ABSTRACT
Prunus padus L. is a wild growing plant in various parts of the world with low soil requirements. This work evaluated the content of selected phytochemicals, antioxidant potential and determined the antimicrobial effect of individual anatomical parts of P. padus (i.e. leaves, bark, and fruits). It was shown that the examined anatomical parts of bird cherry (Prunus padus L.) contained polyphenols and low-molecular organic acids. The highest content of phenolic acids was found in P. padus leaves (1063 ± 26 mg/100 g dm). The analysis regarding flavonols confirmed that the highest content was found in the bark (1530 ± 61 mg/100 g dm). The content of low molecular weight organic acids, whose highest value was found in the fruits (1658 ± 27 mg/100 g dm) was also assessed. The highest antioxidant activity with DPPH radicals was confirmed for the bark (8.9 ± 0.03 mg TE/g dw). The FRAP test also showed the highest activity for the bark extract (380 ± 18 µM FeSO4/g dw). It was shown that signals located in the region of negative potentials in SWV (square wave voltammetry) indicated a high reducing ability of compounds present in P. padus, which means that they can be a very efficient protective barrier against oxidizing agents (i.e. the greatest intensity was for the leaves extract). The highest activity against Gram-negative bacteria was recorded in bird cherry leaves and the lowest in the bark. It showed that the leaves, fruits, and bird cherry bark can be a good source of antioxidant compounds and an attractive raw material with antimicrobial properties that can be widely applied, which is confirmed by the obtained results.
Introduction
Antioxidants are substances that prevent the oxidation of other compounds or neutralize free radicals that contain an unpaired electron. Free radical causes oxidative stress responsible for damage to cellular macromolecules, including lipids, proteins, and DNA in organisms.[Citation1] Therefore, it is important to provide products in the diet that contain antioxidants that reduce the effects of oxidative stress.
Bird cherry is a popular plant belonging to the genus Prunus from the family Rosaceae. It is characterized by edible, dark fruits with a bitter aftertaste that is used as an ingredient in tinctures and syrups.[Citation2] Bird cherry bark, obtained from young twigs, together with leaf buds is also used as an herbal raw material. It was used in folk medicine as an astringent, diuretic, and anti-rheumatic agent. Black cherry juices and tinctures are known for their beneficial health properties. The fruit of the bird cherry is also used to color wines, most often apple ones. Heavily toasted fruit can also become a coffee substitute. In Siberia, dried fruit is made into flour, added to confectionery, cakes, dumplings, and even bread. In Sweden or Kalmykia (a region in Russia), black cherry fruits are eaten boiled in milk. You can also get a bitter oil similar to almond oil from the seeds. It can be used as an ingredient in meat spices. However, information on the application of bird cherry is limited.[Citation2]
Leaves, fruits, and bark of bird cherry (P. padus) contain phenolic compounds such as phenolic acids, e.g. gallic acid, ferulic acid, p-coumaric acid, or chlorogenic acid, as well as flavonoids like quercetin, catechin, or rutin. We can also find organic acids such as citric acid, shikimic acid, malic acid, or quinic acid in its composition. Data on antioxidant properties of individual plant parts are available in the literature,[Citation3–6] but they are not complete.
There is little research available on bird cherry and the compounds contained in its individual parts. No information was found regarding direct determination of their effect on the reduction of guanine oxidative damage caused by the OH• radical using an electrochemical DNA biosensor. Electrochemical biosensors show a significant advantage over other methods enabling the determination of 8-oxoguanine level, because they allow direct testing of the DNA sample, without the need for its hydrolysis, which is necessary in other highly sensitive methods. Hydrolysis can cause additional genetic damage in the tested genetic material samples, including oxidative damage, which may be the cause of false-positive test results.[Citation7]
Available literature also shows antimicrobial and antioxidant activity of selected cultivars and anatomical parts of bird cherry. Therefore, the purpose of the work was to determine the possible beneficial properties of P. padus fruits, bark, or leaves. Water extracts of individual parts of bird cherry have been tested for antioxidant and antimicrobial activity as well as for the content of selected phytochemicals. The aim of the study was to assess the possible pro-health effects of bird cherry and its beneficial properties for health, and thus the possibility of its use in diet therapy and functional food.
Material and methods
Material and extract preparation
The research material consisted of leaves, bark, and fruits of P. padus that had been selected in previous studies as a raw material with a higher antioxidant potential[Citation6] of leaves comparing with P. serrotina.[Citation6] All anatomical parts of the P. padus were in the orchard farm in Ozierany Małe in Podlasie, Poland (53º 13ʹ 14.865” N 23º 51ʹ 9.327” E). The leaves, bark, and fruits were lyophilized (CHRIST 1‐4 LSC freeze dryer – Martin Christ Gefriertrocknungsanlagen GmbH, Osterode am Harz, Germany). Lyophilization conditions were as follows: the condensation temperature in the freeze dryer was at −28°C, the temperature on the freeze dryer shelf at – 20°C, and the product temperature at – 4°C. After that research material was grinding (Grindomix GM 200 Retsch, Haan, Germany) for 150 seconds at 1800 x g at 22°C.
The water extraction was performed using distilled water, at 85 °C temperature. The procedure was as follows: 50 g of examined material was mixed with 1000 mL of distilled water was mixed and extracted in a water bath for 15 minutes. The extracts were filtered and centrifuged (800 x g, 15 minutes). The fractions were decanted and filtered (Whatman 1:11 μm). The prepared extracts were stored in dark tubes until examination at 4 °C. The experiment samples were named as follows: extract from P. padus leaves as PPL, extract from P. padus bark as PPB and extract from P. padus fruits as PPF.
Characteristic of color of P. padus extract
Color of leaves, bark, and fruit extracts was measured. Color measurement was run in the L* a* b* CEN unit system using a CM-5 spectrometer (Konica Minolta, Japan) according to the methodology described by the device producer. As a source of light, D 65 was applied, and color temperature equaled 6504 K. The observation angle of standard colorimetric observer was 10°. Measurements for each sample were repeated fivefold. The instrument calibration was performed with use of black pattern.
Phenolic acids and flavonols
Phenolic compounds in samples were analyzed after alkaline and acidic hydrolysis. Samples were placed in sealed 17 ml culture test tubes, where first alkaline and then acid hydrolysis was run. In order to run alkaline hydrolysis, 1 ml distilled water and 4 ml 2 M aqueous sodium hydroxide was added to test tubes. Tightly sealed test tubes were heated in a water bath at 95°C for 30 min. After cooling (approx. 20 min), test tubes were neutralized with 2 ml 6 M aqueous hydrochloric acid solution (pH = 2). Next, samples were cooled in water with ice. Flavonoids were extracted from the inorganic phase using diethyl ether (2x2ml). Formed ether extracts were continuously transferred to 8 ml vials. Next, acid hydrolysis was run. For this purpose, the aqueous phase was supplemented with 3 ml 6 M aqueous hydrochloric acid solution. Tightly sealed test tubes were heated in a water bath at 95°C for 30 min. After being cooled in water with ice, the samples were extracted with diethyl ether (2 x 2 ml). Produced ether extracts were continuously transferred to 8 ml vials, after which they were evaporated to dryness in a stream of nitrogen. Prior to analysis, samples were dissolved in 1 ml methanol. Analysis was performed using an Acquity H class UPLC system equipped with a Waters Acquity PDA detector (Waters, USA). Chromatographic separation was performed on an Acquity UPLC® BEH C18 column (100 mm×2.1 mm, particle size 1.7 μm) (Waters, Ireland). Elution was carried out in a gradient using the following mobile phase composition: A: acetonitrile with 0,1% formic acid, B: 1% aqueous formic acid mixture (pH = 2). Concentrations of phenolic compounds were determined using an internal standard at wavelengths λ = 320 nm and 280 nm and finally given in mg/100 g dm of P. padus leaves, bark, and fruits. Compounds were identified based on the comparison of retention time of the analyzed peak with standard retention time and by adding a specific amount of the standard to the analyzed samples and repeating the analysis. Detection level was 1 μg/g.
Low molecular weight organic acids (LMWOAs)
The analysis of LMWOAs was performed using a Waters Acquity H-class UPLC system. Separation was achieved on an Acquity UPLC BEH C18 column (150 mm × 2.1 mm, 1.7 µm, Waters) thermostated at 35°C. The gradient elution was performed with water and acetonitrile (both containing 0.1% formic acid, pH = 2) at a flow rate of 0.4 mL/min. Detection was carried out in a Waters Photodiode Array Detector (Waters Corporation, Milford, MA, USA) at λ = 280 nm as the preferred wavelength for oxalic, maleic, citric, malic, quinic, and shikimic acids. Compounds were identified by comparing of retention time of the analyzed peaks with the retention time of standards or by adding a specific amount of the standard to the analyzed samples and a repeated analysis. The LMWOAs found were quantified by comparing of the area of their peaks recorded at 280 nm with calibration curves obtained from commercial standards of each compound obtained from Sigma (St. Louis, MO, USA). The results were expressed in milligrams per 100 g of dry weight of sample.
Electrochemical analysis of antioxidant activity
The content of redox compounds in bird cherry leaves, fruits, and bark extracts was determined using square wave voltammetry (SWV) and the procedure described previously Szczepaniak et al.[Citation8] Voltammetric measurements were performed using potentiostat PGSTAT12 with the GPES 4.9 control software (EcoChemie, The Netherlands). For the measurements was used a three-electrode measuring system consisting of a reference electrode Ag/AgCl (3 M KCl) (Mineral, Poland), platinum as an auxiliary electrode (Mineral, Poland) and carbon paste as a working electrode (CPE). The CPE was made by itself according to a described procedure Ligaj et al.[Citation9] Carbon paste was made by mixing graphite powder (Sigma) with mineral oil (Sigma) in the ratio of 70:30 (w/w). The surface of the carbon paste was renewed before use by removing its outer layer on a filter paper, application of fresh paste, and polishing it to a smooth finish on a frosted glass microscope slide. Before electrochemical measurement, the surface of CPE was conditioned in 0.05 M phosphate buffer mixed with 0.01 M KCl (pH 7.0) at a potential of +1.7 V for 60 s. After that the electrodes were immersed for 120 s in the solution containing extract dissolved in phosphate buffer in the ratio 1:1 (v/v), whether the SWV measurement in the range from −0.3 V to +1.4 V was made. Applied SWV parameters were as follows: step potential of 5 mV, frequency of 50 Hz, and amplitude of 40 mV. Three repetitions of SWV measurement for each extract were performed. SWV voltammograms were smoothed using Savitzky–Golay’s filter.[Citation10] From SWV voltammograms, the baselines determined with moving average procedure were subtracted and finally were determined the data including peak potential, peak height (current), peak area for each signal, and the total peak areas. On the basis of the our results for P. padus extracts[Citation8] were also determined an electrochemical index (EI) describing the electrochemical activity of tested extracts, expressed as the total area of all redox signals, in relation to 1 g dry matter of examined plant material. In the case of tasted samples, 1 ml of extracts was prepared from 0.063 g of weight, which after dilution in the buffer gave the final dry matter content of plant material in the tested sample of 0.03125 g.
Antioxidative potential analysis by spectrophotometric method
The total phenolic content (TPC) of the obtained extracts from individual anatomical parts of bird cherry was determined using the method described by Kulczyński et al. with minor modifications.[Citation11] The method consisted of: Aliquots of 100 µL diluted in 900 µL of 40% ethanol (Sigma-Aldrich, Germany) were mixed with 1 mL of Folin–Ciocalteu reagent (Sigma-Aldrich, Germany), followed by the addition of 1 mL of 35% sodium carbonate (POCH, Poland). Obtained samples were vortexed for 5 s and after incubation in darkness at room temperature for 90 min, the absorbance of the reaction mixture was measured at 765 nm against blank. The TPC content was then examined and expressed as mg of gallic acid (Sigma-Aldrich, Germany) equivalents (GAE) per 1 g (mg/1 g) of dry mass using the calibration curves of gallic acid.
The study on the scavenging power of DPPH-free radicals was based on the reduction of absorbance of a DPPH (2,2-diphenyl-1-picrylhydrazyl) solution at wavelength 517 nm in the presence of free radicals.[Citation12] Measurements were performed using SP-830 Plus apparatus (Metertech, Taiwan). The free radical scavenging strength and its percentage of DPPH radical scavenging were evaluated on the basis of the standard curve for y = 321.54x + 21.54 (R2 = 0.986) and presented as mg TE (Trolox Equivalent)/1 g dw of extract.
The ABTS cation radical scavenging activity was measured according to the TEAC (Trolox Equivalent Antioxidant Capacity) test according to the methodology described by Kobus-Cisowska et al.[Citation12] Spectrophotometric measurement of the ability to scavenge ABTS•+ formed from ABTS (2,20-azinobis-(3-ethylbenzothiazoline-6-sulfonic acid)) by oxidation with potassium persulfate was carried out at a wavelength of 414 nm using SP-830 Plus apparatus (Metertech, Taiwan). The percentage rate of ABTS•+ scavenging was calculated from the standard curve for y = 121.63x +26.33 (R2 = 0.96) and expressed as mg TE/g dw of extract.
In the aqueous extracts obtained from the bird cherry, the antioxidant properties were determined using a ferric reducing/antioxidant power assay (FRAP method) according to procedure described by O’Sullivan et al.[Citation13] FRAP reagent [2 mL; 0.01 mol TPTZ (2,4,6-tripyridyl-s-triazine) in 0.04 mol HCl, 0.02 mol FeCl3 .6H2O and 0.3 mol acetate buffer] was added to 1 µL of each sample diluted in 999 µL distilled H2O. A calibration curve was constructed using FeSO4 7H2O. Samples were incubated for 30 min and then the absorbance was measured at 593 nm (Metertech SP880, Taiwan). The obtained results were expressed as uM FeSO4/1 g dw of extract.
Antimicrobial activity testing using the well-diffusion method
In order to obtain a clear bacterial layer, the liquid Mueller-Hinton agar medium was inoculated with a 10% 24-h indicator culture with an optical density of 0.5 on McFarland scale and poured into Petri dishes. A well was drilled in the surface of the solid medium inoculated with indicator microorganisms, to which 50 µl of the extract was added. Plates were incubated under conditions suitable for a given group of microorganisms for 24–48 h. Then, the growth inhibition zone of indicator microorganisms was measured (clearing around the application site of the sample).
Indicator microorganisms like Gram-positive bacteria Enterococcus faecium (ATCC 27270), Enterococcus faecium (ATCC 27270), Staphylococcus aureus (ATCC 25923), Lactobacillus fermentum (ATCC 14932), Clostridium butyricum (ATCC 13076), Listeria monocytogenes (ATCC 19115) and Bacillus coagulans (GBI-30, 6086) and Gram-negative bacteria: Klebsiella pneumoniae (ATCC 31488), Salmonella enteritidis (ATCC 860), Pseudomonas aeruginosa (ATCC 27853), Acinetobacter baumannii (ATCC 19606) and as well as fungi of the species Candida utillis (ATCC 9950), Aspergillus sp. and Fusarium sp. were propagated in Muller-Hinton medium (Oxoid, UK) at 30°C (yeast) or 37°C (bacteria) for 24 h.
Statistical analysis
All assays were conducted in triplicates and results expressed as mean ± SD. Statistical analysis was performed using Microsoft Excel 2013 software (USA) and Statistica 13 software (StatSoft, Poland). One way ANOVA testing was used to analyze statistical differences. The p value lower than 0.05 was assumed as the level of significance. Additionally, principal component analysis was used (PCA). Electrochemical results were treated as an additional factor to the model based on standard analytical techniques. The p values for Levene’s test of independent variables were calculated.
Results
The obtained extracts were tested in terms of physicochemical properties and the results are presented in . The osmolality of the extracts that indicates the freezing point of the extract and its differences compared to the freezing of water, i.e. giving a measure of the osmotic pressure of the tested extract, showed that the highest values were obtained for bird cherry fruit extract (PPF) 0.21 ± 0.01 mOsm/kg H2O, and the lowest for bark extract (PPB) amounting to 0.14 ± 0.01 mOsm/kg H2O. The freezing point was the lowest for fruit extracts (PPF) and amounted to – 0.39b ± 0.01 °C. The color of the extracts was also assessed, which, as demonstrated, differed in terms of parameters tested. However, the color of the leaf (PPL) and bark extracts (PPB) was similar. Parameter L*, responsible for determining the brightness of the extract, was 26a ± 0.3 for leaves (PPL), 29b ± 1.7 for bark (PPB) and 28b ± 0.3 for fruits (PPF), respectively. Parameter a*, responsible for the color change in the range from green to red, was 11b ± 0.3 for bark (PPB) and it was the highest value. Parameter b*, responsible for color change in the range from blue to yellow, showed lower values for the leaf extract (PPL) 1.8a ± 0.1, while this parameter for bark and fruit extracts was similar and amounted to 3b ± 0.3 (PPB) and 3b ± 0.7 (PPF).
Table 1. Characteristics of the tested leaves, bark, and fruits of P. padus, given in CIE L*a*b* units and osmolality
The highest content of phenolic acids was found in P. padus leaves (PPL), which was 1063 ± 26, while the lowest content was found in the fruits (PPF) and amounted to 502.± 25 (). Dominant acids in all parts of the plant studied were as follows: ferulic acid, p-coumaric acid, gallic acid, and chlorogenic acid. In leaves (PPL), p-coumaric acid was the most abundant (175 ± 9), while syringic acid the least (0.2 ± 0.01). Ferulic acid was dominant in bark (PPB) of P. padus, and vanillic acid (353 ± 11) was the least abundant (1 ± 0.01). Fruits (PPF), on the other hand, had the highest amount of p-coumaric acid (130 ± 5). The analysis regarding flavonols confirmed that the highest content was found in the bark (PPB) of bird cherry (1530 ± 61), and the lowest was in the leaves (PPL) (252 ± 11). Of the flavonols tested, catechin was the dominant in all anatomical parts of the plant. The content of low molecular weight organic acids, whose highest value was found in the fruits (PPF) (1658 ± 27) was also assessed. In contrast, the leaves (PPL) had the highest quantity of shikimic acid (259 ± 10) and citric acid (107 ± 6), and the lowest amount of maleic acid (1 ± 0.01), which in turn was found in a statistically significantly higher amount in the bark (PPB) (7 ± 0.5), which also contained the highest amount of malic acid (356 ± 11) and the least of oxalic acid (1 ± 0.01). Fruits (PPF), on the other hand, had a high content of malic acid and citric acid.
Table 2. Content of phytochemicals compounds in P. padus leaves, bark, and fruits
Antioxidant potential of the tested parts of bird cherry was evaluated by spectroscopic methods (). Differences between the properties of anatomical parts of P. padus were shown. All extracts contained compounds reacting with the Folina-Ciocalteu reagent; however, their highest amount was found for the bark (PPB), where their content amounted to 136 c ± 9 mg GAE/g dw. The FRAP test also showed the highest activity for the bark extract (PPB) (379b ± 18 µM FeSO4/1 g dw) compared to the other two samples. The results of the study have been extended by the DPPH radical test. In this case, the highest value was obtained again for the bark (PPB) (9 c ± 0.03 mg TE/1 g dw). Only in the ABTS radical test, the bark activity (PPB) was the lowest (7a and ± 0.02 mg TE/g dw), and the highest was confirmed for the leaves (PPL) (10 c ± 0.1 mg TE/g dw).
Table 3. Antioxidant activity of P. padus leaves, bark, and fruit extracts with FRAP, DPPH, ABTS radicals, and total polyphenol content
The content of redox compounds in P. padus extracts was evaluated using square wave voltammetry (SWV). This very sensitive technique makes it possible to determine analytes at a very low concentration below 10–8 M.[Citation14] The voltamperograms in indicated the presence of one primary signal located in the +0.3 V region. It showed the greatest intensity for the leaf extract (PPL). The signal height was 26.065 µA and its area was 4.210 V × µA. The additional signal located in the +0.95 V region had a definitely lower current. Slightly lower SWV signals were detected in the fruit extract (PPF), in this case, an additional signal was visible at +0.8 V. Bark extract (PPB) had clearly the lowest electroactivity. In addition to the basic signal, it also contained a blurred signal located in the region from +0.4 to +0.9 V with a maximum at +0.73 V, which indicated the presence of a mixture of various redox compounds in the tested extract. The detailed characteristics of electrochemical parameters determined for the tested extracts are presented in . In addition, on the basis of our previous research,[Citation8] the electrochemical index, expressing the general electroactivity of the tested extracts in relation to the dry matter of plant material was calculated.
Table 4. Electrochemical parameters determined for extracts from P. padus.
Figure 1. Phenolic acids and flavonoids chromatograms
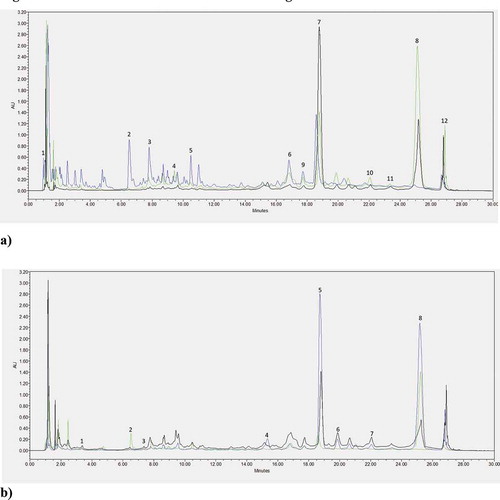
All obtained extracts were also tested for the activity against fungi and bacteria from Gram-positive and Gram-negative groups (). The highest activity against Gram-negative bacteria was recorded in bird cherry leaves (PPL) and the lowest in the bark (PPB). The leaves (PPL) were the most active against Klebsiella pneumoniae (19 ± 30 mm) and the fruits (PPF) were the most active against Pseudomonas aeruginosa and showed value 19 ± 3 mm in this case. The lowest growth inhibition of Gram-negative bacteria was observed for the bark (PPB) against Salmonella enteritidis (1 ± 0 mm). Similar to Gram-positive bacteria, the highest inhibition among Gram-positive bacteria was demonstrated for the leaves (PPL) and the weakest for the bark (PPB). Leaves (PPL) inhibited most effectively the growth of Listeria monocytogenes (24 ± 40 mm) and Enterococcus faecium (22 ± 30 mm). With respect to fungi, Candida utillis proved to be the most sensitive, for which the growth inhibition zone was 13 ± 2 when the fruit extract (PPF) was applied. The same part of P. padus (PPF) plant showed high activity against the remaining Aspergillus sp. (12 ± 1 mm) and Fusarium sp. (10 ± 0 mm) fungi. On the other hand, bird cherry bark (PPB) did not show any activity toward the tested microorganisms.
Table 5. Microbiological activity of P. padus leaves, bark, and fruit extracts
Principal Component Analysis (PCA) was used to observe possible relationships between the content of phytocompounds in bird cherry (P. padus) and antioxidant activity in its individual anatomical parts ( and ). The PCA projection (biplot) of the results of qualitative analysis in the system of the first two components (PC1 and PC2), responsible for over 60% composition variation, illustrated the diversity of samples in terms of the content of active components and the degree of influence of individual compounds on antioxidant activity. The close location of antioxidant activity vector, as measured by DPPH, FRAP, ferulic acid, naringenin, salicylic acid, sinapic acid, vitexin, or luteolin tests indicated a positive correlation of these indicators. In turn, the opposite position of the ABTS vectors to them indicated a negative correlation. Similarly, the opposite position of the SWV vector relative to apigenin, malic acid, catechin showed a negative correlation. Location of individual samples in different parts of the graph indicated the variability of anatomical parts of P. padus in terms of content of the analyzed compounds and antioxidant activity.
Discussion
Bird cherry is a not-so-popular plant in the context of its use in food technology or diet therapy, often referred to as forest weed. Available research results demonstrate that fruits such as bird cherry are a rich source of biologically active substances. In this work, the antioxidant potential of P. padus that results from the presence of polyphenolic compounds in individual parts of the plant has been demonstrated. Phenolic acids, whose highest amounts were found in P. padus leaves were, among others, responsible for this effect. Ferulic acid was dominant in all anatomical parts of the plant. This compound was also detected by other researchers[Citation4] in P. padus fruits (10.45 ± 3.65 mg/100 g FW); however, in our research also in fruits, it showed a much higher value (102 ± 8 mg/100 g dm). These authors pointed out that other compounds from this group, including chlorogenic acid, were also responsible for the antioxidant properties. Olszewska & Kwapisz also indicated a significant role of chlorogenic acid in the flowers of bird cherry, their result was 1,39–1,94% DW.[Citation3] In our work, the highest content of chlorogenic acid was in fruits and it was 109 ± 4 mg/100 g dm. Studies have shown that bird cherry contains a high quantity of flavonoids in its composition. The two dominant compounds in this group were quercetin and catechin, the highest values of which were found in the bark, where quercetin (599 ± 18 mg/100 g dm) and catechin (879 ± 43 mg/100 g dm). Other researchers have also marked these compounds in various anatomical parts of the bird cherry.[Citation3,Citation4] Quercetin has also been found in flowers (1.37–1.56% DW).
Plant products, primarily fruits, contain hydroxy acids, which are present in free form, unlike in vegetables. These acids protect organisms against microbes and unripe fruits contain their highest percentage. The study expanded research assessing the content of organic acids for individual parts of P. padus, and fruit extract contained their highest amount, which was consistent with the results of other Donno et al.[Citation4] and Mikulic-Petkovsek et al.[Citation15]
In addition to many reports on the antioxidant properties of polyphenols, much is also said about their bactericidal and bacteriostatic effects. Higher plants contain secondary metabolites active against bacteria and fungi. The study showed that anatomical parts of P. padus plant could have such properties where action against fungi and Gram-positive and Gram-negative bacteria was demonstrated (the highest antifungal effect was found toward Candida utillis). Gram-negative bacteria can cause many types of infections such as pneumonia, peritonitis, urinary tract infection, blood infection, wound infection, and meningitis.
The basic difference between Gram-positive and Gram-negative bacteria is that the former have a multilayered cell wall made of murein, and Gram-negative bacteria only have one layer. They are commonly treated with antibiotics, but they are not always effective. P. padus was also active against Gram-negative bacteria, such as Enterococcus faecium, for which the leaves showed the highest activity (22 ± 3 mm) Enterococcus faecium is responsible, among others, for urinary tract infections. The leaves were also active against Staphylococcus aureus causing, among others, skin infections, food poisoning, and arthritis. The effects of bird cherry on these two bacteria were also confirmed by Kumarasamy et al., [Citation16] who studied the seed of P. padus. Dichloromethane extracts of seeds also showed activity against Enterococcus faecium (1.0 mg/ml) and against Staphylococcus aureus (1.0x10−4 mg/ml) in methanol extracts.
Antioxidants in the body protect lipid membranes by donating electrons to free radicals, thereby stopping the cascade of the reaction chain.[Citation1] They are the most active group in the process of detoxification and protection of genes, which due to damage by radicals, can cause many types of cancers. Many studies are available in the literature that indicate the high antioxidant potential of plant materials, and this applies to fruits as well as leaves, bark, and flowers. The current work demonstrated that bird cherry contained compounds with anti-radical activity. A positive correlation between antioxidant activity and flavonoid content was shown. The results in the work have confirmed that bird cherry can be a source of antioxidants in a multidirectional diet.
Conclusion
The total antioxidant effect is difficult to predict, because the total antioxidant capacity is determined not only by the properties of individual compounds but above all by their mutual interaction. Therefore, it has been shown in the study that each of the anatomical parts of P. padus is rich in compounds from the group of polyphenols, which may also determine their diverse health-promoting properties. Based on the results, it has been found that it is often difficult to compare the antioxidant capacity using different methods. Studies have confirmed the variety of antioxidant mechanisms present in P. padus. Bird cherry bark was characterized by high antioxidant potential measured by Folin-Ciocalteu, ABTS and DPPH tests, resulting from the properties of active compounds present in them, and above all a rich content of phenolic acids and flavonoids. However, electrochemical methods demonstrated higher activity for leaves. In the antimicrobial test, the fruits were the most active against mold and fungi. Thus, it has been shown that P. padus bark, leaves, and fruits can be an inexpensive and pro-ecological source of antioxidants with multidirectional action.
Conflict of interest
The authors declare that they have no conflict of interest.
Acknowledgments
All authors contributed to the study conception and design. Material preparation, data collection, and analysis were performed by Aleksandra Telichowska, Joanna Kobus-Cisowska, Daria Szymanowska, and Kinga Stuper-Szablewska. The first draft of the manuscript was written by Aleksandra Telichowska, Piotr Szulc, Mariusz Tichoniuk, Marta Ligaj, and all authors commented on previous versions of the manuscript. All authors read and approved the final manuscript.
Additional information
Funding
References
- Lawson, M.; Jomova, K.; Poprac, P.; Kuca, K.; Musílek, K.; Valko, M. Free Radicals and Antioxidants in Human Disease. Nutritional Antioxidant Therapies: Treatments and Perspectives; Springer International Publishing, 2018; pp 283–305.
- Nestby, R. D. J. The Status of Prunus Padus L. (Bird Cherry) in Forest Communities Throughout Europe and Asia. Forests. Apr. 2020, 11 (5): 497.
- Olszewska, M. A.; Kwapisz, A. Metabolite Profiling and Antioxidant Activity of Prunus Padus L. Flowers and Leaves. Nat. Prod. Res. 2011, 25(12), 1115–1131. DOI: 10.1080/14786410903230359.
- Donno, D.; Mellano, M. G.; De Biaggi, M.; Riondato, I.; Rakotoniaina, E. N.; Beccaro, G. L. New Findings in Prunus Padus L. Fruits as a Source of Natural Compounds: Characterization of Metabolite Profiles and Preliminary Evaluation of Antioxidant Activity. Molecules. 2018, 23(4). DOI: 10.3390/molecules23040725.
- Hyun, T. K.; Kim, H. C.; Kim, J. S. In Vitro Screening for Antioxidant, Antimicrobial, and Antidiabetic Properties of Some Korean Native Plants on Mt. Halla, Jeju Island. Indian J. Pharm. Sci. 2015, 77 (6), 668–674. DOI: 10.4103/0250-474X.174984.
- Telichowska, A.; Kobus-Cisowska, J.; Ligaj, M.; Stuper-Szablewska, K.; Szymanowska, D.; Tichoniuk, M.; Szulc, P. Polyphenol Content and Antioxidant Activities of Prunus Padus L. And Prunus Serotina L. Leaves: Electrochemical and Spectrophotometric Approach and Their Antimicrobial Properties. Open Chem. Sep. 2020, 18(1), 1125–1135. doi: 10.1515/chem-2020-0121.
- Ye, Y.; Ji, J.; Sun, Z.; Shen, P.; Sun, X. Recent Advances in Electrochemical Biosensors for Antioxidant Analysis in Foodstuff. TrAC Trends Anal. Chem. Jan 01 2020, 122, 115718. Elsevier B.V. DOI: 10.1016/j.trac.2019.115718.
- Szczepaniak, O. M.; Ligaj, M.; Kobus-Cisowska, J.; Maciejewska, P.; Tichoniuk, M.; Szulc, P. Application for Novel Electrochemical Screening of Antioxidant Potential and Phytochemicals in Cornus Mas Extracts. CYTA - J. Food. Jan. 2019, 17 (1), 781–789. DOI: 10.1080/19476337.2019.1653378.
- Ligaj, M.; Tichoniuk, M.; Filipiak, M. Detection of Bar Gene Encoding Phosphinothricin Herbicide Resistance in Plants by Electrochemical Biosensor. Bioelectrochemistry. Nov 2008, 74 (1), 32–37. DOI: 10.1016/j.bioelechem.2008.03.003.
- Press, W. H., Flannery, B. P.; Teukolsky, S. A.; Vetterling, W. T. Savitzky-Golay Smoothing Filters. In Numerical Recipes in Fortran 77: The Art of Scientific Computing. Cambridge University Press. Cambrigde, UK, 1992.
- Kulczyński, B.; Kobus-Cisowska, J.; Kmiecik, D.; Gramza-Michałowska, A.; Golczak, D.; Korczak, J. Antiradical Capacity and Polyphenol Composition of Asparagus Spears Varieties Cultivated under Different Sunlight Conditions. Acta Sci. Pol. Technol. Aliment. 2016, 15 (3), 267–279.
- Kobus-Cisowska, J.; Szulc, P.; Szczepaniak, O.; Dziedziński, M.; Szymanowska, D.; Szymandera-Buszka, K.; Goryńska-Goldmann, E.; Gazdecki, M.; Telichowska, A.; Ligaj, M.; et al. Variability of Hordeum Vulgare L. Cultivars in Yield, Antioxidant Potential, and Cholinesterase Inhibitory Activity. Sustainability. 2020 Mar, 12(5), 1938. doi: 10.3390/su12051938.
- O’Sullivan, A. M.; O’Callaghan, Y. C.; O’Connor, T. P.; O’Brien, N. M. Comparison of the Antioxidant Activity of Commercial Honeys, before and after In-vitro Digestion. Polish J. Food Nutr. Sci. 2013, 63 (3), 167–171. DOI: 10.2478/v10222-012-0080-6.
- Lovric, M. Square-Wave Voltammetry, In: Electroanalytical Methods. Guide to Experiments and Applications; Scholz, F. Ed.; Springer-V: Berlin, 2002.
- Mikulic-Petkovsek, M.; Stampar, F.; Veberic, R.; Sircelj, H. Wild Prunus Fruit Species as a Rich Source of Bioactive Compounds. J. Food Sci. 2016, 81(8), C1928–C1937. DOI: 10.1111/1750-3841.13398.
- Kumarasamy, Y.; Cox, P. J.; Jaspars, M.; Nahar, L.; Sarker, S. D. Comparative Studies on Biological Activities of Prunus Padus and P. Spinosa. Fitoterapia. 2004, 75(1), 77–80. DOI: 10.1016/j.fitote.2003.08.011.