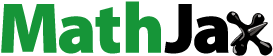
ABSTRACT
The purpose of this study was to provide a new approach to producing a slowly-digestible snack with sensory appeal suitable for people with hyperglycemia. The study was based on naked barley supplemented with other functional components and used low-temperature extrusion technology. The effects of the extrusion temperature (Et = 65–85°C), feed moisture (Fm = 25–35%), and screw speed (Ss = 60–80 rpm) were studied systemically, aiming at minimizing the glycemic index and maximizing the radical scavenging rate while obtaining a good texture and pleasant sensory characteristics. The best response was obtained with an Et of 74.24°C, Fm of 35%, and Ss of 80 rpm. Under these conditions, barley extruded with multiple grains showed a lower degree of gelatinization (70.17%), lower glycemic index (29.37), higher radical scavenging rate (53.66%), and higher sensory evaluation (86) compared to those of extrudates with only naked barley. The present study suggests that the low-temperature extrusion method can effectively produce snacks with a high radical-scavenging rate, slow digestion characteristics, and high market acceptance.
Introduction
The increasing incidence of hyperglycemia is of great concern. It has been suggested that reducing the rate of carbohydrate absorption by lowering the glycemic index (GI) of the diet and balancing the nutrition of each meal could benefit the general population by preventing or delaying the development of diseases that are linked to insulin resistance.[Citation1] Naked barley as a healthy cereal crop for people with hyperglycemia has attracted attention from all walks of life. Wirkijowska et al. reported that (1-3)(1-4)-β-d-glucans in barley can lower blood cholesterol and the GI, and its content in naked barley is higher than in hulled barley.[Citation2] In addition, several bioactive substances found in barley, such as phenolic acids, flavonoids, lignans, tocols, phytosterols, and folate phytochemicals, can exhibit strong antioxidant, antiproliferative, and cholesterol-lowering abilities and potentially lower the risk of certain diseases. Many studies on the application of barley flour in foods have shown that the use of naked barley in food processing is limited due to the coarse taste and the lack of gluten protein. For example, the breads made with barley had lower volume and lower crumb elasticity.[Citation3] Noodles with added barley also showed unpleasant trends of decreased brightness, increased redness, and more visible specking. The proper combination of various grains can not only make foods more nutritionally balanced, but also improve sensory characteristics.[Citation4] It has been reported that products made from barley and dark grains may have better sensory properties,[Citation5] so several dark and healthful grains with similar protective effects for diabetes, including colored kidney beans, quinoa, and black sesame, were chosen for use in this study.
In addition to food raw materials, appropriate processing methods also should be taken into additional consideration in the processing of diabetic diets. Extrusion is a process of simultaneously mixing, heating, and shearing raw food materials to rapidly shape and restructure them. Extrusion has the advantages of high efficiency, low pollution, and low costs. Additionally, it was reported that extrusion can improve the rough texture of grains by converting insoluble dietary fiber into soluble dietary fiber.[Citation6] At present, extruded foods in markets are mainly puffed products characterized by high gelatinization, fast digestion, and crispy texture, which were not suitable for hyperglycemic people. High temperatures and low moisture used for processing puffed foods cause a loss in the nutritional value and the formation of some Maillard reaction products with potentially harmful effects.[Citation7] Conversely, low-temperature extrusion can produce foods with lower degrees of gelatinization while retaining the nutrients and the slow digestion characteristics of the grains, which are suitable characteristics for a diabetic diet.[Citation8] Therefore, exploring the effects of different parameters of low-temperature extrusion on the physiochemical properties, textures, and palatability of food may provide important reference values for the development of low-GI food by low-temperature extrusion.
The aim of this study was to explore the feasibility of multi-grain recombination and low-temperature extrusion in the processing of diabetically suitable food. The effects of low-temperature extrusion on the physicochemical, functional, and sensory properties of extruded products dominated by naked barley and combined with a variety of grains were investigated.
Materials and methods
Materials and chemicals
Naked barley, oats, black rice, black sesame, kidney beans, and quinoa purchased from the local market (Wuxi, China) were ground by a laboratory mill (TAISITE, FW 100, China) through a 250 μm-sieve and stored at room temperature until analysis. Taka-amylase was purchased from URchem (China) and stored at 4°C. All other reagents and chemicals were of analytical grade.
Preparation of formula flours
Naked barley, black rice, quinoa, oats, colored kidney beans, and black sesame, which have healthful and beneficial values, were used in this study to provide hyperglycemic people with a safe substitute for staple foods. After a series of single-factor experiments, the content range of naked barley flour was 40–50%, of black rice flour was 15–25%, of oat flour was 5–15%, of kidney bean flour was 5–10%, of black sesame flour was 5–10%, and of gum Arabic was 5–10%. Products with good effects can be obtained within the ranges of content above. The formula selected for this study was 45% naked barley flour, 20% black rice flour, 10% oat flour, 5% colored kidney bean flour, 5% black sesame flour, and 5% gum Arabic. The contents of the components determined from all experimental grains are shown in .
Table 1. The formula of recombinant rice and compositions of all flours
Extrusion
A twin-screw extruder (Fumach, FMHE36-24, China) with a diameter (D) of 36 mm, a total configured screw length (L) of 24 D, and an extrusion die head containing three cylindrical holes (with the shape of each hole designed as a rice granule with a length of 15 mm and width of 2 mm) was used. The extruder barrel included five temperature-heating sections (Z1, Z2, Z3, Z4, Z5). To obtain extrudates with better shapes, a special temperature zone setting mode was used in this study: Z3 was set as the highest temperature zone; the temperatures of Z2 and Z4 were 10°C lower than that of Z3; the temperatures of Z1 and Z5 were 15°C lower than that of Z3. The extrusion temperature (Et), representing the temperature of Z3, ranged from 65°C to 85°C. The range of feed moisture (Fm) content was 25% to 35%, and the screw speed (Ss) was 70 to 80 rpm. The formula flour and the control sample (naked barley) were extruded to examine the optimal conditions of the extrusion process parameters. Some portions of the extrudates were stored directly at 4°C until further analysis. Others were dried at 40°C for 36 h in the laboratory oven (Boxin, DHG-9123A, China) and then milled to flour by a grinder (TAISITE, FW 100, China) through a 250 μm sieve. Ground extruded samples were also kept at 4°C until further analysis. The sketch view of low temperature extrusion treatment of naked barley-based formula flours is shown in .
Figure 1. Response surface plots of Degree of gelatinization (DG, %), DPPH radical scavenging activity (DPPH, %), Glycemic index (GI, %), Hardness (N) and Sensory Evaluation (SE) as affected by Extrusion temperature (Et), Feed moisture (Fm) and Screw speed (Ss) at corresponding 0 coded level of other two variables
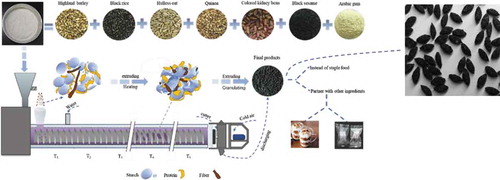
Chemical composition
Moisture, crude protein, crude fat, fiber, and ash content were analyzed according to the standard methods described by AACC[Citation9]: 44–01.01, 46–13.01, 30–20.01, 32–07.01, and 08–01.01, respectively. Total starch content was determined according to the total starch assay kit K-TSTA (Megazyme, Co., Ireland). All measurements were conducted in triplicate. The reported values are averages with relative standard deviations lower than 5%.
Water absorption index and water solubility index
The water absorption index (WAI) and water solubility index (WSI) were determined following the methods described by Anderson, Conway, Pfeifer, and Griffin.[Citation10] All measurements were conducted in triplicate. The reported values are averages with relative standard deviations lower than 5%.
Color measurement
The extrudates were measured directly for color within 1 h after extrusion. A portable chromatic meter (Konica Minolta, CR-400, Japan) was used for the determination of lightness (L), redness (a), and yellowness (b). Each sample was tested six times.
Degree of gelatinization
The gelatinization degree (DG) was determined by an enzymatic method. An accurately weighed 1 g sample was suspended in 50 mL deionized water in a flask (250 mL). The suspension was gelatinized in a boiling water bath for 20 min (shaking the flask every 10 minutes), and then added into 5 mL of 3% taka-amylase and reacted in a thermostatic gas bath (100 rpm) at 37°C for 2 h. After that, 2 mL HCl (1 M) was added to extinguish the enzyme, followed by addition of 2 mL of NaOH (1 M) and dilution with water to 250 mL volume in a volumetric flask. Subsequently, the reducing sugar content was analyzed by the method of Miller[Citation11] and represented the amount of reducing sugar in the completely gelatinized sample. While the determination of the reducing sugar contained in the extruded sample itself is not necessary to gelatinize for 20 min, the other steps are consistent with the method described above. The DG was expressed as the percentage of the reducing sugar of the sample itself relative to the reducing sugar of the absolutely gelatinized sample. All determinations were made in triplicate.
DPPH radical scavenging activity
DPPH radical scavenging activity (DPPH) of the samples was determined by the method of Brand-Williams, Cuvelier, and Berset[Citation12] with some modifications. 8.0 mg of a 0.04% DPPH-ethanol solution (1,1-diphenyl-2-picrylhydrazyl) was precisely weighed into a 200 mL brown volumetric flask, and ethanol was added to the constant volume. The DPPH radical scavenging activity of the milled extrudates and the control samples, including raw formula flour and formula flour boiled completely for 10 min, were analyzed to illustrate the difference in scavenging activity obtained by traditional thermal treatment compared to that obtained by extrusion. Each sample (1 g) was extracted with 10 mL of water and centrifuged at 4500 rpm for 20 min. The upper layer (1 mL) was reacted with 3.0 mL of DPPH-ethanol solution at room temperature. Absorbance (A) of the reaction solution was read at 517 nm at 0 min (Am) and 30 min (An) using an ethanol blank (Ab). All determinations were made in triplicate. Antioxidant activity was calculated by the equation below:
In vitro starch digestibility
Analysis of the GI of samples was based on the methods described by Connolly, Lovegrove, and Tuohy[Citation13] and Frei[Citation14] with some modifications. 100 mg of milled samples were suspended in 2 mL of distilled water in a centrifuge tube (50 mL) and then gelatinized in a boiling water bath for 10 min. After that, 13 mL of acetic acid sodium acetate buffer (pH 5.2, 0.2 mol/L) were added, 0.2 mL of mixed enzyme (290 U/mL α-amylase and 15 U/mL glucoamylase) were added, and the mixture was reacted in an enzyme reactor (MIULAB, MTH-100, China) at 37°C, 300 rpm for 10 min. 0.1 mL of the upper layer was added to 2 mL of ethanol. At 0 min, 30 min, 60 min, 90 min, 120 min, and 180 min, the glucose concentration was measured using the glucose oxidase-peroxidase kit (ref. 510-A, Sigma) and read at 510 nm. The rate of starch digestion was expressed as a percentage of the total starch hydrolyzed. Each cultivar and treatment were analyzed in triplicate. A non-linear model established by Goñi, Garcia-Alonso, and Saura-Calixto[Citation15] was applied to describe the kinetics of starch hydrolysis. The first-order equation has the form
where C is expressed as the percentage of starch hydrolyzed at time t, C∞ corresponds to the percentage of starch hydrolyzed at 180 min, k is the kinetic constant, and t is time (min). The area under the hydrolysis curve (AUC) was calculated using the equation
where C∞ corresponds to the equilibrium percentage of starch hydrolyzed after 180 min, tf is the final time (180 min), t0 is the initial time (0 min), and k is the kinetic constant. The calculated hydrolysis index (HI) was obtained by dividing the AUC of the sample by the AUC obtained for white bread (WB). The expected GI was calculated using the equation described by Ferrer-Mairal, Penalva-Lapuente, Iglesia, Urtasun, De Miguel-Etayo, Remon, et al.[Citation16]:
Instrumental texture analysis
The TA-XT Plus texture analyzer (SMS, TA. XTPLUS, England) was used for the Hardness analysis of extruded products within 6 h after extrusion. The instrument probe P/36 R was used for the test, and the program parameters of TPA test were set as follows: pre-measurement speed 1.00 mm/s; test speed 2.00 mm/s; posttest speed 1.00 mm/s; trigger force value 5.0 g; compression degree (Strain) 50.0%. Two compression intervals of 5.00 s and 6 randomly selected granules were tested in every experiment. Six replicates were made for each sample.
Sensory evaluation
Sensory evaluation (SE) of the target products kept at 4°C was performed within 6 hours after extrusion according to the method described by Jie et al.[Citation17] with some modifications. SE was done to analyze acceptability, including appearance, color, flavor, and palatability. The full grade of SE was 100, with 25% for appearance, 25% for color, 25% for flavor, and 25% for palatability. The total time of the exam was no more than 100 min. The extrudates were evaluated by a total of 20 semi-trained panelists from the Food College, Jiang University, China. The panelists were requested to gargle before and after the SE of each sample. The final scores were expressed as the sum of the scores of each attribute.
Experimental design and statistical analysis
For extrusion experiments, the Box-Behnken design was used to show the effects of interactions of Fm (g/100 g), Et (°C), and Ss (rpm) on the extrudates.[Citation18] This gave a total of 17 experimental runs, as described in . The effects of extrusion parameters on DG, GI, SE, hardness, WAI, WSI, and DPPH radical scavenging activity of extruded products were analyzed by response surface methodology. The experimental data were analyzed by analysis of variance (ANOVA), and the goodness of fit of the models and the significance of the regression coefficients are presented in . Regression models representing all the dependent variables as a function of Fm (X1), Et (X2), and Ss (X3) for the extrusion process were developed using the regression coefficients given in .
Table 2. Box-Behnken experimental design for extrusion process
Table 3. ANOVA and statistical parameters describing the effect of the independent variables on antioxidant, textural, and functional properties of extruded products
Data were statistically analyzed using the Design Expert 10 statistical software package (Stat-Ease Inc., USA). Origin version 8.5 were used to performed statistical analyzes (Origin labs, Northampton, MA). All experiments and procedures were performed in triplicate unless otherwise specified.
Results and discussion
Model fitting
shows that the mathematical models for DG, GI, SE, hardness, and DPPH radical scavenging activity of extrudates were well fitted with high coefficients of determination (R2 > 0.75) and insignificant (p > .05) p-values for lack of fit. The coefficients of Eq. (1) () were obtained by fitting the response data in Design Expert by employing a stepwise backward regression procedure to find the best-fit model.
Interpretation of respzonse surface curves and models
Independent and dependent variables were correlated on the basis of the model equations mentioned above, and response surface plots were obtained to present the effects of two independent variables on the response factor, keeping others at level-coded zero.
Effects of extrusion parameters on physicochemical properties of extrudates
shows that Et and Fm significantly (p < .05) affected the DG linearly. In addition, the quadratic term of Et also influenced the DG of extrudates significantly (p < .05). However, the DG of extrudates was not significantly (p < .05) affected by Ss within its experimental range. Because no significant (p > .05) change was observed in the WAI and WSI within the experimental range of Et, Fm, and Ss, the results of response surface analysis for WAI and WSI were not shown. shows that the WAI and WSI of extrudates had few changes within the experimental range. Overall, the differences in WAI and WSI in relation to Et are more obvious, possibly because the effect of Et within a short range is more significant on WAI and WSI than are Fm and Ss within the experimental ranges. This result was in accordance with the results of Lohani et al.[Citation18] who reported that the change of WAI could reflect the gelatinization of starch, and WAI was more sensitive to temperature. Previously, it was observed that the WAI and WSI were significantly increased with the Ss ranging from 160 rpm to 450 rpm in the extrusion of whole grain oats.[Citation19] Wani and Kumar[Citation20] reported that the WAI of fenugreek oat-pea extruded products were affected significantly by Fm and Et.
Table 4. Regression coefficients describing the relationship between dependent and independent variables for extrudates
Table 5. L, a, b, WAI, WSI of the formula extrudates, and L, a, b of raw barley and the raw formula flour
shows the response surface plots of the effects of Fm, Et, and Ss on DG of the extrudates. With simultaneous variation of Fm and Et, the DG of extrudates significantly (p < .05) increased by 26.3% with an increase in temperature from 65°C to 85°C regardless of the Fm level. Starch gelatinization is generally observed at 60–65°C. With increasing temperature, the starch gradually expands and ruptures, and at 95°C for 30 min, the starch can be completely gelatinized.[Citation21] Compared with traditional hydrothermal treatment, under the extrusion cooking conditions, amylopectin is more easily decomposed and absorbs more water, which makes it easier to be gelatinized.[Citation22] The DG significantly (p < .05) decreased by 8.7% with an increase in Fm from 25% to 35% at a low temperature (65°C), while a decrease of 7.1% was recorded for the DG with an increase in Fm at a higher temperature (85°C). With regard to the combined effect of Et and Ss on DG (), an increase in Et from 65°C to 85°C caused a significant (p < .05) increase of 30.3% in DG at slow Ss (60 rpm), but the DG increased by 27.6% when Et increased within the experimental levels at faster Ss (80 rpm). On the other hand, no significant (p > .05) change was observed in the DG when the Ss increased from 60 rpm to 80 rpm with a constant Et. Similarly, no significant (p < .05) change of the DG affected by both Fm and Ss was observed within the experimental ranges. However, another study reported that moderate Ss (300 rpm) caused a higher content of slowly-digestible starch with an accompanying decrease in rapidly-digestible starch within the experimental range, while low Fm (15%) resulted in the highest resistant starch.[Citation19]
Effect of extrusion parameters on functional properties of the extrudates
Phenolic compounds are well recognized as antioxidants that can prevent diseases such as atherosclerosis, diabetes, and various types of cancer.[Citation23] The majority of these compounds are very sensitive to processing conditions, such as temperature. For instance, greater than 50% of antioxidant activities are lost during thermal and other processing.[Citation24] The antioxidant activities of the extrudates and the control samples (raw formula flour and formula flour boiled completely for 10 min) were compared. The DPPH radical scavenging activity were in the range of 47.83–54.66%, while the values for the control samples were 65.89% and 29.43%, respectively. It can be concluded that the low-temperature extrusion technique (within the experimental range) can better retain the antioxidant activity of the products compared with traditional thermal treatment. This is likely because thermal treatment usually causes the loss of phenolics, while the mechanical effect of extrusion is helpful for releasing bound phenolics from the food matrix.[Citation25] Significant (p < .05) effects of the linear terms Fm, Et, and Ss on antioxidant properties within the experimental ranges were explicated by ANOVA (). shows that the DPPH radical scavenging rate of the extrudates significantly (p < .05) decreased by 6.83% with an increase in temperature from 65°C to 85°C regardless of the Fm level. This result was in accordance with that of Wang and Ryu,[Citation26] who reported that the DPPH radical scavenging activity of samples decreased after extrusion treatment compared with that of raw materials and decreased with increasing Et. The DPPH radical scavenging rate of the extrudates significantly (p < .05) increased by 0.83% with an increase in the Fm from 25% to 35% at a low Et, while decreased 4.01% with an increase in the Fm at a high Et. The combined effect of Ss and Et on the DPPH radical scavenging rate of extrudates is shown in . Previously, many researchers have reported and explained this trend of the milder effect of extrusion on the material with the presence of a greater amount of Fm causing greater retention of the DPPH radical scavenging rate.[Citation27] The DPPH radical scavenging rate of the extrudates significantly (p < .05) decreased by 1.29% with an increase in Ss in the range of 60–80 rpm at low Et (65°C) but increased by 3.62% with an increase in Ss at high Et (85°C). The combined effect of Ss and Fm on the DPPH radical scavenging rate of the extrudates was not significant within the experimental ranges. However, it has been reported that the total antioxidant capacity of samples decreased with an increase in Ss and a decrease in Fm within the Ss range of 220–340 rpm and the Fm range of 11.0–15.0%.[Citation28]
GI is defined as the incremental blood glucose area following the digestion of food, expressed as the percentage of the corresponding area following a carbohydrate-equivalent load of a reference product.[Citation29] The GI was originally developed to help improve blood sugar control in diabetes. GI is a relative ranking of carbohydrate in foods according to how they affect blood glucose levels. Carbohydrates with lower GI values are digested, absorbed, and metabolized more slowly and cause a lower and slower rise in blood glucose. Recent studies have shown that the GI can guide people with diabetes to more effectively arrange their diets and reach the goal of improved health.[Citation1] In vitro starch digestibility was used to evaluate the digestive characteristics of foods. The combined effects of extrusion and Fm on the GI of extrudates are presented in . The GI significantly (p < .05) increased by 6.18 with an increase in Et from 65°C to 85°C. The GI of the extrudates increased significantly (p < .05) by 0.53 with an increase in Fm in the range of 25–35% at a low temperature (65°C), while it increased 2.16 with a similar increase in Fm at a higher temperature (85°C).
Effect of extrusion parameters on the sensory properties of extrudates
Hardness is one of the most important sensory properties of foods to evaluate. At present, the texture of most snacks made by extrusion is crispy, loose, and porous. The purpose of this study was to explore a novel extruded food with a chewy and moderately hard texture. The influence of extrusion parameters on the hardness of the target products was researched in this study. The trend observed for hardness upon simultaneous variation of Et and Fm is shown in . The hardness of the extruded products increased significantly (p < .05) by 0.35 × 103 N when the Et was elevated from 65°C to 85°C at low Fm (25%). However, at high Fm (35%), when Et increased within the experimental range, hardness decreased by 0.48 × 103 N.
Color is another key indicator of the market acceptance of products, which has an important impact on the intention of customers to decide whether to purchase a product. The hunter color values L, a, and b of the extrudates, raw barley grain, and raw formula flour are shown in . The L of raw barley grain was 92.05 and decreased after the addition of other corns. This indicates that the formula flours were slightly darker compared to the raw barley. The L and b values of the products were significantly reduced after extrusion, while the a values were slightly increased. Damodaran[Citation30] correlated decreasing L with the Maillard reaction between carbonyl compounds (reduced sugars) and amino compounds (amino acids and proteins) by heat treatment. However, the a values of the extrudates were higher than those of the formula flour. Similar results were obtained by Kristiawan et al.[Citation31] in the extrusion of pea flour. It can be concluded that some components of the blends changed during the extrusion process. Kristiawan et al.[Citation31] also found that L and a were correlated with protein aggregations by covalent bonds in the samples.
Sensory evaluation plays an important role in food industries by indicating the acceptability of products. Thus, the appearance, color, flavor, and palatability were used as indicators of sensory evaluation in this study to assess the popularity of the extrudates. shows that, at low Fm (25%), the sensory evaluation increased significantly (p < .05) with increased Et until the Et reached 75°C, but it decreased above this temperature. This trend can be explained by the surface of the extruded products becoming rougher and darker with the increase of Et at low Fm (25%), resulting in poor palatability and visual effect. Moisture loss of the extrudates was aggravated by the increasing Et. Similarly, at high Fm (35%), starch generally gelatinized with the increase of Et, and the texture of the extrudates became so soft that palatability became worse. No significant change was found in the sensory evaluation when the Ss changed from 60 rpm to 80 rpm at a low (65°C) or high (85°C) Et. Obviously, the sensory evaluation was significantly affected by the Et and Fm. There was a phenomenon that the flavor of the extrudates was enriched with an increase of Et. It can be concluded that, with the increase of the Et, more Maillard reaction products with a certain flavor were formed, and more flavorful substances in the grains separated out with the intensified Maillard reaction. Flavor is the most important parameter that maximizes food quality and global competitiveness, and the Maillard reaction is factor for the formation of food flavor during heat treatment.[Citation32]
Optimization and validation of the extrusion process
Optimal conditions of the extrusion process parameters were obtained by maximizing the sensory evaluation, DPPH radical scavenging rate, and DG and minimizing the GI. The optimal parameters required to obtain the best products were Et = 74.24°C, Fm = 35%, and Ss = 80 rpm. The predicted and actual experimental values of the formula flour and naked barley obtained at estimated optimum conditions are shown in . The extrudates at optimum extrusion conditions including barley only are referred to as control extrudates. The DG of extrudates was 19.79% lower than that of barley extrudates. This may be due to the gum Arabic and starch competing for water adsorption, which increases the gelatinization temperature of starch. Complexes formed by the combination of lipids (with the fat content in the formula powder being higher than that of naked barley) with amylose are not easily destroyed by heating, which may contribute to the lower DG. The GI of the formula extrudates was 8.48 lower than that of the barley extrudates. This may be due to the addition of more dietary fiber, which form complexes with protein and starch at lower temperatures, making the starch less susceptible to enzymatic hydrolysis.[Citation33] The DPPH radical scavenging rate of the extrudates was 12.97% higher than that of the barley extrudates, which may be attributed to the high content of phenolic compounds in the other flours included in the formula flours. The sensory evaluation of the formula extrudates was higher than that of the barley extrudates, which indicates that multi-grain blending is helpful in improving the sensory quality and acceptability of the extrudates.
Table 6. The predicted and actual experimental values of the formula flour and naked barley obtained at estimated optimum conditions for extrusion process
Conclusion
Naked barley is a naturally low-GI grain, which may help delay or prevent hyperglycemia. However, due to the rough taste of barley, the market acceptance rate for foods made from this grain is low. Similarly, there are many other grains in nature that have protective effects against hyperglycemia. The purpose of this study was to explore the possibility of low-GI food processing by combining low-temperature extrusion and a multi-grain blend. Products prepared by low-temperature extrusion of formula flours showed a higher DPPH radical scavenging rate, lower GI, and higher sensory properties compared with those of naked barley extrudates. Temperature played a prevalent role in influencing the physicochemical properties by increasing the DG and GI of the extrudates. In comparison, a low Et provided better retention of polyphenols and some beneficial ingredients in the grains. Compared with the traditional cooking process, the extrusion process resulted in a higher retention of the DPPH radical scavenging rate. The sensory properties of the extrudates were significantly affected by the Et, Fm, and Ss. Through the analysis of the sensory evaluation results, it was determined that people prefer products with high gelatinization and a certain degree of hardness. Thus, the multi-grain compounding and extrusion technology described here can be used as an efficient way to produce products with high nutritional values, slow digestion characteristics, and high sensory properties suitable for people with hyperglycemia.
Acknowledgments
This study was supported by the National “Thirteenth Five-Year” plan for Science & Technology Support of China (No. 2016 YFD0400304), Jiangsu Agricultural Science and Technology Innovation Fund (CX (17) 2022), the Science & Technology Pillar Program of Jiangsu Province (BE2018304) and Jiangsu Key Laboratory of Advanced Food Manufacturing Equipment & Technology (FM-201904). No conflicts of interest are declared for any of the authors.
Additional information
Funding
References
- Brand-Miller, J. C.;. Postprandial Glycemia, Glycemic Index, and the Prevention of Type 2 Diabetes. Am. J. Clin. Nutr. 2004, 80(2), 243–244. DOI: 10.1051/rnd:2004054.
- Wirkijowska, A.; Rzedzicki, Z.; Kasprzak, M.; Błaszczak, W. Distribution of (1-3)(1-4)-β-d-glucans in Kernels of Selected Cultivars of Naked and Hulled Barley. J. Cereal Sci. 2012, 56(2), 496–503. DOI: 10.1016/j.jcs.2012.05.002.
- Škrbić, B.; Milovac, S.; Dodig, D.; Filipčev, B. Effects of Hull-less Barley Flour and Flakes on Bread Nutritional Composition and Sensory Properties. Food Chem. 2009, 115(3), 982–988. DOI: 10.1016/j.foodchem.2009.01.028.
- Arribas, C.; Cabellos, B.; Sanchez, C.; Cuadrado, C.; Guillamon, E.; Pedrosa, M. M. The Impact of Extrusion on the Nutritional Composition, Dietary Fiber and in Vitro Digestibility of Gluten-free Snacks Based on Rice, Pea and Carob Flour Blends. Food Funct. 2017, 8(10), 3654–3663. DOI: 10.1039/c7fo00910k.
- Lagassé, S.; Hatcher, D.; Dexter, J.; Rossnagel, B.; Izydorczyk, M. Quality Characteristics of Fresh and Dried White Salted Noodles Enriched with Flour from Hull-less Barley Genotypes of Diverse Amylose Content. Cereal Chem. 2006, 83(2), 202–210. DOI: 10.1094/CC-83-0202.
- Guo, Y.; Liu, W.; Wu, B.; Wu, P.; Duan, Y.; Yang, Q.; Ma, H. Modification of Garlic Skin Dietary Fiber with Twin-screw Extrusion Process and in Vivo Evaluation of Pb Binding. Food Chem. 2018, 268, 550–557. DOI: 10.1016/j.foodchem.2018.06.047.
- Masatcioglu Tugrul, M.; Yalcin, E.; Kim, M.; Ryu, G.-H.; Celik, S.; Köksel, H. Physical and Chemical Properties of Tomato, Green Tea, and Ginseng-supplemented Corn Extrudates Produced by Conventional Extrusion and CO2 Injection Process. Eur. Food Res. Technol. 2013, 237(5), 801–809. DOI: 10.1007/s00217-013-2053-3.
- Butterworth, P. J.; Warren, F. J.; Ellis, P. R. Human α-amylase and Starch Digestion: An Interesting Marriage. Starch Starke. 2011, 63(7), 395–405. DOI:10.1002/star.201000150.
- AACC international. Approved Methods of American Association of Cereal Chemists, 11th ed.; American Association of Cereal Chemists: St. Paul, MN, 2010.
- Anderson, R. A.; Conway, H. F.; Pfiefer, V. F.; Griffin, E. L., Jr. Roll and Extrusion-cooking of Grain Sorghum Grits. Cereal Sci. Today. 1969, 14, 11. DOI: 10.1111/j.1365-2184.1969.tb00244.x.
- Miller, G. L.;. Use of Dinitrosalicylic Acid Reagent for Determination of Reducing Sugar. Anal. Biochem. 1959, 31(3), 426–428. DOI: 10.1021/ac60147a030.
- Brand-Williams, W.; Cuvelier, M. E.; Berset, C. Use of a Free Radical Method to Evaluate Antioxidant Activity. LWT Food Sci. Technol. 1995, 28(1), 25–30. DOI: 10.1016/S0023-6438(95)80008-5.
- Connolly, M. L.; Lovegrove, J. A.; Tuohy, K. M. In Vitro Fermentation Characteristics of Whole Grain Wheat Flakes and the Effect of Toasting on Prebiotic Potential. J. Med. Food. 2012, 15(1), 33–43. DOI: 10.1089/jmf.2011.0006.
- Frei, M.; Siddhuraju, P.; Becker, K. Studies on the in Vitro Starch Digestibility and the Glycemic Index of Six Different Indigenous Rice Cultivars from the Philippines. Food Chem. 2003, 83(3), 395–402. DOI: 10.1016/S0308-8146(03)00101-8.
- Goñi, I.; Garcia-Alonso, A.; Saura-Calixto, F. A Starch Hydrolysis Procedure to Estimate Glycemic Index. Nutr. Res. 1997, 17(3), 427–437. DOI: 10.1016/S0271-5317(97)00010-9.
- Ferrer-Mairal, A.; Penalva-Lapuente, C.; Iglesia, I.; Urtasun, L.; De Miguel-Etayo, P.; Remon, S.; Cortes, E.; Moreno, L. A. In Vitro and in Vivo Assessment of the Glycemic Index of Bakery Products: Influence of the Reformulation of Ingredients. Eur. J. Nutr. 2012, 51(8), 947–954. DOI: 10.1007/s00394-011-0272-6.
- Jie, H. U.; De-Yuan, L. I.; Qiao, Y.; Huo, Y. Q.; Quartermaster, D. O. J. F. R., & Development. Studies on the Optimization of Extrusion Restructuring Rice Material Ratio by Formula Uniform Design Method. Food Res. Dev. 2016,37(10), 78-81. DOI: 10.3969/j.issn.1005-6521.2016.10.020
- Lohani, U. C.; Muthukumarappan, K. Process Optimization for Antioxidant Enriched Sorghum Flour and Apple Pomace Based Extrudates Using Liquid CO2 Assisted Extrusion. LWT. 2017, 86, 544–554. DOI: 10.1016/j.lwt.2017.08.034.
- Brahma, S.; Weier, S. A.; Rose, D. J. Effects of Selected Extrusion Parameters on Physicochemical Properties and Invitro Starch Digestibility and β-glucan Extractability of Whole Grain Oats. J. Cereal Sci. 2016, 70, 85–90. DOI: 10.1016/j.jcs.2016.05.001.
- Wani, S. A.; Kumar, P. Development and Parameter Optimization of Health Promising Extrudate Based on Fenugreek Oat and Pea. Food Biosci. 2016, 14, 34–40. DOI: 10.1016/j.fbio.2016.02.002.
- Deshpande, S. S.; Sathe, S. K.; Rangnekar, P. D.; Salunkhe, D. K. Functional Properties of Modified Black Gram (Phaseolus Mungo L.) Starch. J. Food Sci. 2010, 47(5), 1528–1602. DOI: 10.1111/j.1365-2621.1982.tb04975.x.
- Whalen, P. J.; Bason, M. L.; Booth, R. L.; Walker, C. E.; Williams, P. J. Measurement of Extrusion Effects by Viscosity Profile Using the Rapid Visco Analyser. Cereal Foods World. 1997, 42(6), 469–475. DOI: 10.1016/S0168-1605(97)00053-6.
- Meera, K.; Smita, M.; Haripriya, S.; Sen, S. Varietal Influence on Antioxidant Properties and Glycemic Index of Pigmented and Non-pigmented Rice. J. Cereal Sci. 2019, 87, 202–208. DOI: 10.1016/j.jcs.2019.03.005.
- Brennan, C.; Brennan, M.; Derbyshire, E.; Tiwari, B. K. Effects of Extrusion on the Polyphenols, Vitamins and Antioxidant Activity of Foods. Trends Food Sci. Technol. 2011, 22(10), 570–575. DOI: 10.1016/j.tifs.2011.05.007.
- Zhang, R.; Khan, S. A.; Chi, J.; Wei, Z.; Zhang, Y.; Deng, Y.; Liu, L.; Zhang, M. Different Effects of Extrusion on the Phenolic Profiles and Antioxidant Activity in Milled Fractions of Brown Rice. LWT. 2018, 88, 64–70. DOI: 10.1016/j.lwt.2017.09.042.
- Wang, Y.; Ryu, G. Physicochemical and Antioxidant Properties of Extruded Corn Grits with Corn Fiber by CO2 Injection Extrusion Process. J. Cereal Sci. 2013, 58(1), 110–116. DOI: 10.1016/j.jcs.2013.03.013.
- Bisharat, G. I.; Lazou, A. E.; Panagiotou, N. M.; Krokida, M. K.; Maroulis, Z. B. Antioxidant Potential and Quality Characteristics of Vegetable-enriched Corn-based Extruded Snacks. J. Food Sci. Technol. 2015, 52(7), 3986. DOI: 10.1007/s13197-014-1519-z.
- Özer, E. A.; Herken, E. N.; Güzel, S.; Ainsworth, P.; İbanoğlu, Ş. The Effect of Extrusion Process on the Antioxidant Activity and Phenolics in a Nutritious Snack Food. Int. J. Food Sci. Technol. 2010, 41(3), 289–293. DOI: 10.1111/j.1365-2621.2005.01062.x.
- Livesey, G. Glycaemic Responses and Toleration. Sweeteners and Sugar Alternatives in Food Technology, 2nd ed.; Wiley-Blackwell, 2006. Doi: 10.1002/9781118373941.ch1
- Damodaran, S.;. Amino Acids, Peptides, and Proteins. In Food Chemistry, 3rd ed.; Fennema, O. R., Ed.; Springer: New York, 1996; pp 321–429. DOI: 10.1039/9781847557438.
- Kristiawan, M.; Micard, V.; Maladira, P.; Alchamieh, C.; Maigret, J. E.; Reguerre, A. L.; Emin, M. A.; Della Valle, G. Multi-scale Structural Changes of Starch and Proteins during Pea Flour Extrusion. Food Res. Int. 2018, 108, 203–215. DOI: 10.1016/j.foodres.2018.03.027.
- Cui, H.; Yu, J.; Xia, S.; Duhoranimana, E.; Huang, Q.; Zhang, X. Improved Controlled Flavor Formation during Heat-treatment with a Stable Maillard Reaction Intermediate Derived from Xylose-phenylalanine. Food Chem. 2019, 271, 47–53. DOI: 10.1016/j.foodchem.2018.07.161.
- Tacer-Caba, Z.; Nilufer-Erdil, D.; Boyacioglu, M. H.; Ng, P. K. W. Evaluating the Effects of Amylose and Concord Grape Extract Powder Substitution on Physicochemical Properties of Wheat Flour Extrudates Produced at Different Temperatures. Food Chem. 2014, 157(11), 476–484. DOI: 10.1016/j.foodchem.2014.02.064.