monitor the microbiological quality of the white wine samples. The pour plate method was adopted for this TPC analyses[Citation17] which were conducted before and the end of fermentation.
Physicochemical analysis of the wine (total soluble solids, pH, titratable acidity, and transmittance)
The physicochemical analysis of the wine including total soluble solids (TSS), specific gravity, pH value, titratable acidity, pectin, degree of ester (DE), phenolic acid, ethanol, and color (L, a, and b) values, was determined. A hand-held refractometer (Atago, Tokyo, Japan) was used to determine the TSS (as °Brix) of wine.[Citation17] The refractometer was calibrated with distilled water each time before use. The specific gravity was determined by hydrometer. Changes in pH value of wines after fermentation were determined by a pH meter (pH/ION METER SP-2500) at room temperature (25 ± 2°C). Distilled water (25 mL) was added to wine (5 mL), and the pH value was adjusted to 8.1 by adding 0.1 N NaOH. The volume of NaOH used was recorded and calculated as percent tartaric acid. Tartaric acid was used as a standard.[Citation17] The percent tartaric acid was calculated as follows: TA (%) = (V × 0.1 M NaOH × 0.075 × 100)/M (V: mls NaOH used; 0.075: milliequivalent factor; M: grams of sample). The transmittance was determined by spectrophotometer (HITACHI U-2000, JAPAN).
Pectin
Pectin was analyzed in wines after fermentation according to the methods of Hou et al.[Citation18] Precooled H2SO4 (2 mL) and distilled water (15 mL) were slowly added to 5 mg alcohol insoluble solid of wines. The mixture was filtered with Whatman number 1 filter paper to obtain pectin solution after magnetic stirring for 1 h in an ice bath and then heated in a boiling water bath for 5 min. After cooling, the reaction mixture was mixed well with 0.05 mL of 0.15% m-hydroxydiphenyl solution in 0.5% NaOH and then allowed to rest for 5 min. Absorbance at 520 nm was recorded. d-Galacturonic acid was used to construct the standard curve for calculation in the samples.[Citation18]
Dextrose equivalent (DE)
The DE of commercial pectin samples was determined by the titration method of Warnakulasuriya et al.[Citation18] Briefly, 5 g pectin was mixed with 5 mL HCl (2.7 M), and 100 mL of 60% (v/v) ethanol was added and stirred (900 rpm) for 10 min at room temperature. The mixture was further washed with the same solvent mixture (6 × 15 mL) and then with 60% ethanol. The mixture was then rinsed with 20 mL anhydrous ethanol and oven dried for 1 h at 105°C. To the dried pectin sample (0.5 g) in a beaker, we added 2 mL ethanol and 100 mL distilled water. The resulting solution was titrated against 0.1 M NaOH using phenolphthalein indicator. The initial titer value was recorded as V1 (mL). To the titrated reaction mixture, a 20.0-mL aliquot of 0.5 M NaOH was added, and the mixture was then stirred vigorously for 15 min. Subsequently, 20.0 mL of 0.5 M HCl acid was added and stirred until the pink color disappeared. The resulting solution after the addition of 0.5 M HCl was then titrated against 0.1 N NaOH to obtain the titer value Vs (mL). DE was calculated using Eq. (1)[Citation19]:
Total phenolic acids
Gallic acid (dissolved in methanol at concentrations ranging from 0.125 to 2 mg/mL) was used as a standard. Briefly, grape wine was diluted to 1 mg/mL with methanol, and 30 μL of each diluted extract with 150 μL Folin-Ciocalteu reagent was transferred to a 96-well plate. An aqueous 7.5% Na2CO3 solution (120 μL) was added, and the absorbance of the solution was measured at 765 nm after 1 h of incubation.[Citation20]
Ethanol content
A gas chromatography system with a flame ionization detector was employed. The temperature was set at 180°C, the split ratio was set as 1:50, and the injection pore temperature was set at 180°C. The column temperature elevation condition was an initial temperature of 40°C that was maintained for 3 min before the temperature was increased to 180°C at a rate of 40°C/min; the final temperature was maintained for 1 min.[Citation18]
Wine color
The measurement of wine color was carried out using an SA2000 spectrophotometer (NDK NIPPON DENSHOKU, Japan). The L, a, and b parameters were determined according to the regulations of the International Commission on Illumination: red/green color (a∗) and yellow/blue color (b∗) components and luminosity (L∗).[Citation21] Color differences (ΔE) were calculated as the Euclidean distance between two points in the three-dimensional space defined by L, a, and b, as follows:
2,2-diphenyl-1-picrylhydrazyl (DPPH) free radical scavenging activity
The free radical scavenging activity was determined using the DPPH method. The hydrogen atom or electron donation ability of the sample was measured using a methanol solution of DPPH. A 160-µL aliquot of sample solution was mixed with 20 µL DPPH solution (180 μM) in methanol in a 96-well plate. DPPH solution was used as the blank, and ascorbic acid was used as the positive control. After 40 min of incubation in the dark at room temperature (25 ± 2°C), the samples were observed using a 96-well plate reader (HT 340 ELISA reader; Bio Tec) to monitor the decrease in absorbance at 517 nm. The free radical scavenging activity was expressed as the percent inhibition of DPPH and was calculated using the following formula:
Scanning electron microscopy (SEM) of the grape must
In the freeze drying process[Citation22], grape pomace was frozen at −20°C and then lyophilized using a condenser temperature of −110°C and a pressure of 10 Pa. The working pressure was lowered using a rotary pump below the triple point of water (607 Pa), to allow ice sublimation. For SEM micrographs, an FEI Quanta 200 (FEI, USA) was used with a 5-mm working distance and accelerating voltage of 5–10 kV in secondary electron detection mode.[Citation23]
Sensory analysis
Fifteen panelists were standardized by tasting with the commercial red wine provided by the Song Hen Farm Produce. The samples were evaluated using seven descriptors including color, aroma, flavor, continuing taste (long-lasting), the balance taste of sweetness, acidity and bitterness, the complexity of wine flavor and aroma, and overall quality. Totally, three sessions were taken place before formal sensory evaluation. The commercial red wine was used as a standard and tasted simultaneously throughout the study. The protocols were conducted in accordance with the implementation of the sensory evaluation guidelines.[Citation24] The sample red wine was stored at 4°C until tasting. Wine was placed in a clear glass cup under the regular fluorescent lights. The serving temperature of the wine samples was between 14°C and 18°C and the room temperature was ~25°C. The descriptors were scored on a continuous scale from 1 to 9 (1 = minimum intensity, 9 = maximum intensity). One cup (about 20 mL) was tasted for each group and the panelists rinsed by blank drinking water twice between each group.
Statistical analysis
Three replicates samples were conducted for each group and the tests were repeated three times. Totally, nine replicates were analyzed. Data were expressed as the mean ± standard deviation and evaluated using one-way analysis of variance to determine the significance of differences among the mean values (least-squares difference test). Statistical analysis was performed using SPSS for Windows (v.14.0; SPSS Inc., Chicago, IL, USA), and significance was determined at p < .05.
Results and discussion
Examination of the bacteriostatic ability of α-pinene during fermentation from bacterial counts before and after fermentation
Bacterial counts in wine were determined before and after the fermentation. As shown in , the total plate count of the puree before fermentation was 3.98 log10 CFU/mL, demonstrating that there was a risk of bacterial contamination in residual bacterial counts if SO2 was not used. During the fermentation, the inoculated yeast gradually became the dominant organism. After fermentation, no microorganism was detected for all treated groups including SO2 and all concentrations of α-pinene. In the control group, bacterial population was 2.46 log10 CFU/mL which was about 1 log reduction before fermentation. The results showed that SO2 and all concentrations of α-pinene were effectively inhibited the growth of non-desired bacteria. In the contrast, the control group still had a bacterial population at 2.46 log10CFU/mL which could potentially cause non-desired flavor and physicochemical characteristics. These suggested that α-pinene possessed the similar ability of inhibiting bacterial growth with SO2. Therefore, adding α-pinene could effectively prevent the risk of bacterial contamination during fermentation and storage.
Table 1. Effects of different concentrations of α-pinene on the total plate count (TPC) of black queen wine
Effects of α-pinene to the physiochemical characteristics of the black queen wine
The quality characteristics of the black queen wine were analyzed. All results are shown in , which includes the specific gravity, TSS, ethanol, titratable acidity, total phenol, DE, and pH values of different treatment groups. Specific gravity is one of the markers to assess fermentation results and gradually decreases as fermentation proceeding. As shown in , the specific gravity of all groups approached 1.00, and there were no significant differences among groups, indicating that all groups achieved the similar degree of fermentation.
Table 2. Effects of different concentrations of α-pinene on the chemical composition of Black Queen Muscat wine
Ethanol concentration is usually around 12–14% in wine.[Citation25] In this study, the black queen grapes harvested in Taiwan in 2018 had a glucose content of 12°Brix, and the sugar content was adjusted to 25°Brix. As shown in , there was no significant difference between different groups for the ethanol contents though ethanol content gradually decreased while the concentrations of α-pinene was increased. In addition, the residual sugar content in the groups with the higher concentrations of α-pinene (0.25%, 0.5%) significantly higher than those in other groups. These results suggested that α-pinene at 0.25% and 0.5% could be too great that not only inhibited the growth of non-desired bacteria but the yeast as well. In addition, the residual sugar contents in the groups with high concentrations of α-pinene (0.25%, 0.5%) were higher than the control, SO2, and 0.125% α-pinene groups. The higher contents of sugar residue also indicated that the concentrations of α-pinene at 0.25% and 0.5% could negatively affected the conversion of sugar to alcohol. Therefore, 0.125% should be the suitable concentration to achieve the balance of antimicrobial ability and fermentation.
Acids are important components of wine and affect physiochemical and sensory characteristics of wine. As shown in , the pH values of all groups of wine were around 3.5, which was in the range of commercial products. In addition, there was not significant difference between all groups (p > .05). These results indicated that adding α-pinene did not alter the pH of wines.
Pectin could cause the high viscosity and turbidity of wine. The degree of pectin esterification or methylation is defined as the percentage of methylated galacturonic acid and is known as DE. In this study, addition of α-pinene significantly decrease DE than those in the control and SO2 groups. Higher concentration of α-pinene caused higher decrease of DE. However, even at the lowest concentration, 0.125%, the DE was still significantly lower than those in the control and SO2 groups. The mechanism of DE decrease could be the interference of α-pinene in pectinase activity and further study is needed.
Color is an essential quality in winemaking and provides useful information regarding the concentration of phenols and the oxidation tendency in wine.[Citation5] As shown in , adding α-pinene significantly increased the L and a values of wines and not in b values. Thus, the wine adding with α-pinene showed higher brightness and reddish even at the lowest concentration. Therefore, adding α-pinene improved the wine appearance, which offered the greater perception for consumers. Oxidative browning is a well-known problem in winemaking, and SO2 has been used as an antioxidant to control wine browning.[Citation26] In our study, the increase of the brightness and reddish demonstrated that α-pinene potentially inhibited the browning reaction in wine and be an effective alternative for SO2.
Table 3. Effects of different concentrations of α-pinene on the color of black queen wine
High transmittance means high transparency in wine and is the key factor for the clarity of wine. As shown in , the transmittance of the five groups ranged from 50 to 60 and adding α-pinene at all concentrations showed higher results than the control and SO2 groups. When adding α-pinene at the highest concentration (0.5%), lower transparency values than the 0.125% and 0.25% were obtained. This could be due to when greater DE decreased, small-molecule pectin could link together in wine and caused the decrease of transparency. However, adding α-pinene generally increased transparency of wine. These results were correlated the previous results, in which the lower DE, higher L and a values were obtained in the α-pinene groups.
Effects of α-pinene addition on other sensory characteristics in black queen wine
As shown in , the group added the 0.125% and 0.25% demonstrated similar performance for sensory characteristics with the SO2 groups. Overall, sensory performance decreased in the following order: control > 0.125% α-pinene > 0.25% α-pinene > SO2 > 0.5% α-pinene group. In terms of overall performance, the 0.125% and 0.5% α-pinene group had the highest and lowest score in all treatment groups. As a terpene compound, α-pinene possesses strong odor, which negatively interfere the wine sensory even at a very low concentration. The GS-MS results also showed that residual α-pinene was detected in the group with 0.5% α-pinene but not in the groups with 0.125% and 0.5% α-pinene after fermentation (Table S1). Thus, the low scores of sensory performance in the group with 0.5% α-pinene should be due to the existence of residual α-pinene. In addition, α-pinene was degraded during fermentation if low concentrations such as 0.125% and 0.25% were used. Therefore, adding α-pinene at low concentrations could be commercially practical since it offered better or similar sensory performance with adding SO2.
Effects of α-pinene addition on free radical scavenging abilities in black queen wine
Wines contain large amounts of anthocyanin and other phenolic compounds, which offer strong antioxidative activities. As shown in , the addition of α-pinene enhanced scavenging abilities significantly. The scavenging abilities in all α-pinene groups were significantly higher than control and SO2 groups (p < .05). In addition, the free radical scavenging abilities were correlated with the concentrations of α-pinene. Higher abilities were obtained at the higher concentrations of α-pinene. In the contrast, significant lower total phenolic contents were obtained in the groups of α-pinene than control and SO2 groups and lower phenolic contents were obtained in the groups with higher concentrations of α-pinene (). Therefore, α-pinene addition affected the release of total phenolic substances during winemaking. However, a previous study in our laboratory demonstrated that α-pinene possessed a strong DPPH free radical scavenging activity (half-maximal inhibitory concentration value = 12.57 ± 0.18 mg/mL) and reducing power (213.7 ± 5.27 μg/mL of l-ascorbic acid equivalents).[Citation16] This could explain that higher scavenging abilities still obtained in the groups of α-pinene though lower phenolic contents were observed in the groups.
Figure 3. Effects of adding different concentrations of α-pinene on the free radical scavenging ability of black queen wine. Data are averages of at least three independent experiments. One-way ANOVA with LSD tests; values with different letters within the same group are significantly different (p < .05)
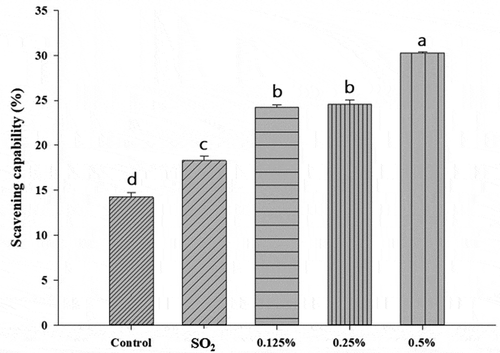
Effects of α-pinene addition on SEM images of black queen grape pomace
SEM was used to observe the images of grape pomace after fermentation and the degradation of pomace after fermentation. As shown in , (a) Control, (b) SO2 (55 ppm), (c) 0.125% α-pinene, (d) 0.25% α-pinene, (e) 0.5% α-pinene, respective. The pomace was not completely degraded in the control group, whereas degradation was complete in the SO2 and α-pinene groups. Particularly, the degradation of the groups with 0.125% and 0.25% of α-pinene was more completed than the group with 0.5% α-pinene. These results were corresponded with the higher L and a values as well as transparency in groups with 0.125% and 0.25% of α-pinene than in the group of 0.5% α-pinene.
Conclusion
Results showed that adding α-pinene effectively inhibited microbial growth and reduced the total plate count. Higher transparency and free radical scavenging ability were also obtained in the groups of α-pinene. However, the highest concentration, 0.5%, showed negative effects to ethanol production and sensory performance. Therefore, adding α-pinene at lower concentration, 0.125% or 0.25%, should be more suitable. Particularly, when the lowest concentration, 0.125%, was added, better physicochemical and sensory characteristics were obtained. Thus, adding α-pinene at 0.125% should be the optimal concentration to be a potential alternative for SO2 in winemaking. Further studies are needed to evaluated the addition of α-pinene in other types of wine winemaking and large quantity production.
Supplemental Material
Download MS Word (21.5 KB)Acknowledgments
The author would thank all those who volunteered for assisting with the study. The authors declare no conflicts of interest.
Supplementary Material
Supplemented data of this article can be accessed publisher’s website.
Additional information
Funding
References
- Pretorius, I. S.; van der Westhuizen, T. J.; Augustyn, O. P. H. Yeast Biodiversity in Vineyards and Wineries and Its Importance to the South African Wine Industry. A Review. S. Afr. J. Enol. Vitic. 2017, 20(2), 10.
- Fazio, T.; Warner, C. R. A Review of Sulphites in Foods: Analytical Methodology and Reported Findings. Food Addit. Contam. 1990, 7(4), 433–454. DOI: 10.1080/02652039009373907.
- Vally, H.; Misso, N. L. A.; Madan, V. Clinical Effects of Sulphite Additives. Clin. Exp. Allergy. 2009, 39(11), 1643–1651. DOI: 10.1111/cea.2009.39.issue-11.
- Guerrero, R. F.; Cantos-Villar, E. Demonstrating the Efficiency of Sulphur Dioxide Replacements in Wine: A Parameter Review. Trends Food Sci. Technol. 2015, 42(1), 27–43. DOI: 10.1016/j.tifs.2014.11.004.
- Bartowsky, E. J.; Costello, P. J.; Villa, A.; Henschke, P. A. The Chemical and Sensorial Effects of Lysozyme Addition to Red and White Wines over Six Months’ Cellar Storage. Aust. J. Grape Wine Res. 2004, 10(2), 143–150. DOI: 10.1111/j.1755-0238.2004.tb00017.x.
- Raposo, R.; Ruiz-Moreno, M. J.; Garde-Cerdán, T.; Puertas, B.; Moreno-Rojas, J. M.; Zafrilla, P.; Gonzalo-Diago, A.; Guerrero, R. F.; Cantos-Villar, E. Replacement of Sulfur Dioxide by Hydroxytyrosol in White Wine: Influence on Both Quality Parameters and Sensory. LWT - Food Sci. Technol. 2016, 65, 214–221. DOI: 10.1016/j.lwt.2015.08.005.
- Santos, M. C.; Nunes, C.; Cappelle, J.; Gonçalves, F. J.; Rodrigues, A.; Saraiva, J. A.; Coimbra, M. A. Effect of High Pressure Treatments on the Physicochemical Properties of a Sulphur Dioxide-free Red Wine. Food Chem. 2013, 141(3), 2558–2566. DOI: 10.1016/j.foodchem.2013.05.022.
- Zacchino, S. A.; Butassi, E.; Liberto, M. D.; Raimondi, M.; Postigo, A.; Sortino, M. Plant Phenolics and Terpenoids as Adjuvants of Antibacterial and Antifungal Drugs. Phytomedicine. 2017, 37, 27–48. DOI: 10.1016/j.phymed.2017.10.018.
- Buckle, J. Chapter 3 - Basic Plant Taxonomy, Basic Essential Oil Chemistry, Extraction, Biosynthesis, and Analysis. In Clinical Aromatherapy, 3rd ed.; Buckle, J., Ed.; Churchill Livingstone: St. Louis, 2015; pp 37–72.
- Rabi, T.; Bishayee, A. D -limonene Sensitizes Docetaxel-induced Cytotoxicity in Human Prostate Cancer Cells: Generation of Reactive Oxygen Species and Induction of Apoptosis. J. Carcinog. 2009, 8, 9. DOI: 10.4103/1477-3163.51368.
- Bhattacharjee, B.; Chatterjee, J. Identification of Proapoptopic, Anti-Inflammatory, Anti-Proliferative, Anti-Invasive and Anti-Angiogenic Targets of Essential Oils in Cardamom by Dual Reverse Virtual Screening and Binding Pose Analysis. Asian Pac. J. Cancer Prev. 2013, 14(6), 3735–3742. DOI: 10.7314/APJCP.2013.14.6.3735.
- Ložienė, K.; Švedienė, J.; Paškevičius, A.; Raudonienė, V.; Sytar, O.; Kosyan, A. Influence of Plant Origin Natural α-pinene with Different Enantiomeric Composition on Bacteria, Yeasts and Fungi. Fitoterapia. 2018, 127, 20–24. DOI: 10.1016/j.fitote.2018.04.013.
- de Torres, C.; Schumacher, R.; Alañón, M. E.; Pérez-Coello, M. S.; Díaz-Maroto, M. C. Freeze-dried Grape Skins By-products to Enhance the Quality of White Wines from Neutral Grape Varieties. Food Res. Int. 2015, 69, 97–105. DOI: 10.1016/j.foodres.2014.12.016.
- Carballeira Lois, L.; Cortés Diéguez, S.; Gil de la Peña, M. L.; Fernández Gómez, E. SPE-GC Determination of Aromatic Compounds in Two Varieties of White Grape during Ripening. Chromatographia. 2001, 53(1), S350–S355. DOI: 10.1007/BF02490355.
- Williams, P. J.; Strauss, C. R.; Wilson, B.; Massy-Westropp, R. A. Use of C18 Reversed-phase Liquid Chromatography for the Isolation of Monoterpene Glycosides and Nor-isoprenoid Precursors from Grape Juice and Wines. J. Chromatogr. A. 1982, 235(2), 471–480. DOI: 10.1016/S0021-9673(00)85911-7.
- Wang, C.-Y.; Chen, Y.-W.; Hou, C.-Y. Antioxidant and Antibacterial Activity of Seven Predominant Terpenoids. Int. J. Food Prop. 2019, 22(1), 230–238. DOI: 10.1080/10942912.2019.1582541.
- Bhat, R. Impact of Ultraviolet Radiation Treatments on the Quality of Freshly Prepared Tomato (Solanum Lycopersicum) Juice. Food Chem. 2016, 213, 635–640. DOI: 10.1016/j.foodchem.2016.06.096.
- Hou, C. Y.; Lin, Y. S.; Wang, Y. T.; Jiang, C. M.; Lin, K. T.; Wu, M. C. Addition of Phenolic Acids on the Reduction of Methanol Content in Wine. J. Food Sci. 2008, 73(5), C432–7. DOI: 10.1111/j.1750-3841.2008.00768.x.
- Warnakulasuriya, S.; Pillai, P. K. S.; Stone, A. K.; Nickerson, M. T. Effect of the Degree of Esterification and Blockiness on the Complex Coacervation of Pea Protein Isolate and Commercial Pectic Polysaccharides. Food Chem. 2018, 264, 180–188. DOI: 10.1016/j.foodchem.2018.05.036.
- Choi, M. Y.; Chai, C.; Park, J. H.; Lim, J.; Lee, J.; Kwon, S. W. Effects of Storage Period and Heat Treatment on Phenolic Compound Composition in Dried Citrus Peels (Chenpi) and Discrimination of Chenpi with Different Storage Periods through Targeted Metabolomic Study Using HPLC-DAD Analysis. J. Pharm. Biomed. Anal. 2011, 54(4), 638–645. DOI: 10.1016/j.jpba.2010.09.036.
- Fernández-Vázquez, R.; Stinco, C. M.; Hernanz, D.; Heredia, F. J.; Vicario, I. M. Colour Training and Colour Differences Thresholds in Orange Juice. Food Qual. Preference. 2013, 30(2), 320–327. DOI: 10.1016/j.foodqual.2013.05.018.
- Yen, G. C.; Duh, P. D. Scavenging Effect of Methanolic Extracts of Peanut Hulls on Free-Radical and Active-Oxygen Species. J. Agric. Food Chem. 1994, 42(3), 629–632. DOI: 10.1021/jf00039a005.
- Pathan, A. K.; Bond, J.; Gaskin, R. E. Sample Preparation for SEM of Plant Surfaces. Mater. Today. 2010, 12, 32–43. DOI: 10.1016/S1369-7021(10)70143-7.
- Golia, S.; Brentari, E.; Carpita, M. Causal Reasoning Applied to Sensory Analysis: The Case of the Italian Wine. Food Qual. Preference. 2017, 59, 97–108. DOI: 10.1016/j.foodqual.2017.02.009.
- Ciani, M.; Capece, A.; Comitini, F.; Canonico, L.; Siesto, G.; Romano, P. Yeast Interactions in Inoculated Wine Fermentation. Front. Microbiol. 2016, 7, 555. DOI: 10.3389/fmicb.2016.00555.
- Li, H.; Guo, A.; Wang, H. Mechanisms of Oxidative Browning of Wine. Food Chem. 2008, 108(1), 1–13. DOI: 10.1016/j.foodchem.2007.10.065.