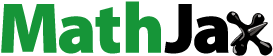
ABSTRACT
This study was aimed to investigate the effect of low-temperature vacuum heating (LTVH; versus the traditional thermal method– TC - 100°C boiling water) on water content/distributions, microstructure, and tenderness of sturgeon fillets. Although all fillets were well done, those fillets heated at lower temperature/time showed higher moisture content (P< .05). Furthermore, microstructure by paraffin section and shear force results also indicated LTVH70-30 (LTVH method; 70°C, 30 min) shown the most similar to TC fillets, the less firm and less juicy. Oppositely, LTVH60-15/30 (LTVH method, 60°C, 15/30 min) showed the best tenderness/juiciness among all fillets. Therefore, the LTVH method is agood alternative to the TC for fish processing in the catering industry, with tenderness/juiciness gains.
Graphical abstract of sturgeon fillets by low temperature vacuum heating GA

Introduction
Fish are the main superb source of animal protein, with ahigh nutritive value owing to their favorable essential amino acid composition which is necessary in adiet .[Citation1,Citation2] Although fish are sometimes eaten in raw (e.g., sashimi), fish are usually consumed after cooking in boiling water, steaming, frying, or subjected to other heating treatments. The main advantages of heating treatment are sterilization, which ensures safe consumption, increased digestibility, [Citation3] enhanced flavor and taste, and the increase shelf life .[Citation4] As aculinary tradition, boiling is popular in China, the USA, Japan, and other countries and has accepted the most attention in the context of home cooking .[Citation5]
In addition to their valuable caviar, sturgeons are also popular for their boneless flesh with excellent taste; in fact the sturgeon flesh accounts for approximately 300 g/kg skinless fillets .[Citation6] However, in contrast to marine fish and animal meat, sturgeon, as afreshwater fish, has asmaller muscle fiber diameter, less connective tissue, and atender taste .[Citation7] This means that the sturgeon fillets have poor thermal tolerance and their fiber muscles are much more easily broken during thermal processing. The natural features of the protein molecules are denatured during thermal treatment, and the reactive groups of proteins are exposed, resulting in loss of water, soluble vitamins, and proteins, and ultimately leading to changes in the surface and taste of food .[Citation3] Anumber of studies exploring the effects of thermal treatments on the eating quality of cooked fish occurring by protein denaturation have been reported.Citation8, Citation9, Citation10 Of note, the changes during processing depended on the processing method used and the heating time and temperature. For instance,Citation11reported that the heat treatment at high temperatures causes protein oxidation overhand, which consequently leads to changes in the physicochemical and organoleptic characteristics of food, including texture deterioration induced by the loss of water-holding capacity, and alteration of flavor. However, thermal treatment of food at lower heating temperatures usually requires alonger heating time, which is generally not appropriate for industrial applications because of the lower productivity of this cooking method .[Citation12]
Low-temperature vacuum heating (LTVH) is similar to the traditional domestic boiling method. Samples are heated in boiling water, but at mild temperatures (under 100°C) vis the use of special self-made equipment that allows controllable vacuum conditions. Our previous study showed that the LTVH method can reduce the oxidation of protein and lipid in fillets, compared to those observed in the context of traditional domestic cooking (100°C boiling water) .[Citation13] Additionally, the LTVH fillets had agreat shelf life after 7 days storage, performing abetter storage quality than the control groups thermal treatment at 100°C boiling water .[Citation14] However, there is still limited data available on the water-holding capacity and tenderness of sturgeon fillets after processing via different heating time/temperature combinations.
Hence, the aim of this study was to evaluate the effect of heating time/temperature combinations by LTVH method (using once again the traditional heating method as the control condition), on water-holding capacity (WHC), water distributions, and the microstructure of sturgeon fillets. Abetter understanding of the water mobility and fiber structure changes induced in fillets during heat-mediated processing is essential to elucidate their role in the protein matrix structure and for the development of new healthy aquatic products.
Materials and methods
Sample preparation
Frozen Russian sturgeons, male, 3year old and 3 ± 1kg weight, provided by Quzhou Sturgeon Aquatic Food Technology Development Co. Ltd, Zhejiang, China, were transported to the laboratory within 2 h. The middle part of the sturgeon was cut into fillets (approximately 1.0cm thickness and 65 to 75 g weight) after thawing with running water for half anhour. The fillets were bathed without any package in the boiling water using the self-made equipment under controllable vacuum conditions and randomly divided into 5 groups: traditional thermal method (TC, fillets were treated at 100°C– boiling water– for 15 min under normal pressure), LTVH60 (including LTVH60-15 and LTVH60-30, fillets were treated at 60°C for 15 or 30 min, respectively, under a− 812 mbar pressure), and LTVH70 (including LTVH70-15 and LTVH70-30, fillets were treated at 70°C for 15 or 30 min, respectively, at a− 700 mbar pressure). After thermal treatment, the fillets were collected, placed into with plastic bags, and cooled to room temperature in an ice bath for subsequent quantitative and qualitative analyses.
Cooking loss rate
The cooking loss rate was calculated based on the weight loss (before versue) after heating treatment. Determinations were carried out in triplicate. The performed equation is shown to be the following equation:
where W1 is the mass (g) of the sturgeon fillet before heating and W2 is the mass (g) of the sturgeon fillet after heating.
Moisture content
The moisture content was determined based on the weight loss after drying 5 g of heated fillet in an assisted air circulation oven (GZX-9140MBE, Boxun Industrial Co., Ltd., Shanghai, China) at 105°C to aconstant weight (differences of weight limited to 2mg). Determinations were duplicated in triplicate. The data were calculated with the following equation:
Where m1 is the mass (g) of the sturgeon fillet before drying and m2 is the mass (g) of the sturgeon fillet after drying to aconstant weight.
Water-holding capacity [WHC)
The WHC of fillets was determined according to the method described by,Citation15 using an high-speed refrigerated centrifuge (H2050R, Xiangyi Laboratory Instrument Development Co., Ltd, Hunan, China]. All determinations were carried out three times, independently. The following equation was used for the determination of the WHC:
Where C1 is the mass (g) of the sturgeon fillet before centrifugation (1000 × g for 10 min at 4°C) and C2 is the mass (g) of the sturgeon fillet after centrifugation (1000 × g for 10 min at 4°C), g.
Low-field nuclear magnetic resonance [LF-NMR)
The water distribution in sturgeon fillet subjected to thermal-treatment was determined via LF-NMR relaxation experiments according to the method of proposed by,Citation16Briefly, transverse relaxation time (T2] measurements were performed using aMeso MR23-060 V–I nuclear magnetic resonance analysis system (Niumag Electric Corporation, Shanghai, China). Astandard copper sulfate solution was placed in the RF coil (25mm diameter) center of the magnet case, under the following condition: magnet temperature of 32°C, the magnet strength of 0.5 T. Using the FID sequence, the center frequency SFO1 was 23.20 MHz, automatically find the 90° and 180° pulse widths, determined the 90° pulse time (P1) was 30.00 μs, and the 180° pulse time (P2) was 56.00 μs. T2 values were measured by collecting CPMG (Carr-Purcell-Meiboom-Gill) decay signals with the sampling parameters are set as follows: repeat sampling waiting time (Tw) of 2000 ms, analog gain (RG1) of 20, digital gain (DRG) of 2, pre-amplification gain (PRG) of 1, accumulation number (NS) of 8, echo number (NECH) of 1000, and delay (DL1) of 0.1 ms. The Multi-Exp Inv Analysis software (Niumag Electric Corporation, Shanghai, China) was used for the distributed multi-exponential fitting of the CPMG decay curves and visualization of the lateral relaxation spectrum of the fillets. Three independent scan repetitions were conducted for each group, and the average value was recorded.
Proton density imaging
The proton density images of fillets subjected to thermal treatment were acquired by using aMINI MR-60 device [Niumag Electric Corporation, Shanghai, China) according to the method of,Citation17and with minor modifications. The device was operated at afrequency of 23 MHz and 32°C. The main parameters were set as follows: repetition time (TR] of 500 ms, viewing field of 50mm×50mm, and echo time (TE) of 19.0 ms. The final images were obtained by using the respective image processing software [Shanghai Niumag Corporation, Shanghai, China).
Extraction of myofibrillar proteins
The extraction and calculation of myofibrillar proteins was performed as described by,Citation13The determinations were repeated three times. The extraction rate of myofibrillar proteins was calculated as follows (Citation16):
where E1 is the quantity (mg) of myofibrillar proteins extracted from heated sturgeon fillet and E2 is the quantity [mg) of myofibrillar protein extracted from raw sturgeon fillet.
Light microscopy microstructural analysis
The microstructures of the heated fillet were investigated following the method of reported by.Citation18 Briefly, the fillet samples were fixed in fixative (including 4% paraformaldehyde], embedded in paraffin, and then cut (5–8 μm thick sections) using amicrotome (RM2016, Leica Instruments Co., Ltd., Shanghai, China). After de-paraffinization with xylene, the samples were stained with Ehrlich’s hematoxylin for 5 min and dehydrated in absolute ethanol for 5 min twice. The microstructures of the samples were observed and photographed using alight microscope (Nikon Eclipse E100, Nikon Co., Tokyo, Japan) coupled to an imaging system [Nikon DS-U3, Nikon Co., Tokyo, Japan).
Shear force analysis
According to the method reported by,Citation19but with minor modifications, the shear force analysis in the context of sturgeon fillets was performed using atexture analyzer (TA.XT Express, Stable Micro Systems, Surrey, UK] combined with aWarner Bratzler shearing device and aspeed of 5cm/min and adistance of 25cm. Six replicates were used per fillet.
Statistical analysis
All results are expressed as mean values ± standard deviation. Duncan’s multiple comparison test was used to determine statistical significance, set at P< .05. Pearson’s correlation analysis was performed to understand the relationships between the parameters.
Results and discussion
Extraction rate of myofibrillar proteins
Myofibrillar proteins are the most abundant proteins in the sturgeon (Acipenser gueldenstaedti) fillets, constituting approximately 50–55% of the total protein content .[Citation13] Therefore, changes in the myofibrillar proteins during thermal treatment play an important role in the alteration of the characteristics of sturgeon fillets. In daily life, we usually judge the readiness of cooked fillets based on their visual appearance and color. For instance, well-done fillets are pale, grayish-brown, and dry. Of note,,Citation16reported that the abalones were effectively cooked when the extraction rate of myofibrillar proteins was limited to less than 10%. As shown in , the extraction rates of myofibrillar proteins in all heat-treated fillets were below 10%. These results suggest that all fillets (LTVH and TC) were well done. Of note, the extraction rates of LTVH fillets decreased with the increase in the heating temperature (P< .05) and heating time (P> .05) (). Therefore, altogether, these results suggest that increased heating temperatures have agreater impact on decreased myofibrillar proteins than prolonged heating time.Citation20 reported that the myofibrillar proteins were sensitive to heating temperatures and get denatured during thermal treatment, resulting in protein aggregation and decreased extraction rate. Notably, as shown in , there were no significant differences between the extraction rates of the LTVH70-15, LTVH70-30, and TC groups. The denaturation of myofibrillar proteins may have been completed at the respective temperatures.,Citation21reported that proteolysis and aggregation occur together during thermal treatment. The insignificant difference in LTVH70 and TC was presumably due to the combination of protein aggregation and degradation at high temperatures.,Citation22,and Citation23also reported that protein digestibility decreased as the heating temperature increased, which was attributed to protein aggregation. Overall, in our study, LTVH60 fillets exhibited better protein retention than both LTVH70 fillets and TC fillets.
Table 1. Changes on the extraction rate of myosfibrillar protein, cooking loss, moisture content and shears force of sturgeon fillets by low temperature vacuum heating
Cooking loss rate, moisture content and WHC
The cooking loss rate, playing an important role in food processing, is responsible for the juiciness and yield of final products .[Citation24] Changes in the cooking loss rates and moisture contents of the different groups of fillets in this study are presented in . The heating time played acritical role in cooking loss rates. When the fillets were heated at 60°C for 15 min (LTVH60-15), the cooking loss rate was 27.56% ± 0.53%; while significantly increased to 31.26%±1.01% at LTVH60-30 (P< .05). However, there is no significant difference between the cooking loss rate of LTVH70-15 and LTVH70-30 (P> .05). Notably, the highest value of cooking loss rate was observed at TC fillets, and significantly higher than those heated by LTVH method (P< .05, ). Some water-soluble proteins were lost with the water during cooking process, [Citation25] which enhanced the cooking loss in the TC fillets. The results illustrate the advantages of the LTVH method compared with TC in serving better juiciness and yield of final products.
As presented in , the moisture content of the heated samples tended to decline with the heating time (P< .05) and heating temperature (P> .05) in fillets processed via the LTVH method. However, there was no significant difference between the WHC of LTVH and TC fillets (P> .05). There are three main states of water in muscles: bound water, immobilized water, and free water [introduced in moisture distribution).,Citation26reported that immobilized water and free water, entrapped in the myofibrillar network or within the space between the thick and thin filaments, are sensitive to thermal treatment. It is, therefore, not surprising that increased the heating temperature or prolonging the heating time leads to water evaporation, and consequently, shrinking of myofibrils proteins (due to the weakened-binding forces between proteins and water] .[Citation27] In line with this notion, here, the conducting the boiling process at low temperature or for less time persevered more water in the fillet structures; the LTVH60-15 samples showed the highest moisture content and WHC (68. ± 0.19%, 75. ± 1.66%, respectively; ). We hypothesize that the capillary water loss during thermal treatment was one of the reasons for the reduction of free water in fillets, as reported by others .[Citation28] Overall, our results suggest that LTVH fillets treated at lower temperature had batter moisture content than TC fillets, probably due to the preservation of protein solubility.
Moisture distribution
Moisture distribution and migration are influenced by the heating temperature and heating time during thermal treatment. The different of T2 relaxation spectra obtained in this study are shown in ; of note, these data exhibit astrong correlation with water-holding capacity .[Citation29] As shown in , three peaks, T21, T22, and T23, are observed in the sturgeon fillets processed using low-temperature vacuum heating, indicating three distinct water states; T21 (ranging from 0 to 10 ms), T22 (ranging from 25 to 35 ms) and T23 (ranging from 140 to 1000 ms) were assigned to bound water, immobile water, and free water, respectively (). These results are in line with previous studies reporting that the existence of three water states in heated muscle tissues, [Citation30,Citation31] with apredominance of immobile water.
Figure 1. Changes on NMR T2 relaxation time of sturgeon fillets by low temperature vacuum heating
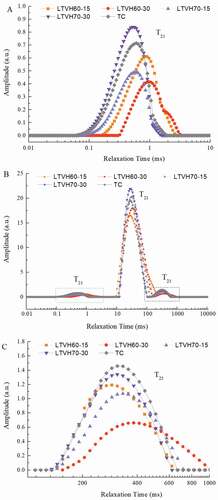
As shown in , the relaxation time T21 shifted downward with the heating temperature and heating time, and its amplitude of T21 declined. However, there was no significant difference in T21, except for LTVH60-30 (, P> .05). As mentioned above, the relation time T21 represents bound water components, which bind to protein molecules via hydrogen bonds, having the lowest mobility in fillet muscles, [Citation32] which is responsible for its resistance to thermal treatment .[Citation26] Interestingly, Pearson’s correlation analysis showed that there was avery significant positive correlation (R = 0.910, P< .01, ) between the relaxation time T21 and the extraction rate of myofibrillar proteins in heated fillets. As shown in , A21, corresponding to the peak area of T21, increased with the heating temperature and heating time [P> .05), suggesting that thermal treatment indeed influences protein–water interactions.,Citation33also reported that the reduction in protein solubility was responsible for the decreased water-holding capacity due to weakening of the protein–water interactions and strengthening of the protein–protein interactions. In line with this nation, T21 of the LTVH70 fillets and TC fillets changed very little while that of LTVH60 fillets changed much more as myofibrillar proteins varied.
Table 2. Changes on NMR parameters obtained from sturgeon fillets by low temperature vacuum heating
Table 3. Pearson’s correlation measurement of indicators from sturgeon fillets by low temperature vacuum heating
As shown by the NMR date, immobilized water, accounting for more than 90% of moisture, was the highest in fillets after thermal treatment (]. Of note, the relaxation time T22 and T23 decreased, as the relaxation components shifted to the left in , indicating that water mobility weakened as the heating temperature/time increased. Notably, the peak area proportion of immobilized water (A22) was inclined, while that of free water [A23) increased with both the heating temperature and time.,Citation34reported that changes in water may be correlated with the shrinkage and toughening of the fillet structure and cooking loss due to heating. In our study, the correlation analysis revealed avery significant positive correlation (R = 0.779, P< .01] between the peak area proportion A23 and the cooking loss rate in heated fillets. These results suggest that immobilized water was transformed into free water, and then released into the broil during thermal treatment, causing an increase in the cooking loss rate.
Magnetic resonance imaging (MRI) is anoninvasive technique that has been used to analyze the water distribution in food .[Citation35] In this study, the distribution and migration patterns of water in fillets are also presented in the form of MRI-derived proton density images (; the high proton densities are highlighted in red, while low proton densities are highlighted in blue). From the red zone ratios of these images, it is clear that the thermal treatment had asignificant influence on the content and distribution of water in the fillets. Notably, there were some red lines obviously present in fillets (), demarking the connective tissue wrapping the myofibrils in fillet structures.,Citation36speculated that the proteins in the connective tissues can restore main water loss during thermal treatment. Our data suggests that the water, existing between muscle filaments, myofibrils, and membranes, did not easily flow because of the strong interactions with proteins. As shown in , the moisture content decreased when the heating temperatures increased. However, no significant difference was observed in fillets heated via the LTVH method at the same temperature but during different time, which is in line with the results of T2 relaxation. Of note, TC fillets showed fewer red zones than LTVH fillets, which may be due to the changes in the muscle tissues in the context of high-temperature boiling water.
Figure 2. Changes on proton density images of sturgeon fillets by low temperature vacuum heating
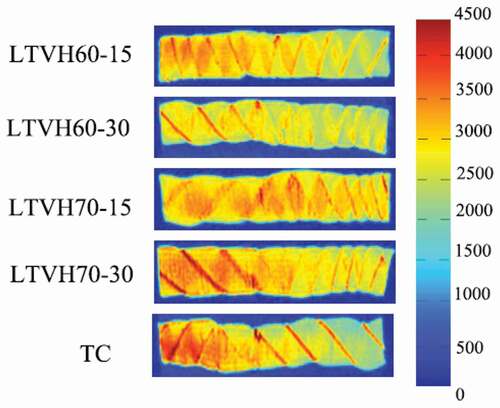
Microstructure
The microstructural changes of fillets subjected to different thermal treatments were observed on transverse and longitudinal sections of paraffin-embedded tissues via light microscopy are shown in . The tissue structure of the fillets was significantly affected by thermal treatment. Denaturation and shrinking of myofibrillar proteins and connective tissues were occurred during the heating process, causing achange in the tissue microstructure; gaps were clearly observed in fillets, as reported elsewhere .[Citation37] However, fillets showed better tissue integrity after low-temperature heating processing (LTVH method). As shown in , the LTVH60-15 fillets showed the most intact tissue architecture; in contrast, wider intermyofibrillar spaces and expansion of muscle fibers, were clearly observed in the LTVH60-30 fillets. The same trend was observed in the LTVH70-15 fillets versus LTVH60-30 fillets. However, when the heating time was increased to 30 minin the context of the 70°C LTVH processing, some breaks were observed in the muscle fibers [LTVH70-30). Notably, the myofibril bundles of the TC fillets were severely broken with adissolved myofibrillar package and larger gaps. These results are in line with the results of the study by,Citation38which reported that treatment at high temperatures caused shrinking and dissolution of the myofibrillar fibers, resulting in the re-arrangement of broken myofibril proteins. Of note, large intercellular spaces were observed in LTVH70-30 fillets and TC fillets and to agreater extent in the latter, in where some solutes produced after the dissolution/degradation of cellular networks were fulfilled. Altogether, these results indicate that fillets treated using the LTVH method have better tenderness and WHC ( and4].
Figure 3. Changes on microstructure graphs of transverse (A) and longitudinal (a) sections in sturgeon fillets by low temperature vacuum heating (400ⅹ)
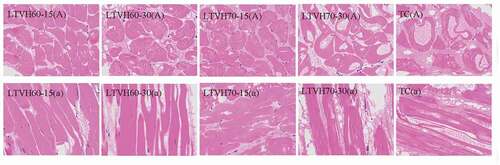
Shear force
Tenderness is one of the most critical characteristics of fillets after thermal treatment. As shown in , thermal treatment, especially the heating temperature had asignificant influence on the shear force value of the fillets. With respect to the LTVH60-15 and LTVH60-30 fillets (treated at 60°C with −812 mbar), the low heating temperature led to low shear force values, which consequently leading to abetter tenderness of the fillets. Structural alterations of proteins are associated with simultaneous changes in the physicochemical properties of the water within the fillet structure .[Citation39] An earlier study had reported that changes in shear force values were mainly related to the changes in the tissue strength and water-holding capacity, which was responsible by the changes in myofibrillar .[Citation40] As mentioned previously, fillets were treated at low heating temperatures, which led to the better preservation of water and the tissue structures, resulting in the tenderization of LTVH60-15 and LTVH60-30 fillets.,Citation41investigated the tenderness of meat after thermal processing (also using the Warner-Bratzler shearing device) and their results suggested that the tenderness of meat heated at 60°C was better than that of meat heated at 50°C. However, fillets treated at 70°C under −700 mbar or at 100°C under normal pressure, showed higher shear force values (non-statistically difference among the LTVH70-15, LTVH70-30, and TC groups). These results are in line with aforementioned results that the muscle tissues were damaged severely occurring to the decline in water-holding capacity during high processing temperature.
Figure 4. Changes on shear force of sturgeon fillets by low temperature vacuum heating

Conclusion
Although all fillets investigated in this study were well-done fillets (extraction rate of myofibrillar proteins was below 10%), different heating time/temperature combinations, resulted in different characteristics in sturgeon fillets. Due to treated at lower temperature and vacuum condition with boiling water, LTVH fillets showed better results in protecting the thermal loss in myofibrillar proteins and moisture. As the results of integrity of muscle tissue and moisture retention capacity, LTVH60 fillet performed best tenderness values out of all of the fillets via thermal processing. TC fillets, heated at 100°C boiling water under normal pressure, showed apredominant loss of structure at the microstructural level. This was closely linked to lower firmness and juiciness, which consumers perceived as undesirable. Therefore, the application of the LTVH method is agood alternative to the conventional processing fish (below 100°C boiling water) in the catering industry; notably, the final product is expected to be higher quality, with better tenderness and lower cooking loss. This would benefit both customers and the food processing industry.
Highlights
The comparison between LTVH method and domestic heating at 100°C boiling water.
The effects on water-holding capacity and microstructure of fillets investigated.
The water distribution in fillet was observed by LF-NMR relaxation experiment.
All fillets were well done and LTVH60 samples preserved more moisture.
LTVH method can be served to achieve better tenderness and juicy fillets.
Acknowledgments
This work was supported by National Key R&D Program of China (Grant No.2018YFD0400600), Department of Education of Zhejiang Province (Grant No. Y201924135), and Zhejiang Provincial Natural Science Foundation (Grant No. LQ21C200004). And thanks for Quzhou Sturgeon Aquatic Food Technology Development Co. Ltd, Zhejiang China supported sturgeons for this study. The authors declare no conflict of interest.
Additional information
Funding
References
- Larsen,R.; Stormo, S.K.; Dragnes, B.T.; Elvevoll, E.O. Losses of Taurine, Creatine, Glycine and Alanine from Cod (Gadus Morhua L.) Fillet during Processing. J.Food Compost. Anal. 2007, 20(5), 396–402. DOI: https://doi.org/10.1016/j.jfca.2006.10.001.
- Wang,X.; Muhoza,B.; Wang,X.; Feng,T.; Zhang,X. Comparison between Microwave and Traditional Water Bath Cooking on Saltiness Perception, Water Distribution and Microstructure of Grass Crap Meat. Food Res. Int. 2019, 125, 108521.
- Alipour, H.J.; Shabanpour,B.; Shabani,A.; Mahoonak, A.S. Effects of Cooking Methods on Physico-chemical and Nutritional Properties of Persian Sturgeon Acipenser Persicus Fillet. Int. Aquat. Res. 2010, 2(1), 15–23.
- Garcia-Arias, M.T.; Alvarez-Pontes,E.; Garcia-Linares, M.C.; Garsia-Fernandez, M.C.; Sanchez, F.J. Cooking-freezing-reheating (CFR) of Sardine (Sardina Pilchardus) Fillets. Effect of Different Cooking and Reheating Procedures on the Proximate and Fatty Acid Compositions. Food Chem. 2003, 83(3), 349–356. DOI: https://doi.org/10.1016/S0308-8146(03)00095-5.
- Liu,S.; Yu,X.; Fukuoka,M.; Sakai,N. Modeling of fish Boiling under Microwave Irradiationfi. J.Food Eng. 2014, 140, 9–18. DOI: https://doi.org/10.1016/j.jfoodeng.2014.04.017.
- Nikoo,M.; Ghomi, M.R. Influence of Frying Oil Type and Chill Storage on the Nutritional Quality of Farmed Great Sturgeon (Huso Huso). Revista de Nutrio. 2013, 26(1), 67–74. DOI: https://doi.org/10.1590/S1415-52732013000100007.
- Jiang,Q.; Han,J.; Gao,P.; Yu,L.; Xu,Y.; Xia,W. Effect of Heating Temperature and Duration on the Texture and Protein Composition of Bighead Carp (Aristichthys Nobilis) Muscle. Int. J.Food Prop. 2018, 21(1), 2110–2120. DOI: https://doi.org/10.1080/10942912.2018.1489835.
- Hassoun,A.; Heia,K.; Lindberg, S.K.; Nilsen,H. Spectroscopic Techniques for Monitoring Thermal Treatments in Fish and Other Seafood: AReview of Recent Developments and Applications. Foods. 2020, 9(6), 767. DOI: https://doi.org/10.3390/foods9060767.
- Kong, F.B.; Oliveira,A.; Tang,J.; Rasco,B.; Crapo,C. Salt Effect on Heat-Induced Physical and Chemical Changes of Salmon Fillet (O-gorbuscha). Food Chem. 2008, 106(3), 957–966. DOI: https://doi.org/10.1016/j.foodchem.2007.07.008.
- Schubring,R.;. Comparative Study of the DSC Pattern, Color, Texture and Water-binding Capacity of Rainbow Trout Muscle during Heating. J.Food Process. Preserv. 2008, 32(2), 190–218. DOI: https://doi.org/10.1111/j.1745-4549.2007.00174.x.
- Hu,L.; Ren,S.; Shen,Q.; Chen,J.; Ye,X.; Ling,J. Proteomic Study of the Effect of Different Cooking Methods on Protein Oxidation in Fish Fillets. RSC Advance. 2017, 7(44), 27496–27505. DOI: https://doi.org/10.1039/C7RA03408C.
- Ammar, K.M.; Ali,S.; Yang,H.; Ali, K.A.; Ahmad,Z.; Keith, T.R.; Zhou,G. Improvement of Color, Texture and Food Safety of Ready-to-eat High Pressure-heat Treated Duck Breast. Food Chem. 2018, 277(30), 646–654. doi: https://doi.org/10.1016/j.foodchem.2018.11.006.
- Cai,W.; Wei,J.; Chen,Y.; Dong,X.; Zhang,J.; Bai,F.; Zheng,L.; Shi,Y. Effect of Low Temperature Vacuum Heating on Physicochemical Property of Sturgeon (Acipenser Gueldenstaedti) Fillets. J.Sci. Food Agric. 2020, 100(12),4583-4591. DOI:https://doi.org/https://doi.org/10.1002/jsfa.10517.
- Shen, S.K.; Chen, Y.W.; Dong, X.P.; Liu, F.J.; Cai, W.Q.; Wei, J.L.; Bai,F.; Shi, Y.G.; Li,P.; Wang, Y.R. Changes in Food Quality and Microbial Composition of Russian Sturgeon (Acipenser Gueldenstaedti) Fillets Treated with Low Temperature Vacuum Heating Method during Storage at 4°C. Food Res. Int. 2020, 138, 109665. DOI:https://doi.org/https://doi.org/10.1016/j.foodres.2020.109665.
- Gao,R.; Wijaya, G.Y.; Yu,J.; Jin,W.; Bai,F.; Wang,J.; Yuan,L. Assessing Gel Properties of Amur Sturgeon (Acipenser Schrenckii) Surimi Prepared by High-temperature Setting (40 °C) for Different Durations. J.Sci. Food Agric. 2020, 100(7), 3147–3156. DOI: https://doi.org/10.1002/jsfa.10349.
- Dong,X.; Hou,Y.; Wang,Y.; Xu,X.; Wang,K.; Zhao,M.; Orakash,S.; Yu,C. Effect of Temperature-time Pretreatments on the Texture and Microstructure of Abalone (Haliotis Discus Hanai). J.Texture Stud. 2018, 49(5), 503–511. DOI: https://doi.org/10.1111/jtxs.12354.
- Li,X.; Wang,H.; Mehmood,W.; Qian,S.; Sun,Z.; Zhang,C.; Blecker,C. Effect of Storage Temperatures on the Moisture Migration and Microstructure of Beef. J.Food Qual. 2018, (2018, 1–8.
- Zhuang,X.; Jiang,X.; Han,M.; Kang, Z.L.; Zhao,L.; Xu, X.L.; Zhou, G.H. Influence of Sugarcane Dietary Fiber on Water States and Microstructure of Myofibrillar Protein Gels. Food Hydrocolloids. 2016, 57, 253–261. DOI: https://doi.org/10.1016/j.foodhyd.2016.01.029.
- Fogaca,F.; Trinca, L.A.; Bombo, A.J.; Silvia Sant, A.L. Optimization of the Surimi Production from Mechanically Recovered Fish Meat (Mrfm) Using Response Surface Methodology. J.Food Qual. 2013, 36(3), 209–216. DOI: https://doi.org/10.1111/jfq.12019.
- Tornberg,E.;. Effects of Heat on Meat Proteins: Implications on Structure and Quality of Meat Productsff. Meat Sci. 2005, 70(3), 493–508. DOI: https://doi.org/10.1016/j.meatsci.2004.11.021.
- Sankar, T.V.; Ramachandran,A. Thermal Stability of Myofibrillar Protein from Indian Major Carps. J.Sci. Food Agric. 2005, 85(4), 563–568. DOI: https://doi.org/10.1002/jsfa.1956.
- Wen,S.; Zhou,G.; Li,L.; Xu,X.; Li,C. Effect of Cooking on in Vitro Digestion of Pork Proteins: APeptidomic Perspective. J.Agric Food Chemi. 2015, 63(1), 250–261. DOI: https://doi.org/10.1021/jf505323g.
- Liu,F.; Dong,X.; Shen,S.; Shi,Y.; Ou,Y.; Cai,W.; Chen,Y.; Zhu,B. Changes in the Digestion Properties and Protein Conformation of Sturgeon Myofibrillar Protein Treated by Low Temperature Vacuum Heating during in Vitro Digestion. Food Funct. Online. 2021. doi: https://doi.org/10.1039/d0fo03247f.
- Dominguez-Hernandez,E.; Salaseviciene,A.; Ertbjerg,P. Low-temperature Long-time Cooking of Meat: Eating Quality and Underlying Mechanisms (A Review). Meat Sci. 2018, 143, 104–113. DOI: https://doi.org/10.1016/j.meatsci.2018.04.032.
- Oz,F.; Zikirov,E. The Effects of Sous-vide Cooking Method on the Formation of Heterocyclic Aromaticamines in Beef Chopsff. LWT - Food Sci. Technol. 2015, 64(1), 120–125. doi: https://doi.org/10.1016/j.lwt.2015.05.050.
- McDonnell, C.K.; Allen,P.; Duggan,E.; Arimi, J.M.; Casey,E.; Duane,G.; Lyng, J.G. The Effect of Salt and Fibre Direction on Water Dynamics, Distribution and Mobility in Pork Muscle: ALow Field NMR Study. Meat Sci. 2013, 95(1), 51–58. DOI: https://doi.org/10.1016/j.meatsci.2013.04.012.
- Davey, C.L.; Niederer, A.F.; Graafhuis, A.E. Effects of Ageing and Cooking on the Tenderness of Beef Muscle. J.Sci. Food Agric. 1976, 27(3), 251–256. DOI: https://doi.org/10.1002/jsfa.2740270309.
- Wan,J.; Cao,A.; Cai,L. Effects of Vacuum or Sous-vide Cooking Methods on the Quality of Largemouth Bass (Micropterus Salmoides)ff. Int. J.Gastronomy Food Sci. 2019, 18, 100181. DOI: https://doi.org/10.1016/j.ijgfs.2019.100181.
- Zheng, H.B.; Xiong, G.Y.; Han, M.Y.; Deng, S.L.; Xu, X.L.; Zhou, G.H. High Pressure/thermal Combinations on Texture and Water Holding Capacity of Chicken Batters. Innovative Food Sci. Emerging Technol. 2015, 30, 8–14. DOI: https://doi.org/10.1016/j.ifset.2015.06.002.
- Zhou,F.; Dong,H.; Shao, J.H.; Zhang, J.L.; Liu, D.Y. Effect of Chopping Time and Heating on1HNuclear Magnetic Resonance and Rheological Behavior of Meat Batter Matrix. Anim. Sci. J. 2017, 89(4), 695–702. DOI: https://doi.org/10.1111/asj.12971.
- Zhang,M.; Fangfei,L.; Diao,X.; Kong,B.; Xia,X. Moisture Migration, Microstructure Damage and Protein Structure Changes in Porcine Longissimus Muscle as Influenced by Multiple Freeze-thaw Cycles. Meat Sci. 2017, 133, 10–18. DOI: https://doi.org/10.1016/j.meatsci.2017.05.019.
- Sánchez-Alonso,I.; Moreno,P.; Careche,M. Low Field Nuclear Magnetic Resonance (LF-NMR) Relaxometry in Hake (Merluccius Merluccius, L.) Muscle after Different Freezing and Storage Conditions. Food Chem. 2014, 153, 250–257. DOI: https://doi.org/10.1016/j.foodchem.2013.12.060.
- Jiang,Q.; Nakazawa,N.; Hu,Y.; Osako,K.; Okazaki,E. Microstructural Modification and Its Effect on the Quality Attributes of Frozen-thawed Bigeye Tuna (Thunnus Obesus) Meat during Salting. LWT - Food Sci. Technol. 2019, 100, 213–219. DOI: https://doi.org/10.1016/j.lwt.2018.10.070.
- Shaarani, S.M.; Nott, K.P.; Hall, L.D. Combination of NMR and MRI Quantitation of Moisture and Structure Changes for Convection Cooking of Fresh Chicken Meat. Meat Sci. 2006, 72(3), 398–403. DOI: https://doi.org/10.1016/j.meatsci.2005.07.017.
- Mehmood,W.; Qian,S.; Zhang,C.; Li,X. Biophysical Properties and Volumetric Changes in Breast Meat of Broilers and Yellow-feathered Chicken as Affected by Cooking Process. Int. J.Food Prop. 2019, 22(1), 1935–1951. DOI: https://doi.org/10.1080/10942912.2019.1696361.
- Sun, H.X.; Huang,F.; Ding, Z.J.; Zhang, C.J.; Zhang,L.; Zhang,H. Low-field Nuclear Magnetic Resonance Analysis of the Effects of Heating Temperature and Time on Braised Beef. Int. J.Food Sci. Technol. 2017, 52(5), 1193–1202. DOI: https://doi.org/10.1111/ijfs.13384.
- Martens,H.; Staburvik,E.; Martens,M. Texture and Colour Changes in Meat during Cooking Related to Thermal Denaturation of Muscle Proteins. J Texture Stud. 1982, 13(3), 291–309. DOI: https://doi.org/10.1111/j.1745-4603.1982.tb00885.x.
- Angel-Rendon, S.V.; Filomena-Ambrosio,A.; Hernandez-Carrion,M.; Llorca,E.; Hernando,I.; Quiles,A.; Sotelo-Diaz,I. Pork Meat Prepared by Different Cooking Methods. AMicrostructural, Sensorial and Physicochemical Approach. Meat Sci. 2020, 163, 108089. DOI: https://doi.org/10.1016/j.meatsci.2020.108089.
- Xue,S.; Yang,H.; Liu,R.; Qian,C.; Wang,M.; Zou,Y.; Xu,X.; Zhou,G. Applications of High Pressure to Pre-rigor Rabbit Muscles Affect the Functional Properties Associated with Heat-induced Gelation. Meat Sci. 2017, 129, 176–184. DOI: https://doi.org/10.1016/j.meatsci.2017.03.006.
- Bouton, P.E.; Harris, P.V. The Effects of Cooking Temperature and Time on Mechanical Properties of Meat. J.Food Sci. 1972, 37(1), 140. DOI: https://doi.org/10.1111/j.1365-2621.1972.tb03404.x.
- Bouton, P.E.; Harris, P.V. Changes in the Tenderness of Meat Cooked at 50-65 °C. J.Food Sci. 2010, 46(2), 475–478. DOI: https://doi.org/10.1111/j.1365-2621.1981.tb04889.x.