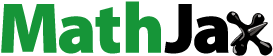
ABSTRACT
Native corn starch has a lack of characteristics such as low thermal stability, low resistance to shear force, and a high tendency to retrograde. To address this problem, it needs to be modified to improve its application in the food sector. Hydrothermal modification is one of the physical modifications by heating the starch above the gelatinization temperature with a limited water content. In this study, native corn starch was modified using various methods of hydrothermal modification which did not cause full gelatinization of starch and maintained starch granule integrity i.e., heat-moisture treatment (HMT), autoclave-heat treatment (AHT), microwave-heat treatment (MHT), and osmotic-pressure treatment (OPT). HMT, AHT, and MHT were modified by limited moisture content (30%) and then was heated using an oven, autoclave, and microwave, respectively, while OPT was heated in Na2SO42- solution using an autoclave. The results showed that the thermal modification did not alter the A-type crystallinity pattern of its native starch, but an amylose-lipid complex occurred resulting A + V-type crystallinity pattern. AHT starch has the highest V-type peak which indicated the highest amylose-lipid complex. The relative crystallinity increased after modification treatment except for OPT. The thermal modification increased thermal stability and increase the retrogradation ability of native corn starch. Parameters of thermal characteristics such as To, Tp, and Tc increased and Range of Tc – To decreased (except for HMT) after thermal modification. Solubility, water absorption capacity, gel strength, and syneresis of native corn starch tend to increase after modification. The best thermal modification of corn starch was OPT because it can produce the modified starch that not only had the highest thermal stability but also a lower retrogradation ability, the lowest swelling volume, higher water absorption capacity, and gel strength. Therefore, the hydrothermally modified corn starch could be applied to canned food, noodle, and bakery.
Introduction
The production of corn keeps increasing which makes it a very strategic commodity to be utilized. As the largest component of corn, the processing of corn into starch for industrial raw materials is very important in increasing the value of corn. Corn starch plays an important role in the food industry, such as a raw material for corn syrup production and thickening ingredient in the manufacture of various condiments. Starch in the food systems and food processing has to retain its desired functionality after undergoing a diverse range of conditions such as high-temperature processing, low pH processing, freezing-thawing operations, and prolonged refrigeration storage. Unfortunately, native corn starch could not provide such characteristics because it generally has low thermal stability, low resistance to shear force, and is easy to retrograde at low temperatures. [Citation1,Citation2] To improve starch application and promote its utilization, there is a need to modify its characteristics. Such modification can be achieved through several methods i.e., chemical, physical, and enzymatic.
Physical modification of starch was widely used because it was not using any chemicals, eco-friendly, do not create harmful by-products [Citation3] which is classified into thermal and non-thermal modifications. Hydrothermal treatment is a thermal modification where starch was heated above gelatinization temperature in limited moisture content. Some previous studies have reported that hydrothermal modification can improve the thermal stability of native starch.[Citation4–7] The hydrothermal treatment decreased starch molecule chains mobilities in amorphous regions which were caused by increased association among the starch polymer chain such as amylose-amylopectin, amylopectin-amylopectin, and also starch-lipid complexes, resulting in higher thermal stability of native starch.[Citation7]
On the other hand, Grgić, et al.[Citation8] have reported that non-thermal treatment also improves the starch characteristics such as increased thermal stability and swelling volume and reduced ability to retrograde. Non-thermal treatment can also retain the nutrient, color, and aroma of the product. Although non-thermal treatment has several advantages, however, it is still rarely applied in industry, needs specific equipment, and remains at a laboratory scale, i.e., ultrasound, cold plasma, pulsed electric field, etc. .[Citation9]
Heat-moisture treatment is a hydrothermal modification in which starch was heated above gelatinization temperature (80–120°C) and limited moisture content (10–35%) for 15 min – 16 h. [Citation10,Citation11] It was considered a green, safe, and low-cost technique to improve starch characteristics. The conventional heat-moisture treatment using a hot-air oven has some drawbacks i.e., required longer heating treatment and higher energy,[Citation12] heterogenous heat distribution and penetration in large-scale process, steam condensation during treatment resulting in a mixture of gelatinized starch and heat-moisture treatment starch.[Citation13]
In this present study, heat-moisture treatment was also conducted using other heating methods such as microwave and autoclave heating to overcome the issue of conventional heat-moisture treatment. Microwaves are non-ionizing electronic radiation capable to induce changes in material properties because of the presence of rapid alternations of the electronic field at higher frequency levels. Microwave is more efficient than the conventional heating process because it needs shorter heating time and produces a more homogeneous heat transfer in the whole volume of material. [Citation12] Whereas autoclave heating which was commonly used in commercial processes uses pressurized saturated steam to generate not only heat but also pressure in heating treatment.
In addition, this study also uses osmotic pressure treatment to modify the corn starch. Osmotic-heating treatment is a hydrothermal modification technique where starch was heated in saturated sodium sulfate solution to inhibit starch gelatinization. Sodium sulfate (Na2SO4) is a salt commonly used in this treatment, where it plays a role to increase the osmotic-pressure condition of the solution and restrict starch granules to gelatinize.[Citation14] This process is similar to heat-moisture treatment where the starch could not gelatinize due to the limited moisture content and high temperature. The inhibitory effect of Na2SO4 on starch gelatinization was caused by the limitation of the solvent mobility and a decrease of aw and inhibited the water to penetrate starch granules.[Citation13]
Several previous studies have reported that heat-moisture treatment could improve some characteristics of native starch. i.e, increased thermal stability of starch,[Citation4,Citation5] slowly digestibility starch,[Citation5] and water absorption capacity.[Citation11,Citation15] Some previous studies also reported the application of AHT on several starches i.e. proso millet,[Citation16] breadfruit,[Citation4] sweet potato.[Citation17] AHT increased water absorption index, water solubility index, setback viscosity,[Citation17] decreased breakdown,[Citation4,Citation17] and peak viscosity.[Citation16]
On the other hand, Marta, et al.[Citation4] have reported MHT reduced peak and breakdown viscosities of native breadfruit starch. MHT was also evidenced to increase water-holding capacity as well as to decrease peak, breakdown, and setback viscosities,[Citation16] and also reduce swelling volume.[Citation18] Some previous studies on OPT have reported that OPT increased the thermal stability of native breadfruit starch[Citation4] and potato starch.[Citation13] Although a lot of study on hydrothermal modification has been carried out, however, information on comparative studies between these hydrothermal methods on corn starch is still limited. Therefore, this study aims to determine the effect of different hydrothermal modifications on the physicochemical and pasting properties of corn starch and determine the best treatment to improve the characteristics of native corn starch, especially thermal stability and retrogradation ability.
Materials and methods
Materials
The raw material used in this research is Pioneer 21 Corn obtained from Kiara Payung, Sumedang, West Java, Indonesia, sodium sulfate, and sodium bisulfite that were analytical grade.
Corn starch extraction
Corn kernels were washed in water and soaked in 50°C water for 2 h to soften the corn kernel, which can facilitate the extraction process. The soaked kernels were then blended with 3 Liter water for 1 kg corn kernels and the slurry was filtered using the filter cloth. The filtrate was then allowed to precipitate for 24 h. The precipitated starch was then decanted and washed with water and allowed to precipitate again. The resulting starch was dried at 50°C for 24 h in a drying oven. Then, it was milled using a miller machine and sieved using a 100-mesh sieve.
Modification of corn starch
The heat-moisture treated corn starch was prepared according to the method reported by Trung, et al.[Citation19] with a slight modification. The first, native corn starch was adjusted to 30% moisture content. The resulting starch was then poured into an air-tight container and put into a refrigerator at 4°C for 24 h to equilibrate the water content. The starch was heated in an oven (Memmert UNB-300) at 100°C for 16 h and dried at 50°C for 24 h in a drying oven (Shel Lab FX-14-2). Then it was milled and sieved to particle-sized ≥ 100-mesh and packed in impermeable plastics for later analysis.
The autoclave-heat treated corn starch was prepared according to the previous method.[Citation4] The starch sample was adjusted to 30% moisture content. The moistened starch was then equilibrated in a refrigerator at 4°C for 24 h before heating at 120°C, 15 psi for 1 h in an autoclave (Raypa AE-28 Dry). AHT starch was removed from the Duran bottle after cooling to room temperature and dried at 50°C for 24 h in a drying oven (Shel Lab FX-14-2). Then it was milled and sieved to particle-sized ≥ 100-mesh packed in impermeable plastics for later analysis.
The microwave-heat treated corn starch was prepared according to the previous method.[Citation4] The corn starch sample was adjusted to 30% moisture content by adding the considered amount of distilled water to the starch. The moistened starch was then placed into a microwave-safe container and put into the refrigerator at 4°C for 24 h. The equilibrated starch was heated in a microwave at 1 W/g power for 20 min. The resulting starch was dried at 50°C for 24 h in a drying oven (Shel Lab FX-14-2). Then it was milled and sieved to particle-sized ≥ 100-mesh packed in impermeable plastics for later analysis.
The osmotic-pressure treated corn starch was prepared using the method of Pukkahuta, et al.[Citation13] Saturated sodium sulfate solution and autoclave was used to obtain osmotic-pressure condition in the solution. A saturated sodium sulfate solution was prepared by dissolving 100 g of Na2SO4 per 200 mL of distilled water. The solution (200 mL) was then transferred into a 500 mL Duran glass bottle followed by adding 100 g starch (dry basis). The suspension was heated at 120°C, 15 psi for 1 h in an autoclave to produce the osmotic pressure of 341 atm (345 bar) (assuming sodium sulfate dissociated completely).[Citation13] The starch was removed from the bottle, washed with 500-mL distilled water eight times to remove the residue of Na2SO4, and precipitated by centrifugation at 2050 × g after the bottle was allowed at room temperature. The obtained starch was dried at 50°C for 24 h in a drying oven (Shel Lab FX-14-2). Then it was milled and sieved using a 100-mesh sieve then packed in impermeable plastics for later analysis.
Chemical composition and amylose content determined
Moisture content was determined by referring to AOAC 925.04[Citation20] method which was then gravimetrically determined. Protein and fat content were determined using the Kjeldahl method, AOAC 981.10,[Citation20] and the Soxhlet extractor method, respectively. Whereas starch and amylose content was determined by the direct acid hydrolysis method[Citation21] and International Rice Research Institute (IRRI) method,[Citation22] respectively.
Granular morphology determined
Granular morphology was determined using scanning electron microscopy (SEM); a JEOL JSM-6360 LA at 15 kV. Starch samples were mounted, and the sample holder was sealed with silver paint and coated with gold/palladium at 8–10 mA for 10–15 min, under low pressure of fewer than 10 torrs. Representative digital images of starch granules were obtained at 10000x magnifications.
Starch crystallinity measurement
The crystallinity of starch samples was analyzed using a PANalytical X’Pert PRO series PW3040/x0 (x-ray diffraction) with a copper tube operating at 40 kV and 30 mA. This diffractometer produces a Cu-K α-radiation of 1.540 nm wavelength. The diffractogram of the starch samples was obtained by scanning the sample from 5° to 50° (2θ) with a 2.9 s scanning rate.
Fourier transform infrared spectroscopy (FTIR)-ATR
The starch samples were completely mixed with KBr (1:3 w/w) and pressed into pellets and then subjected to Attenuated Total Reflectance (ATR) Spectroscopy using an FT-IR spectrophotometer (Thermo Scientific Nicolet iS10, Massachusetts, USA). The wavelength range and spectral resolution used were 4000–500 cm−1 and 8 cm−1 by 32 scans, respectively. The absorbance ratio of 1047 cm−1/1022 cm−1 and 995 cm−1/1022 cm−1 were calculated to express the amount degree of order (DO) and degree of the double helix (DD) ordered crystalline to amorphous domains in starch samples.[Citation23]
Pasting properties
Pasting properties of starch samples were analyzed using Rapid Visco Analyzer (RVA) (Model 4D, Newport Scientific, Australia). About 3 g of starch sample (10% moisture basis) was added and mixed into 25 g of distilled water to form a starch suspension. The suspension was then poured into an RVA canister. The sample was maintained at 50°C for 1 min, heated at a rate of 12.0°C /min to 95°C, and then held at 95°C for 2.5 min. Afterward, it was cooled to 50°C for 2.5 min and then kept at 50°C for 2 min.
Thermal properties measurement
Thermal properties of the starch such as To (onset temperature), Tp (peak temperature), Tc (conclusion temperature), and the enthalpy of gelatinization (ΔH) were measured using DSC. A DSC-Q100, TA Instruments were used in this study. The starch was first turned into slurries by mixing one part of starch with three-part of water. The slurries were then sealed hermetically using a DuPont encapsulation press before weighing. The next step was to heat the sample at 5°C/min rate from 20 to 100°C.
Functional properties
Swelling volume & solubility: Swelling volume (SV) was determined by adding 12.5 mL of distilled water to a centrifuge tube that contains 0.35 g (dry basis) of corn starch.[Citation24] Samples were mixed using a vortex mixer for 20 seconds then heated in a water bath at 92.5°C for 30 min with constant stirring. Samples were cooled in ice water for 1 min before centrifugation at 2050 × g for 30 min. The volume of the supernatant obtained was measured and dried in a hot air oven. The swelling volume and solubility of the starch can be calculated using EquationEquations 1(1)
(1) and Equation2
(2)
(2) . Where TV is total volume, SV is supernatant volume, Ws is the weight of the sample, and Wds is the weight of dried supernatant.
Water Absorption Capacity (WAC): WAC of the starch samples was determined by mixing 1 g of the starch sample with 10 mL of water in a centrifuge tube. The sample was allowed to stand at room temperature for 1 h and centrifugated at 2050 × g for 30 min. The volume of the supernatant obtained was measured and the WAC was calculated by dividing the volume of water absorbed (g) by the weight of the sample (g).[Citation24]
Gel Strength: Gel strength was measured according to the method of Zhu, et al.[Citation25] Starch (11% w/v dry basis) was mixed with distilled water at 95°C for 30 min with constant stirring. The resulting paste was then poured into a pipe container (2.5 cm diameter and 3 cm high) at room temperature to form a gel and left for 1 h, covered with aluminum foils then left in the refrigerator at 4°C for 24 hours. The gel formed was then measured with a Texture Analyzer (TA-XT2).
Freeze-Thaw Stability (% Syneresis): The freeze-thaw stability was determined according to the method of Cahyana, et al.[Citation5] A starch solution (8% w/v) was made on a centrifuge tube and the pH was adjusted to 6. The starch solution was then heated and held in a water bath at 95°C for 30 min and cooled until 50°C. 20 g of the starch paste was then weighed accurately and transferred to pre-weighed 50 mL centrifugal tubes. The tubes were then capped tightly and stored at 4°C for 24 h before freezing at −20°C for 48 h. The frozen samples were then thawed at room temperature for 4 h and centrifuged at 2050 × g for 15 min. The exuded water (supernatant) was then weighed. The percentage of syneresis was calculated by dividing the weight of the supernatant by the weight of the starch paste.
Color characteristics measurement
A Spectrophotometer CM 5 (Konica Minolta Co., Osaka, Japan) with Spectra Magic software was used to measure the color characteristics of native and modified corn starches. The color measurement includes L* (lightness, 0 = black /100 = white), a* (+a* = redness/-a* = greenness), and b* (+b* = yellowness/-a* = blueness). Whiteness index was calculated using EquationEquation 3(3)
(3) .[Citation26]
Statistical analysis
Data are presented as the mean ± SD of triplicate experiments. All the data were further analyzed by ANOVA (analysis of variance) and Duncan’s multiple range test at a confidence level of 0.95 (P < 0.05) to determine if a significant difference between the two mean values. Pearson correlation coefficients were determined to identify relationship between variables. One-way ANOVA and Pearson correlation was evaluated by using IBM SPSS 21 Statistical Software Program.
Result and discussions
Chemical composition
The chemical composition of the native and modified starches is presented in . The protein, fat, ash, and starch content of the native and modified corn starch were not significantly different, which means the modification process might not have affected the quantities of these components, although their molecule structure might have changed. These results were in line with those observed by Deka and Sit[Citation12] for hydrothermal treated taro starches. The ash content in OPT starch was not significantly different from native starch which showed that there was no Na2SO4 residue in OPT starch. The starch content of native and all modified starches was not significantly different ranging from 89.43 to 90.44%, which indicated the amount of non-starch component was less than 10%. The lower of non-starch component content, the higher purity of starch.
Table 1. Chemical composition of native and various thermally modified corn starches
Amylose content of all modified starches (28.31–28.57 %db) increased significantly from the native starch (26.70 %db). The hydrothermal treatment in HMT might breakdown the amylopectin chain resulting in the formation of the amylose chain. In autoclave heating, starch granules were heated using high temperature and pressure which might have a higher heat intensity than HMT resulting in the highest amylose content compared to the other modified starches. Whereas in microwave heating, starch granule heated instantly and in very less time absorbed energy but the amylose content was not significantly different with HMT starch. This result was in agreement with other studies.[Citation12] Therefore, the amylose content of OPT starch was not significantly different from HMT. Although the heating process in OPT used a pressurized autoclave, the amylose produced was lower than AHT. It might be caused that the OPT process involved the presence of a sodium sulfate solution which could inhibit gelatinization by limiting the amount of water that can enter the starch granules and reducing the number of broken amylopectin chains.
Granule morphology
Corn starch granules tend to be polyhedral in shape and were smooth on the surface, which was in line with some previous reports.[Citation27–29] The thermal modification altered the surface but maintain the integrity of some corn starch granules (). All modified starches showed starch granule surface deformation which appeared to be less shaped, cracks, cavities, or coarse. Some of the modified starch granules clumped each other which indicated that modified starch could lead to a formation of a cohesive mass of starch granules.
Figure 1. Granule morphology of native and various thermally modified starches at 10.000x magnification (HMT = heat-moisture treatment, MHT = microwave-heat treatment, AHT = autoclave-heat treatment, OPT = osmotic-pressure treatment).
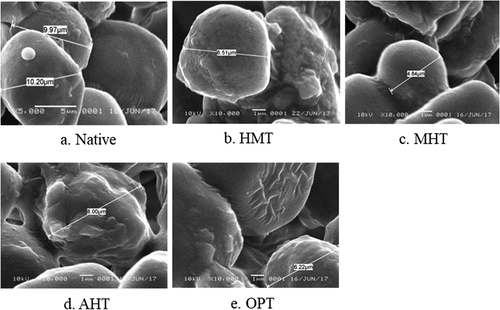
Granule morphological changes on HMT, MHT, and AHT could occur due to the limited moisture content and thermal force that was applied to the starch during the modification process. The absorption of water during heat treatment causes the starch molecules to have more mobility, causing them to expand and promoting morphological changes to the starch granules. The changes on starch granule surfaces and integrity could also occur due to the melting of the starch granule surface and the partial gelatinization of the starch granules, which are facilitated by the addition of water and heat energy to the starch system. Similar findings were reported for hydrothermally modified taro starch,[Citation12] finger millet,[Citation30] banana starch,[Citation11] and breadfruit starch.[Citation4]
The different modifications resulted in just slightly different effects on the surface of the starch granule. HMT, MHT, and AHT starches were heated above glass transition temperature and restricting moisture content, which caused partial gelatinization, while OPT starch was heated in saturated sodium sulfate solution that produce osmotic pressure in the system. The presence of sodium sulfate in the solution system can inhibit the gelatinization of the starch. The different methods of heating in starch modification (HMT, AHT, and MHT) did not give a greater effect on the starch granule surface, whereas OPT corn starch showed more layered strips. The presence of Na2SO4, heat, and pressure in OPT produced osmotic pressure in the solution system, which caused plasmolysis on the starch granule.[Citation13]
Starch crystallinity
The crystallinity of native and modified corn starches is presented in . Native corn starch has a single sharp peak at 15° 2θ, a double peak between 17° and 18° 2θ, and a single sharp peak at 22° 2θ. According to Patel, et al.[Citation31] this XRD pattern showed an A-type starch characteristic. A similar pattern was also shown on modified corn starches, whereas there was an additional small peak at 20° 2θ that indicated the formation of V-type starch due to the complex formation between amylose and lipid.[Citation32] Therefore, the thermal modification of corn starch can lead to the formation of A + V-type starches. The unaltered crystalline properties of A-type starch in HMT corn starch are in agreement with Hoover[Citation33] and Zhao, et al.[Citation34]
Figure 2. XRD diffractograms of native and various thermally modified starches (HMT = heat-moisture treatment, MHT = microwave-heat treatment, AHT = autoclave-heat treatment, OPT = osmotic-pressure treatment).
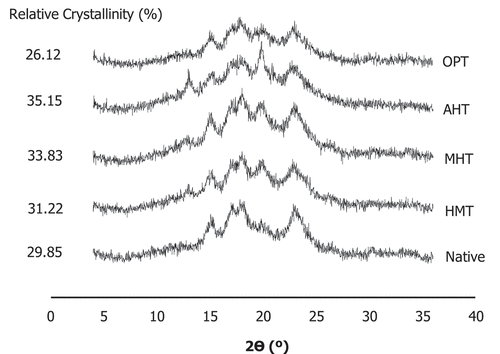
The XRD pattern of MHT starches showed that microwave treatment did not alter the basic XRD pattern of the native corn starch (A-type), but similar to the other modification treatments in this study, it produced a V-type crystallinity pattern resulting in A + V-type crystallinity pattern. Oyeyinka, et al.[Citation35] have reported that the alteration of XRD crystallinity pattern after MHT was occurred to the starch with B-type and did not occur to the starch with A-type crystalline pattern. The corn starch in this study has an A-type crystalline, which will not be altered by MHT which was in line with some previous studies.[Citation36–39] MHT also produced the V-type crystallinity pattern (peak at 20° 2θ) which indicated the presence of an amylose-lipid complex.
AHT and OPT starches also have a similar crystallinity pattern to the native starch formed A + V-type crystallinity pattern. The AHT starch has the highest peak at 20° 2θ which indicated that AHT starch produces more amylose-lipid complexes than other modified corn starches. The amylose-lipid complex formation could be attributed to the appearance of a peak at 20° 2θ. Hence, the stronger the peak at 20° 2θ, the more amylose-lipid complex were formed. [Citation31]
The relative crystallinity (RC) of all modified starches was higher than its native starch except for the OPT starch. An increasing RC value after heat-moisture treatment was in line with some previous studies.[Citation5,Citation40] Furthermore, Chen, et al.[Citation40] has reported that the increase of RC after HMT was most likely caused by the rearrangement of the starch molecules which lead to an increasing interaction between the starch molecules.
AHT starch has the highest RC among all modified starches, which might be due to the presence of high temperature and pressure in autoclave heating resulting in more order crystalline and amylose-lipid complex than other modification treatments. However, this result was not in line with the degree of molecular order which was obtained from FTIR measurement. Although the number of the double helix of modified starch was decreased, their RC was relatively higher than native except for OPT. A similar observation was reported by Qin, et al.[Citation23] for modified arrowhead starch, which also found that the higher RC did not show a higher degree of molecular order. The higher degree of RC was probably due to the peak produced by the amylose-lipid complex at 20° 2θ resulting in increased RC.
The increased RC on MHT starches was also reported in a study by Xia, et al.[Citation41] which shows an increasing RC value from 31.72% to 33.05% on microwave-heat treated potato starch. The increase of RC value after microwaving the starch can be caused by the generation of double helix chains which can lead to the formation of a more ordered crystalline array compared to the native one. On the other hand, RC of OPT starch has a different trend from other modification treatments, where RC of OPT starch was lower than native starch. The presence of Na2SO4 in OPT could increase the relative crystallinity of native corn starch which was in line with some previous studies.[Citation42,Citation43] Starch degradation was accelerated by salt addition which might lead to producing more simple polymer entities that then might undergo further fragmentation and reduce the relative crystallinity.[Citation43] The crystallinity of starch is affected by several factors i.e., amylose/amylopectin ratio, starch granule size, amylose-lipid complexes, length of amylopectin chains, double helices orientation within crystalline domains, the extent of interaction between double helices, and the lipid content of the starch.[Citation44,Citation45]
Molecular order degree (FTIR-ATR)
The FTIR spectra of native and modified corn starches are presented in , which provide information for short-range structure characteristics such as helical order, helicity, and chain conformation since the FTIR-ATR only penetrates as deep as 2 μm into the granule. [Citation23,Citation46] FTIR can also be used to identify the changes that occurred following the physical modification of starch on a structural level. Native and all modified corn starches showed similar FTIR spectra indicating there was no new functional group formed and suggesting all of these modification treatments belong to physical modification.
Figure 3. FTIR-spectra of native and various thermally modified corn starches (HMT = heat-moisture treatment, MHT = microwave-heat treatment, AHT = autoclave-heat treatment, OPT = osmotic-pressure treatment).
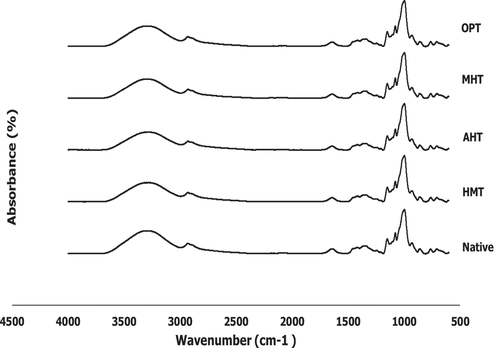
However, the absorbance strength difference of each treatment could be observed. The broadband around 3100–3700 cm−1 represents the – OH group vibrational stretches of intra- or intermolecular hydrogen bonds.[Citation23] All modified starch has a narrow absorption band between 3100 and 3700 cm−1 than native starch, which was similar to the result of Qin, et al.[Citation23] The vibration stretches of C – H bond were also observed on native and modified corn starches, which was indicated by the peak around 2936–2934 cm−1. The native corn starch shows higher absorption intensity compared to the modified starches. The sharp peak at around 1641 cm−1 was related to the water bending of starch granules. The broadband at 1641–1642 cm−1 was shifted to the lower value on the modified starch, which might be caused by a partially gelatinized starch sample.[Citation47] Meanwhile, the absorption intensity at 1200–800 cm−1 region with a strong peak at 995 cm−1 represented the C – C, C – O, C – H stretching vibration, and bending modes of COH. [Citation23] Furthermore, The absorption peak at around 930 cm−1 was also observed, which was attributed to the stretching of C – O bonds in C – O – C groups, which also represents an α 1→4 glycosidic linkages. [Citation48]
FTIR could also measure the degree of order and internal changes in the degree of the double helix of starch granules, which was calculated by the ratio of the absorbance band at 1047 cm−1 and 1022 cm−1 and the ratio of the absorbance band at 995 cm−1 and 1022 cm−1, respectively.[Citation23] The degree of order is the amount of ordered crystalline region to the starch amorphous region or the degree of starch short-range ordered structure.[Citation49] shows that 1047/1022 cm−1 ratio of the modified starch was significantly lower than native starch, which indicated that the modified starches might have a lesser degree of order. AHT starch has the lowest degree of order among modified starches, which might be due to AHT involved heat and pressure resulting in higher heat intensity and higher structural damage than other treatments. This was presumably due to partial gelatinization, which disrupted amylopectin’s ordered crystalline structure.[Citation50] Similar observation for hydrothermally modified arrowhead starch,[Citation24] heat-moisture treated banana starch, [Citation11] and microwave heating treated barley starch.[Citation51]
Table 2. The degree of order (DO) and degree of double helix (DD) native and various thermally modified corn starches
A similar trend has been observed in the degree of double (DD) helix data, where the modified starches tend to have a lower degree of double helix than the native starch, although DD of MHT and OPT starches are not significantly different from its native starch. The degree of double helix of AHT and HMT starch was significantly lower than its native starch. The decrease DD of the hydrothermal treatment might be due to disrupt the crystalline region and the double helix’s structure.
Pasting properties
The pasting properties of native and modified corn are shown in and . Thermal modification significantly increased the pasting temperature (PT) of starch. HMT and MHT starch were not significantly different but both significantly different from AHT and OPT starch. The increase of PT might be caused by the alteration in crystalline regions as the result of the reorientation of starch granules chains.[Citation52] Furthermore, Stute[Citation53] has reported that during the modification process, the molecular interaction between amylose in the crystalline region and amylopectin in the amorphous regions forms a strong structure with a hydrogen bond.
Figure 4. Viscoamylograph of native and various thermally modified corn starches (HMT = heat-moisture treatment, MHT = microwave-heat treatment, AHT = autoclave-heat treatment, OPT = osmotic-pressure treatment).
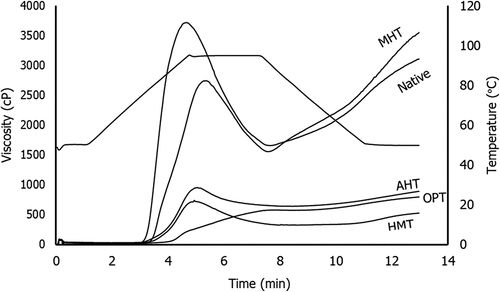
Table 3. Pasting properties of native and various thermally modified corn starches
OPT starch has the highest PT among the other treatments. It might be due to the presence of SO42-ions could facilitate the structure formation and protect or strengthen the hydrogen bonds in starch, causing an inhibition of starch-water molecules interaction due to their smaller diameter and more asymmetry structure compared to the structure-breaking ions.[Citation54] This increase in PT was in agreement with the increase of To, Tp, and Tc from the DSC analysis. The increase of gelatinization properties of OPT starch was due to the presence of a structure-forming ion, which is SO42-. A similar finding was reported for OPT potato starch.[Citation13] OPT has a lower setback than its native starch, which indicates a lower retrogradation ability to retrograde. OPT involved the presence of Na+ ions, which have relatively large asymmetric and electron particle structures resulting in a low tendency for retrogradation.[Citation54]
The PT of MHT starch was higher than the native starch which was in agreement with the previous work on MHT starch from waxy maize starch,[Citation37] breadfruit starch,[Citation4] and cassava starch.[Citation35] The MHT could affect the crystalline region of the native starch which increases the fraction of amylose in the starch granule.[Citation39] The increase in amylose content after MHT was due to the decomposition of amylopectin chains.[Citation55] The presence of water molecules in starch granules induced the breakage of the glycosidic bond at α-1,4 and α-1,6.[Citation37] The polar water molecules were vibrated and the linkages were broken caused by microwave irradiation. The microwave-treated potato starch has a greater amorphous region proportion thus increasing the amylose fraction in a starch granule.[Citation56] Increasing amylose content after microwave heating treatment was also reported by some previous studies.[Citation37,Citation39,Citation57]
Huang, et al.[Citation58] have reported that the increase in PT of AHT starch occurs due to the increase of intramolecular bonding strength. Furthermore, Maache-Rezzoug, et al.[Citation59] explained that the modification reorganized the amylose and amylopectin molecules to form new, more complex, stronger, and denser which leads to the stability of molecular interactions in the starch granules.
The PV of all modified corn starches was decreased. The PV of OPT starch was the lowest among the other treatments but it was not significantly different from the HMT and AHT starches, which were six- to sevenfold smaller than native starch. According to Luo, et al.,[Citation36] the decrease in PV was due to the association of the starch chains caused by the increase in the inter and intramolecular hydrogen bonds that can be attributed to the limited development of the granules before they finally broke and formed the paste. In MHT starch, the PV was higher than the other modified starches, which that showed the integrity of the granules did not completely change during microwave heating.[Citation60]
All modified starches showed a decrease in breakdown viscosity, which indicated that the modified starches have higher thermal stability. The decrease of breakdown viscosity in all modified starches was significantly different. It might be due to the rearrangement of starch granules which makes it more resistant to heat and shear.[Citation53] Furthermore, the decrease in breakdown viscosity might be attributed to the formation of lipid-amylose complexes during modification, which was in line with a previous study.[Citation27]
Physical modification altered the setback viscosity of corn starch. HMT, AHT, and OPT starch have a significantly lower setback viscosity compared to native corn starch, whereas MHT starch was significantly higher. The decrease in setback viscosity of HMT starch might be due to an increase in bond strength in the amorphous region.[Citation61] The amylose molecules in native corn starch were dispersed more randomly than the modified corn starch so it can be oriented to form aggregates and gel formations.[Citation62]
Based on pasting properties, the best thermal modification is OPT because it has the lowest breakdown viscosity and lower setback viscosity which indicated the best thermal stability and the lower tendency to retrograde, which was relevant to the aim of this study. This study aimed to improve the lack of native corn starch such as lower thermal stability and higher retrogradation ability.
Thermal properties
The thermal properties parameters that are onset temperatures (To), peak temperatures (Tp), conclusion temperatures (Tc), and gelatinization enthalpy (ΔH) of native and modified corn starch are shown in . All modified starches lead to an increasing value of To, Tp, and Tc. The onset temperature (To) was defined as the melting temperature of the weakest crystalline in starch granules, while the conclusion temperature (Tc) was defined as the melting temperature of high-perfection crystalline.[Citation63] The increase in To, Tp, Tc, and Tc-To values on HMT starch might be due to the increasing interactions between amylose-amylose, amylose-amylopectin, and amylose-lipid which could lead to the more perfect crystalline formation and the higher the temperature was needed to gelatinize the starch.[Citation64] A similar finding has been reported by Chen, et al.[Citation32] and Liao, et al.[Citation65] for heat-moisture treated normal maize starch and sweet potato starch, respectively.
Table 4. Thermal properties of native and various thermally modified corn starches
The highest To, Tp, and Tc value of OPT starch was affected by the heat treatment of native corn starch in saturated Na2SO4 solution. Pukkahuta, et al.[Citation13] explained that the SO42- ions decrease the fraction of free water and the repulsion between the SO42- ions which have negatively charged with electronegative – OH groups of starch might inhibit starch gelatinization. The SO42- ions could protect or strengthen the hydrogen bonds in starch, causing a reduced or inhibition of starch-water molecules interaction due to their smaller diameter and more asymmetry structure compared to the structure-breaking ions. This phenomenon could lead to an increase in the gelatinization temperature of starch.[Citation54] This observation was in agreement with another study for OPT potato starch.[Citation13]
AHT has a similar process to HMT but with a different heating method where starch with limited moisture content was heated using an autoclave. This treatment involved not only heat but also pressure. During heating, the temperature was gradually increased at constant pressure. The increase of some parameters on the thermal properties (To, Tp, and Tc) might be due to the interaction between amylose-amylose, amylose-amylopectin, and amylose-lipid increasing the crystalline area, so that required higher temperature for gelatinization.
The increase in all parameters of thermal properties from microwave-treated starch is in agreement with Uthumporn, et al.[Citation66] The increase in To could indicate that microwave treatment needs a higher temperature to destroy the weaker crystallites in starch granules compared to the native.[Citation38] The formation of crystallites that have greater stability and the formation of a helical structure during microwave treatment, might be caused by the rearrangement of detached starch chains and the improved interaction between them, indicated by an increase in conclusion temperature (Tc). The double-helical order of the starch which was corresponded with the content and stability of the double helices was associated with ΔH. The decreasing ΔH value on HMT, MHT, and OPT starch could indicate that those physical modifications of corn starch break double helices, thus decreasing the stability of the crystalline region, which was consistent with degree of order and degree of double helix by FTIR. Whereas this data was inconsistent with AHT starch.
The HMT starch has the highest Tc-To value among all the starch samples, which can assume the HMT starch has a higher heterogeneity of crystal in the starch granule. It might be caused by inhomogeneous heat transfer during HMT. Pukkahuta, et al.[Citation13] also reported similar findings on heat-moisture treated potato starch. Conversely, the MHT, AH, and OPT starches have a lower Tc-To value than native starch, which indicates the heating treatment using a microwave and autoclave provides a more homogenous heat transfer than the conventional hot-air oven.
ΔH value (enthalpy) represents the energy required to break the double helix structure during starch gelatinization. All modified starches except AHT starch showed a lower enthalpy than native starch, which indicated a reduction of double helix content in the crystalline and non-crystalline area. This result was consistent with the degree of order data from FTIR for HMT, MHT, and OPT starches. AHT starch showed the reverse trend, AHT starch has higher enthalpy compared to native starch and the highest enthalpy compared to other modified starches. It might be due to the enthalpy not only influenced by the degree of order or degree of double helix but also other factors, such as relative crystallinity. AHT starch has the highest RC which was consistent with the highest enthalpy data. This observation was in agreement with a previous study on sago starch.[Citation67] Thermal properties were influenced by several factors such as degree of order, degree of double helix, starch crystallinity, the presence of ions, and amylose-lipid complex.
Functional properties
The functional properties that were analyzed in this research were swelling volume (SV), solubility (SOL), water absorption capacity (WAC), gel strength, and freeze-thaw stability (). The modification restricted granule swelling compared to the native one. The SV of all modified starches was not significantly different among them except for MHT starch. The decrease of SV in HMT starch was due to the rearrangement of the starch molecule, increase in intramolecular force, amylose-amylopectin interaction, and changes in the crystalline arrangement.[Citation68] This reduction of SV in HMT-treated starch can be also caused by the disruption of the helices in the crystalline areas which could affect the starch granular stability. These data were also in line with the degree of the double helix (DD) which was obtained from FTIR-ATR measurement (). The data showed that all modification treatments decrease the degree of the double helix of native corn starch, which was associated with decreased swelling volume. Furthermore, the decreasing SV after HMT was also in line with the ΔH value which decreased after HMT. The lower the ΔH value, the lower the stability and the content of the double helices in the starch, thus could lower the SV of the starch. The decrease in SV of HMT-treated starch was in agreement with Cordeiro, et al.,[Citation69] which has reported that the swelling power of banana starch was decreased after HMT due to the broken double helices which lead to the reduction of the granular stability.
Table 5. Functional Properties of native and various thermally modified corn starches
The presence of pressure in AHT modification caused an extension to the matrix and gelatinization of the granules, and during the cooling process, the partially dissolved fragments will be re-associated to a rigid film formation on the granular surface. This prevented water penetration and starch swelling.[Citation70] The decrease of SV in OPT starch is because of the SO42- ion role in protecting the starch structure and strengthening the hydrogen bond between the starch molecules.[Citation54] Ion SO42- have low polarization due to its smaller and more symmetric structure, which could inhibit water to absorb into starch granule so decreasing swelling volume. Furthermore, the formation of structure-making ions (salting-out ions) could decrease swelling volume.[Citation54]
The decrease in starch swelling after microwave heating is thought to be due to a reorganization of crystalline regions within the starch granules.[Citation39] Xu, et al.[Citation38] studied the effect of microwave treatment on the double helices contained in starch using 13C CP/MAS NMR Spectroscopy and showed that the number of double helices in the microwave-treated starch is lower than in the native starch. The decreasing number of double helices could lead to more unstable granular, hence decreasing the swelling volume of the starch.
The solubility of HMT, AHT, and OPT starches have significantly different compared to the native corn starch, which was higher. Whereas MHT did not give any significant differences in native corn starch. The highest solubility on AHT starch might be due to high temperature and pressure during AHT caused the degradation of the amylopectin chain.[Citation71] The decrease in the degree of order after modification might be increased the surface of the amorphous area, which increased the solubility of starch,[Citation27] which was consistent with degree of order data from FTIR.
The increase in OPT starch was in line with the most recent study by Gayary and Mahanta.[Citation72] In MHT starch, the heating involved radiation, which caused some degree of intragranular molecular rearrangement that led to lesser accessibility to the amorphous areas. Thus, the increase in the compactness of starch granules.[Citation73] resulting in a lower solubility than other modified starches, which was not significantly different from native starch.
The physical modification increased the WAC of corn starch. The modified corn starches were significantly different, except for the AHT and OPT starch. The increase in WAC was caused by the starch’s tendency to have rising hydrophilic properties [Citation74] which might be due to the disruption of the hydrogen bond between the amorphous and crystalline area resulting in a slight extension of the amorphous area.[Citation75,Citation76] In addition, the increase in WAC in modified starch was also caused by changes in the structure of the starch granule surface, which are more porous, making it easier to absorb water.
The modification treatment increased the gel strength of corn starch. All modified starches have higher gel strength compared to the native starch. The physical modification increased the gel formation.[Citation74] HMT, MHT, and AHT starches were modified using hydrothermal treatment but with different heating methods. The hydrothermal treatment could increase the gel strength by the formation of cross-linking between starch chains which induced more junction zone formation in the continuous phase of starch gel.[Citation75,Citation77] The gel strength of AHT, HMT, and AHT starches were not significantly different, which indicated the heating process using a hot air oven, autoclave and microwave did not affect the gel strength of modified starches. A similar trend to other modification treatments, OPT starch has higher gel strength which was not significantly different from other modified starches. OPT starch has a similar process to other modified starch where starch was heated using an autoclave in Na2SO4 solution to restrict the water intake to the granule resulting in a similar effect on gel strength.
The gel strength of MHT starch was not significantly different from other thermal modified starches, which was higher than native starch. This was different from the MHT starch which was different in the heating way. The high energy provided to the microwave-heat treated starch may lead to a different mechanism on how microwave-heating treatment yields a starch with higher gel strength compared to native starch. Even before the system hits gelatinization temperature, heating with a microwave causes the water present in the crystalline regions of the starch granules to vibrate, disrupting the lamellar structure of the amylopectin crystals and probably fragmenting the granule at higher temperatures while in the conduction heating, the gradually increased temperature could provide sufficient time which is needed to undergo all the steps in starch gelatinization. Choi and Kerr[Citation78] have reported that the gel strength was affected by the complex interplay of the dispersed and continuous phases of starch, and the crystallization of leaching amylose and amylopectin.
Freeze-thaw stability was calculated as the percentage of starch syneresis. Higher syneresis shows low stability and low resistance of starch at low-temperature storage. All modifications treatment significantly increased the syneresis of native corn starch. The syneresis of HMT, AHT, and OPT starch was not significantly different. The increase of syneresis in HMT, AHT, and OPT starches during thermal modification may be due to the randomly generated interaction which reduced the water-holding capacity of starch gel.[Citation79] The syneresis of MHT starch was significantly higher compared to the other modified starches. It might be due to the low amylose leaching, which caused more amount of amylose to be contained in the starch gel. The amylose content of all modified starches ranged from 26 up to 29%db (). Amylose leaching was represented by solubility value. MHT starch has the lowest solubility compared to other modified starches, indicating low amylose leaching. The syneresis measurement involved heating treatment to gelatinize the starch, which caused amylose to leach out from the starch granule. The amylose interaction was occurred in the starch granule, resulting in a greater volume of water coming out from the gel during thawing.
Color characteristics
Color characteristics of native and modified starches are presented in . All color parameters (L*, a*, b* values, and WI) were affected by modification treatment. All modified starches have lower lightness (L*) and whiteness index (WI) than native starch. The color change in modified starch was caused by the Maillard reaction during the modification proses, where the reducing sugars from heated starch react with the amino acids in the protein,[Citation12] producing dark color. A similar finding was reported for bambara groundnut starch,[Citation39] buckwheat starch,[Citation75] and taro starch.[Citation12] The lowest lightness and whiteness index of AHT starch might be caused by a combination of heat and pressure during the modification process, which gave a more severe effect than other heating methods.
Table 6. Color characteristic of native and various thermally modified corn starches
The a* and b* value of native and modified starches ranged from −0.40 to −0.48 and from 4.46 to 4.92, respectively, which indicated the slight greenish and yellowness in color. HMT starch has the lowest a* and b* value than native and other modified starches, which indicated less greenish and yellowness than other starch samples. Statistically, a* and b* value of native starch was significantly different from modified starches although the values are slightly different, visually indistinguishable.
Pearson correlation
The amylose content of the starch was negatively correlated with the degree of starch crystalline order (r = −0.726, p < 0.05) and swelling volume of the starch (r = −0.672, p < 0.05) but it was positively correlated with both syneresis value (r = 0.651), p < 0.05) and gel strength value (r = 0.674, p < 0.05) (). The positive correlation of amylose content and syneresis percentage was in agreement with Srichuwong, et al.[Citation80] who stated that the gels made from starches with high amylose content exhibit more syneresis behavior when compared to the gel with lower amylose content. This would be due to the long linear chain and high mobility of amylose molecules, leading to the greater syneresis. The negative correlation between amylose content and swelling volume of starch was in line with another study.[Citation81]
Table 7. Pearson correlation between some characteristic parameter of corn starch
As for the degree of crystalline order of the starch (DO), it was positively correlated with both breakdown viscosity (r = 0.666, p < 0.05) and swelling volume of the starch (r = 0.789, p < 0.01) but it has a negative correlation with water absorption capacity (r = −0.720, p < 0.05), syneresis value (r = −0.977, p < 0.01) and gel strength (r = −0.933, p < 0.01). The degree of double helices (DD) has positive correlation with all of the starch pasting properties except with pasting temperature. DD has a very strong, positive correlation with peak viscosity (r = 0.841, p < 0.01), holding viscosity (r = 0.848, p < 0.01), breakdown viscosity (r = 0.749, p < 0.01), final viscosity (r = 0.817, p < 0.01), and setback viscosity (r = 0.768, p < 0.01). On the other hand, the negative correlation was seen between DD and solubility of starch (r = −0.720, p < 0.01), and gel strength (r = −0.717, p < 0.05).
Peak viscosity of the starch was negatively correlated with solubility (r = −0.918, p < 0.01), water absorption capacity (r = −0.780, p < 0.01), and gel strength (r = −0.732, p < 0.01). As for the breakdown viscosity of the starch, it was negatively correlated with solubility (r = −0.878, p < 0.01), water absorption capacity (r = −0.886, p < 0.01), and gel strength (r = −0.813, p < 0.01). Swelling volume has a negative correlation with solubility (r = −0.697, p < 0.05), water absorption capacity (r = −0.946, p < 0.01), syneresis (r = −0.714, p < 0.05), and gel strength (r = −0.868, p < 0.01).
Conclusion
All modification methods did not alter the chemical compositions of native corn starch, except for amylose content. Amylose content increased after the modification. Starch morphology of modified starch have some deformation on the starch granule surface; become rougher, ruptured, and stuck each other but they still maintain the granule integrity. Native corn starch has an A-type crystallinity pattern and after thermal modification, the crystallinity pattern did not change but formed a V-type, which indicates the presence of amylose-lipid complexes, resulting A + V type crystallinity pattern. The relative crystallinity of native corn starch increased after modification except for OPT. Generally, all modification treatments improve the pasting properties of native corn starch, and the best thermal treatment to improve thermal stability and retrogradation ability is OPT. Some functional properties also improve after modification such as lower swelling volume, higher water absorption capacity, and gel strength. All Modification of corn starch increases the onset temperature, peak temperature, and conclusion temperature but it decreases the enthalpy of gelatinization of the corn starch. The recommended modification treatment to improve the characteristic of native corn starch is osmotic-pressure treatment due to the homogeneous heating transfer and produce modified starch with better characteristics such as higher thermal stability, gel strength, water absorption capacity, lower retrogradation ability, and swelling volume. OPT starch could be applied to canned food, noodle, and bakery.
Disclosure statement
No potential conflict of interest was reported by the author(s).
Additional information
Funding
References
- Singh, J.; Kaur, L.; McCarthy, O. J. Factors Influencing the Physico-Chemical, Morphological, Thermal and Rheological Properties of Some Chemically Modified Starches for Food Applications—a Review. Food Hydrocoll. 2007, 21(1), 1–22. DOI: 10.1016/j.foodhyd.2006.02.006.
- Niu, H.; Han, Q.; Cao, C.; Liu, Q.; Kong, B. Short-Term Retrogradation Behaviour of Corn Starch Is Inhibited by the Addition of Porcine Plasma Protein Hydrolysates. Int. J. Biol. Macromol. 2018, 115, 393–400. DOI: 10.1016/j.ijbiomac.2018.04.047.
- Thirumdas, R.; Kadam, D.; Annapure, U. S. Cold Plasma: An Alternative Technology for the Starch Modification. Food Biophys. 2017, 12(1), 129–139. DOI: 10.1007/s11483-017-9468-5.
- Marta, H.; Cahyana, Y.; Arifin, H. R.; Khairani, L. Comparing the Effect of Four Different Thermal Modifications on Physicochemical and Pasting Properties of Breadfruit (Artocarpus Altilis) Starch. Int. Food Res J. 2019, 26(1), 269–276.
- Cahyana, Y.; Wijaya, E.; Halimah, T. S.; Marta, H.; Suryadi, E.; Kurniati, D. The Effect of Different Thermal Modifications on Slowly Digestible Starch and Physicochemical Properties of Green Banana Flour (Musa Acuminata Colla). Food Chem. 2019, 274, 274–280. DOI: 10.1016/j.foodchem.2018.09.004.
- Xing, J.-J.; Liu, Y.; Li, D.; Wang, L.-J.; Adhikari, B. Heat-Moisture Treatment and Acid Hydrolysis of Corn Starch in Different Sequences. LWT - Food Sci. Technol. 2017, 79, 11–20. DOI: 10.1016/j.lwt.2016.12.055.
- BeMiller, J. N.; Huber, K. C. Physical Modification of Food Starch Functionalities. Annu. Rev. Food Sci. Technol. 2015, 6, 19–69. DOI: 10.1146/annurev-food-022814-015552.
- Grgić, I.; Ačkar, Đ.; Barišić, V.; Vlainić, M.; Knežević, N.; Medverec Knežević, Z. Nonthermal Methods for Starch Modification—a Review. J. Food Process. Preserv. 2019, 43(12), e14242. DOI: 10.1111/jfpp.14242.
- Jadhav, H. B.; Annapure, U. S.; Deshmukh, R. R. Non-Thermal Technologies for Food Processing. Front. Nutrit. 2021, 8, 657090–657090. DOI: 10.3389/fnut.2021.657090.
- Gunaratne, A.; Hoover, R. Effect of Heat–Moisture Treatment on the Structure and Physicochemical Properties of Tuber and Root Starches. Carbohydr. Polym. 2002, 49(4), 425–437. DOI: 10.1016/S0144-8617(01)00354-X.
- Marta, H.; Cahyana, Y.; Djali, M. Densely Packed-Matrices of Heat Moisture Treated-Starch Determine the Digestion Rate Constant as Revealed by Logarithm of Slope Plots. J. Food Sci. Technol. 2021, 58(6), 2237–2245. DOI: 10.1007/s13197-020-04734-6.
- Deka, D.; Sit, N. Dual Modification of Taro Starch by Microwave and Other Heat Moisture Treatments. Int. J. Biol. Macromol. 2016, 92(Supplement C), 416–422. DOI: 10.1016/j.ijbiomac.2016.07.040.
- Pukkahuta, C.; Shobsngob, S.; Varavinit, S. Effect of Osmotic Pressure on Starch: New Method of Physical Modification of Starch. Starch - Stärke. 2007, 59(2), 78–90. DOI: 10.1002/star.200600509.
- Gayary, M. A.; Mahanta, C. L. Optimization of Process Parameters of Osmotic Pressure Treatment and Heat Moisture Treatment for Rice Starch Using Response Surface Methodology. J. Food Meas. Charact. 2020, 14(5), 2862–2877. DOI: 10.1007/s11694-020-00531-z.
- Olayinka, O. O.; Adebowale, K. O.; Olu-Owolabi, B. I. Effect of Heat-Moisture Treatment on Physicochemical Properties of White Sorghum Starch. Food Hydrocoll. 2008, 22(2), 225–230. DOI: 10.1016/j.foodhyd.2006.11.004.
- Zheng, M. Z.; Xiao, Y.; Yang, S.; Liu, H. M.; Liu, M. H.; Yaqoob, S.; Xu, X. Y., and Liu, J. S. 2020 Effects of Heat–Moisture, Autoclaving, and Microwave Treatments on Physicochemical Properties of Proso Millet Starch. Food Sci. Nutr. 8 2 , 735–743. 10.1002/fsn3.1295.
- Silva, G.; Bento, J.; Bataus, L.; Soares Júnior, M.; Caliari, M.; Neto, M. A. D.; Ferreira, K.; Oliveira, A.; Andrade, J. White and Orange Fleshed-Sweet Potato Starches Modified by Autoclave. Res. Soc. Dev. 2021, 10, e14210111620. DOI: 10.33448/rsd-v10i1.11620.
- Lewandowicz, G.; Jankowski, T.; Fornal, J. Effect of Microwave Radiation on Physico-Chemical Properties and Structure of Cereal Starches. Carbohydr. Polym. 2000, 42, 193–199. DOI: 10.1016/S0144-8617(99)00155-1.
- Trung, P. T. B.; Ngoc, L. B. B.; Hoa, P. N.; Tien, N. N. T.; Hung, P. V. Impact of Heat-Moisture and Annealing Treatments on Physicochemical Properties and Digestibility of Starches from Different Colored Sweet Potato Varieties. Int. J. Biol. Macromol. 2017, 105(Part 1), 1071–1078. DOI: 10.1016/j.ijbiomac.2017.07.131.
- AOAC. Official Method of Analysis; The Association of Official Analytical Chemistry: Washington DC, 1995.
- AOAC. Official Method of Analysis; The Association of Official Analytical Chemistry: Washington DC, 1970.
- Graham, R. A Proposal for Irri to Establish A Grain Quality and Nutrition Research Center. IRRI Discussion Paper Series No.44 2002, 15 p.
- Qin, W.; Wen, C.; Zhang, J.; Dzah, C. S.; Zhang, H.; He, Y.; Duan, Y. Structural Characterization and Physicochemical Properties of Arrowhead Resistant Starch Prepared by Different Methods. Int. J. Biol. Macromol. 2020, 157, 96–105. DOI: 10.1016/j.ijbiomac.2020.04.096.
- Marta, H.; Cahyana, Y.; Djali, M.; Arcot, J.; Tensiska, T. A Comparative Study on the Physicochemical and Pasting Properties of Starch and Flour from Different Banana (Musa Spp.) Cultivars Grown in Indonesia. Int. J. Food Prop. 2019, 22(1), 1562–1575. DOI: 10.1080/10942912.2019.1657447.
- Zhu, F.; Cai, Y.-Z.; Sun, M.; Corke, H. Effect of Phytochemical Extracts on the Pasting, Thermal, and Gelling Properties of Wheat Starch. Food Chem. 2009, 112(4), 919–923. DOI: 10.1016/j.foodchem.2008.06.079.
- Yang, Z.; Wang, W.; Wang, H.; Ye, Q. Effects of a Highly Resistant Rice Starch and Pre-Incubation Temperatures on the Physicochemical Properties of Surimi Gel from Grass Carp (Ctenopharyn Odon Idellus). Food Chem. 2014, 145, 212–219. DOI: 10.1016/j.foodchem.2013.08.040.
- Pukkahuta, C.; Suwannawat, B.; Shobsngob, S.; Varavinit, S. Comparative Study of Pasting and Thermal Transition Characteristics of Osmotic Pressure and Heat–Moisture Treated Corn Starch. Carbohydr. Polym. 2008, 72(3), 527–536. DOI: 10.1016/j.carbpol.2007.09.024.
- Liu, H.; Liang, R.; Antoniou, J.; Liu, F.; Shoemaker, C. F.; Li, Y.; Zhong, F. The Effect of High Moisture Heat-Acid Treatment on the Structure and Digestion Property of Normal Maize Starch. Food Chem. 2014, 159(Supplement C), 222–229. DOI: 10.1016/j.foodchem.2014.02.162.
- Almeida, R. L. J.; Santos, N. C.; Feitoza, J. V. F.; da Silva, G. M.; Muniz, C. E. D. S.; Eduardo, R. D. S.; Ribeiro, V. H. D. A.; Silva, V. M. D. A.; Mota, M. M. D. A. Effect of Heat-Moisture Treatment on the Thermal, Structural and Morphological Properties of Quinoa Starch. Carbohydr. Polym. Technol. Appl. 2022, 3, 100192. DOI: 10.1016/j.carpta.2022.100192.
- Adebowale, K. O.; Afolabi, T. A.; Olu-Owolabi, B. I. Hydrothermal Treatments of Finger Millet (Eleusine Coracana) Starch. Food Hydrocoll. 2005, 19(6), 974–983. DOI: 10.1016/j.foodhyd.2004.12.007.
- Patel, H.; Day, R.; Butterworth, P. J.; Ellis, P. R. A Mechanistic Approach to Studies of the Possible Digestion of Retrograded Starch by Α-amylase Revealed Using A Log of Slope (Los) Plot. Carbohydr. Polym. 2014, 113(Supplement C), 182–188. DOI: 10.1016/j.carbpol.2014.06.089.
- Chen, Y.; Yang, Q.; Xu, X.; Qi, L.; Dong, Z.; Luo, Z.; Lu, X.; Peng, X. Structural Changes of Waxy and Normal Maize Starches Modified by Heat Moisture Treatment and Their Relationship with Starch Digestibility. Carbohydr. Polym. 2017, 177(Supplement C), 232–240. DOI: 10.1016/j.carbpol.2017.08.121.
- Hoover, R. The Impact of Heat-Moisture Treatment on Molecular Structures and Properties of Starches Isolated from Different Botanical Sources. Crit. Rev. Food Sci. Nutr. 2010, 50(9), 835–847. DOI: 10.1080/10408390903001735.
- Zhao, K.; Zhang, B.; Su, C.; Gong, B.; Zheng, J.; Jiang, H.; Zhang, G.; Li, W. Repeated Heat-Moisture Treatment: A More Effectiveway for Structural and Physicochemical Modification of Mung Bean Starch Compared with Continuous Way. Food Bioproc. Tech. 2020, 13(3), 452–461. DOI: 10.1007/s11947-020-02405-0.
- Oyeyinka, S. A.; Akintayo, O. A.; Adebo, O. A.; Kayitesi, E.; Njobeh, P. B. A Review on the Physicochemical Properties of Starches Modified by Microwave Alone and in Combination with Other Methods. Int. J. Biol. Macromol. 2021, 176, 87–95. DOI: 10.1016/j.ijbiomac.2021.02.066.
- Luo, Z.; He, X.; Fu, X.; Luo, F.; Gao, Q. Effect of Microwave Radiation on the Physicochemical Properties of Normal Maize, Waxy Maize and Amylomaize V Starches. Starch - Stärke. 2006, 58(9), 468–474. DOI: 10.1002/star.200600498.
- Yang, Q.; Qi, L.; Luo, Z.; Kong, X.; Xiao, Z.; Wang, P.; Peng, X. Effect of Microwave Irradiation on Internal Molecular Structure and Physical Properties of Waxy Maize Starch. Food Hydrocoll. 2017, 69, 473–482. DOI: 10.1016/j.foodhyd.2017.03.011.
- Xu, X.; Chen, Y.; Luo, Z.; Lu, X. Different Variations in Structures of A- and B-Type Starches Subjected to Microwave Treatment and Their Relationships with Digestibility. LWT. 2019, 99, 179–187. DOI: 10.1016/j.lwt.2018.09.072.
- Oyeyinka, S. A.; Umaru, E.; Olatunde, S. J.; Joseph, J. K. Effect of Short Microwave Heating Time on Physicochemical and Functional Properties of Bambara Groundnut Starch. Food Biosci. 2019, 28, 36–41. DOI: 10.1016/j.fbio.2019.01.005.
- Chen, X.; He, X.; Fu, X.; Huang, Q. In Vitro Digestion and Physicochemical Properties of Wheat Starch/Flour Modified by Heat-Moisture Treatment. J. Cereal Sci. 2015, 63, 109–115. DOI: 10.1016/j.jcs.2015.03.003.
- Xia, T.; Gou, M.; Zhang, G.; Li, W.; Jiang, H. Physical and Structural Properties of Potato Starch Modified by Dielectric Treatment with Different Moisture Content. Int. J. Biol. Macromol. 2018, 118, 1455–1462. DOI: 10.1016/j.ijbiomac.2018.06.149.
- Moreau, L.; Bindzus, W., and Hill, S. 2011. Influence of Salts on Starch Degradation: Part Ii – Salt Classification and Caramelisation. Starch - Starke. 63 11 , 676–682. DOI: 10.1002/star.201100032.
- Moreau, L.; Bindzus, W.; Hill, S. Influence of Salts on Starch Degradation: Part I – Sodium Chloride. Starch - Stärke. 2011, 63(11), 669–675. DOI: 10.1002/star.201100031.
- Sun, Q.; Gong, M.; Li, Y.; Xiong, L. Effect of Retrogradation Time on Preparation and Characterization of Proso Millet Starch Nanoparticles. Carbohydr. Polym. 2014, 111, 133–138. DOI: 10.1016/j.carbpol.2014.03.094.
- Cornejo, Y.; Martínez-Cruz, O.; Toro-Sánchez, C.; Wong-Corral, F.; Borboa-Flores, J.; Cinco-Moroyoqui, F. The Structural Characteristics of Starches and Their Functional Properties. CyTA J. Food. 2018, 16, 1003–1017. DOI: 10.1080/19476337.2018.1518343.
- Ambigaipalan, P.; Hoover, R.; Donner, E.; Liu, Q. Starch Chain Interactions within the Amorphous and Crystalline Domains of Pulse Starches during Heat-Moisture Treatment at Different Temperatures and Their Impact on Physicochemical Properties. Food Chem. 2014, 143, 175–184. DOI: 10.1016/j.foodchem.2013.07.112.
- Rashid, I.; Omari, M. H. A.; Leharne, S. A.; Chowdhry, B. Z.; Badwan, A. Starch Gelatinization Using Sodium Silicate: Ftir, Dsc, Xrpd, and Nmr Studies. Starch - Stärke. 2012, 64(9), 713–728. DOI: 10.1002/star.201100190.
- Ratnaningsih, N.; Suparmo,; Harmayani, E.; Marsono, Y. Physicochemical Properties, in Vitro Starch Digestibility, and Estimated Glycemic Index of Resistant Starch from Cowpea (Vigna Unguiculata) Starch by Autoclaving-Cooling Cycles. Int. J. Biol. Macromol. 2020, 142, 191–200. DOI: 10.1016/j.ijbiomac.2019.09.092.
- Warren, F.; Gidley, M.; Flanagan, B. Infrared Spectroscopy as a Tool to Characterise Starch Ordered Structure- a Joint Ftir-Atr, Nmr, Xrd and Dsc Study. Carbohydr. Polym. 2015, 139. DOI: 10.1016/j.carbpol.2015.11.066.
- Zhou, D.; Ma, Z.; Yin, X.; Hu, X.; Boye, J. I. Structural Characteristics and Physicochemical Properties of Field Pea Starch Modified by Physical, Enzymatic, and Acid Treatments. Food Hydrocoll. 2019, 93, 386–394. DOI: 10.1016/j.foodhyd.2019.02.048.
- Ma, M.; Zhang, Y.; Chen, X.; Li, H.; Sui, Z.; Corke, H. Microwave Irradiation Differentially Affect the Physicochemical Properties of Waxy and Non-Waxy Hull-Less Barley Starch. J. Cereal Sci. 2020, 95, 103072. DOI: 10.1016/j.jcs.2020.103072.
- Sun, Q.; Han, Z.; Wang, L.; Xiong, L. Physicochemical Differences between Sorghum Starch and Sorghum Flour Modified by Heat-Moisture Treatment. Food Chem. 2014, 145(Supplement C), 756–764. DOI: 10.1016/j.foodchem.2013.08.129.
- Stute, R. Hydrothermal Modification of Starches: The Difference between Annealing and Heat/Moisture -treatment. Starch - Stärke. 1992, 44(6), 205–214. DOI: 10.1002/star.19920440603.
- Wang, W.; Zhou, H.; Yang, H.; Zhao, S.; Liu, Y.; Liu, R. Effects of Salts on the Gelatinization and Retrogradation Properties of Maize Starch and Waxy Maize Starch. Food Chem. 2017, 214(Supplement C), 319–327. DOI: 10.1016/j.foodchem.2016.07.040.
- Nadiah, N.; Uthumporn, U.; Syahariza, Z. A. Effect of Microwave Heating on Potato and Tapioca Starches in Water Suspension. Int. J. Adv. Sci. Eng. Inf. Technol. 2015, 5, 264. DOI: 10.18517/ijaseit.5.4.502.
- Shen, H.; Fan, D.; Huang, L.; Gao, Y.; Lian, H.; Zhao, J.; Zhang, H. Effects of Microwaves on Molecular Arrangements in Potato Starch. RSC Adv. 2017, 7(24), 14348–14353. DOI: 10.1039/C6RA28048J.
- Zailani, M. A.; Kamilah, H.; Husaini, A.; Awang Seruji, A. Z. R.; Sarbini, S. R. Functional and Digestibility Properties of Sago (Metroxylon Sagu) Starch Modified by Microwave Heat Treatment. Food Hydrocoll. 2022, 122, 107042. DOI: 10.1016/j.foodhyd.2021.107042.
- Huang, -T.-T.; Zhou, D.-N.; Jin, Z.-Y.; Xu, X.-M.; Chen, H.-Q. Effect of Repeated Heat-Moisture Treatments on Digestibility, Physicochemical and Structural Properties of Sweet Potato Starch. Food Hydrocoll. 2016, 54(Part A), 202–210. DOI: 10.1016/j.foodhyd.2015.10.002.
- Maache-Rezzoug, Z.; Maugard, T.; Zarguili, I.; Bezzine, E.; El Marzouki, M. N.; Loisel, C. Effect of Instantaneous Controlled Pressure Drop (Dic) on Physicochemical Properties of Wheat, Waxy and Standard Maize Starches. J. Cereal Sci. 2009, 49(3), 346–353. DOI: 10.1016/j.jcs.2008.10.005.
- Palav, T.; Seetharaman, K. Impact of Microwave Heating on the Physico-Chemical Properties of a Starch–Water Model System. Carbohydr. Polym. 2007, 67(4), 596–604. DOI: 10.1016/j.carbpol.2006.07.006.
- Hoover, R.; Manuel, H. The Effect of Heat–Moisture Treatment on the Structure and Physicochemical Properties of Normal Maize, Waxy Maize, Dull Waxy Maize and Amylomaize V Starches. J. Cereal Sci. 1996, 23(2), 153–162. DOI: 10.1006/jcrs.1996.0015.
- Adebowale, K. O.; Henle, T.; Schwarzenbolz, U., and Doert, T. Food Hydrocoll;Rich, E. A., Ed., 2009 Modification and properties of African yam bean (Sphenostylis stenocarpa Hochst. Ex A. Rich.) Harms starch I: Heat moisture treatments and annealing ;Vol. 23 7 , pp 1947–1957. 7. DOI: 10.1016/j.foodhyd.2009.01.002
- Luo, Z.; Fu, X.; He, X.; Luo, F.; Gao, Q.; Yu, S. Effect of Ultrasonic Treatment on the Physicochemical Properties of Maize Starches Differing in Amylose Content. Starch - Stärke. 2008, 60(11), 646–653. DOI: 10.1002/star.200800014.
- Sharma, M.; Yadav, D. N.; Singh, A. K.; Tomar, S. K. Effect of Heat-Moisture Treatment on Resistant Starch Content as Well as Heat and Shear Stability of Pearl Millet Starch. Agric. Res. 2015, 4(4), 411–419. DOI: 10.1007/s40003-015-0177-3.
- Liao, L.; Liu, H.; Gan, Z.; Wu, W. Structural Properties of Sweet Potato Starch and Its Vermicelli Quality as Affected by Heat-Moisture Treatment. Int. J. Food Prop. 2019, 22(1), 1122–1133. DOI: 10.1080/10942912.2019.1626418.
- Uthumporn, U.; Nadiah, N.; Wee Yin, K.; Zaibunnisa, A. H.; Mat Lazim, A. Effect of Microwave Heating on Corn Flour and Rice Flour in Water Suspension. Int. Food Res J. 2016, 23(6), 2493–2503.
- Dewi, A. M.; Santoso, U.; Pranoto, Y.; Marseno, D. W. Dual Modification of Sago Starch via Heat Moisture Treatment and Octenyl Succinylation to Improve Starch Hydrophobicity. Polymers. 2022, 14(6). DOI: 10.3390/polym14061086.
- Zavareze, E. D. R.; Dias, A. R. G. Impact of Heat-Moisture Treatment and Annealing in Starches: A Review. Carbohydr. Polym. 2011, 83(2), 317–328. DOI: 10.1016/j.carbpol.2010.08.064.
- Cordeiro, M. J. M.; Veloso, C. M.; Santos, L. S.; Bonomo, R. C. F.; Caliari, M.; Fontan, R. The Impact of Heat-Moisture Treatment on the Properties of Musa Paradisiaca L. Starch and Optimization of Process Variables. Food Technol. Biotechnol. 2018, 56(4), 506–515. DOI: 10.17113/ftb.56.04.18.5490.
- Raja, M. K. C.; Sindhu, P. Properties of Steam-Treated Arrowroot (Maranta Arundinacea) Starch. Starch - Stärke. 2000, 52(12), 471–476. DOI: 10.1002/1521-379X(200012)52:12<471::AID-STAR471>3.0.CO;2-U.
- Li, S.; Ward, R.; Gao, Q. Effect of Heat-Moisture Treatment on the Formation and Physicochemical Properties of Resistant Starch from Mung Bean (Phaseolus Radiatus) Starch. Food Hydrocoll. 2011, 25(7), 1702–1709. DOI: 10.1016/j.foodhyd.2011.03.009.
- Gayary, M.; Mahanta, C. Optimization of Process Parameters of Osmotic Pressure Treatment and Heat Moisture Treatment for Rice Starch Using Response Surface Methodology. J. Food Meas. Charact. 2020, 14. DOI: 10.1007/s11694-020-00531-z.
- González, Z.; Pérez, E. Evaluation of Lentil Starches Modified by Microwave Irradiation and Extrusion Cooking. Food Res. Int. 2002, 35(5), 415–420. DOI: 10.1016/S0963-9969(01)00135-1.
- Adebowale, K. O.; Olu-Owolabi, B. I.; Olawumi, E. K.; Lawal, O. S. Functional Properties of Native, Physically and Chemically Modified Breadfruit (Artocarpus Artilis) Starch. Ind. Crops Prod. 2005, 21(3), 343–351. DOI: 10.1016/j.indcrop.2004.05.002.
- Sindhu, R.; Devi, A.; Khatkar, B. Physicochemical, Thermal and Structural Properties of Heat Moisture Treated Common Buckwheat Starches. J. Food Sci. Technol-Mysore-. 2019, 56, 2480–2489. DOI: 10.1007/s13197-019-03725-6.
- Solaesa, Á. G.; Villanueva, M.; Muñoz, J. M.; Ronda, F. Dry-Heat Treatment Vs. Heat-Moisture Treatment Assisted by Microwave Radiation: Techno-Functional and Rheological Modifications of Rice Flour. LWT. 2021, 141, 110851. DOI: 10.1016/j.lwt.2021.110851.
- Hoover, R.; Manuel, H. Effect of Heat—Moisture Treatment on the Structure and Physicochemical Properties of Legume Starches. Food Res. Int. 1996, 29(8), 731–750. DOI: 10.1016/S0963-9969(97)86873-1.
- Choi, S.; Kerr, W. Water Mobility and Textural Properties of Native and Hydroxypropylated Wheat Starch Gels. Carbohydr. Polym. 2003, 51, 1–8. DOI: 10.1016/S0144-8617(02)00083-8.
- Yadav, B. S.; Guleria, P.; Yadav, R. B. Hydrothermal Modification of Indian Water Chestnut Starch: Influence of Heat-Moisture Treatment and Annealing on the Physicochemical, Gelatinization and Pasting Characteristics. LWT - Food Sci. Technol. 2013, 53(1), 211–217. DOI: 10.1016/j.lwt.2013.02.007.
- Srichuwong, S.; Isono, N.; Jiang, H.; Mishima, T.; Hisamatsu, M. Freeze–Thaw Stability of Starches from Different Botanical Sources: Correlation with Structural Features. Carbohydr. Polym. 2012, 87(2), 1275–1279. DOI: 10.1016/j.carbpol.2011.09.004.
- Kong, X.; Zhu, P.; Sui, Z.; Bao, J. Physicochemical Properties of Starches from Diverse Rice Cultivars Varying in Apparent Amylose Content and Gelatinisation Temperature Combinations. Food Chem. 2015, 172, 433–440. DOI: 10.1016/j.foodchem.2014.09.085.