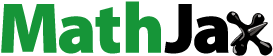
ABSTRACT
The study aimed to select the best extraction technology of Angelica dahurica polysaccharides (ADP), characterize its structure, and antioxidant activity. On the basis of the single-factor experiment result, the ultrasonic-assisted extraction (UAE) of ADP was ameliorate using the response surface method. The optimal conditions were: extraction time 32 min, extraction temperature 74°C, ratio of water to material 19 mL/g, extraction power 270 W. The practical extraction rate was 11.21 ± 0.12% (g/g). C-1: Not clear?? g/s??? ADP was purified by column chromatography, and then three polysaccharide fractions (ADP-H, ADP-0.1 and ADP-0.3) were obtained using DEAE-Cellulose 52. The ADP was mainly composed of D-Mannose, D-Glucose, D-Galactose, L-Arabinose, the ADP-H was mainly composed of D-Mannose, D-Glucose, D-Galactose, L-Arabinose, the ADP-0.1 was mainly composed of D-Mannose, D-Galacturonic acid, D-Glucose, D-Galactose, L-Arabinose, and the ADP-0.3 was mainly composed of D-Mannose, L-Rhamnose, D-Galacturonic acid, D-Galactoe, L-Arabinose. The molecular weights of ADP-H, ADP-0.1 and ADP-0.3 were 1.01 × 104 Da, 1.45 × 107 Da and 6.96 × 106 Da. In addition, the purified polysaccharides have certain scavenging ability of DPPH free radical, hydroxyl free radical and ferric reducing power, which can be used as natural products of antioxidants.
Introduction
Angelica dahurica is the dried root of Angelica dahurica (Fisch.exHoffm.) Benth.etHook.f. or A. dahurica (Fisch.exHoffm.) Benth.et Hook.f.var.formosana (Boiss.) of the umbelliferous family. Angelica tastes hot, warm, has the effect of relieving cold, dispelling wind and relieving pain, deswelling and discharging pus, nasal orifice, dryness and dampness, dispelling wind and relieving itching.[Citation1] Volatile oil, alkaloids, coumarins, polysaccharides, flavonoids and amino acids were all found in A. dahurica.[Citation2] A. dahurica is rich in nutrients as a medicinal and edible herb.[Citation3] Currently, in the edible aspect, it is mainly used as an accompaniment as well as a condiment and can be used in the formulation of nutritious foods and functional foods. Modern pharmacological studies have found that A. dahurica polysaccharides (ADP) have antioxidant, antiviral, boost body immunity, promote cell growth and other activities.[Citation4]
Hot water is usually used to extract plant polysaccharides. But the traditional solvent of water extraction is water, dilute acid or dilute alkali, it has the disadvantages of long extraction time, high temperature, waste of solvent, environmental protection and low extraction rate.[Citation5,Citation6] At present, new extraction technologies include ultrasonic-assisted extraction, enzyme-assisted extraction and microwave-assisted extraction,etc. These methods were widely used for the extraction of polysaccharides.[Citation7–11] Ultrasound-assisted extraction greatly reduces extraction time and increases extraction yield compared to conventional extraction methods.[Citation12–14]
Excess oxygen radicals can damage biological macromolecules and organelles in the organism, causing oxidative damage at the molecular, cellular and tissue organ levels, leading to aging reactions and inducing various diseases,[Citation15] and it can be scavenged from the body by reacting with natural antioxidants in the body in order to stop the chain reaction. Polysaccharides are low-toxicity free radical scavengers and antioxidants that display a crucial utility in keeping the body safe from oxidative injury.[Citation16–18]
In this study, a single-factor experiment was conditioned to investigate the efficacy of four factors, namely extraction time, extraction temperature, ultrasonic power, and the ratio of water to material on the extraction rate of ADP. The Box-Behnken design was used to optimize each extraction variable to obtain a high extraction rate of polysaccharides. The coarse polysaccharides from A. dahurica (ADP) were separated making use of a DEAE-Cellulose 52 cellulose column and the antioxidant activities of the coarse and purified polysaccharides was determined.
Materials and methods
Materials and chemicals
Angelica dahurica was obtained from Hebei Anguo Chinese Medicinal Materials Market. (Identified by Zijing Xue, Hebei University of Chinese Medicine). DEAE-52 cellulose was acquired from GE Healthcare Life Science (Uppsala, Sweden). Standard monosaccharides (including glucuronic acid, rhamnose, arabinose, fucose, xylose, mannose, glucose, galactose and inositol) were obtained from Shanghai Aladdin Bio-Technology Co. Ltd (Shanghai, China). Other reagents used in the experiments were of analytical grade.
Ultrasonic assisted extraction of polysaccharides from A. dahurica
The dried A. dahurica was smashed into debris and defatted with 80% ethanol for 2 h at 100°C, dried and crushed. A single factor experimental design was used to extract dry pretreated samples (10 g) in a KQ-300DE Ultrasonic Cleaner bath (Dimension: 24 × 30.5 × 18 cm, Kunshan Ultrasound Instrument Co., Jiangsu, China) under the conditions of extraction time (10, 30, 50, 70 min), extraction temperature (50, 60, 70, 80°C), ultrasonic power (210, 240, 270, 300 W),the ratio of water to material (10, 20, 30, 40 mL/g) and extraction times (1, 2, 3, 4 times).The mixture after the ultrasound was centrifuged at 4200 rpm (Eppendorf Centrifuge 5804/5804 R) for 10 min. Then, the concentration of supernatant was reduced to a small volume by rotary evaporator and and precipitated with 4 times the concentration of 95% ethanol for 10 hours. The precipitate was centrifuged (4200 rpm, 10 min), dissolved in distilled water and freeze-dried to obtain water-soluble coarse polysaccharides (ADP).The extraction yield of polysaccharides was calculated by following formula:
W1 is the crude polysaccharide quantity of A. dahurica (g), W0 is the dried powder quantity of A. dahurica (g).
Optimization of extraction conditions by Box-Behnken design (BBD)
BBD was used to discover the best variables for extracting ADP based on a single factor trial. These four variables were X1, X2, X3, and X4, and the three levels of the high, medium, and low were +1, 0 and −1, respectively (), as programmed by Formula. The extraction conditions were optimized a total of 29 times, as shown in , using four variables, as well as three levels of the center point. Design Expert software was used to examine the data (Version 8.0, Stat-Ease Inc., Minneapolis, USA). The relationships and interrelationships between variables are based on the following second-order polynomial model:
Table 1. Factors and levels of BBD.
Table 2. Four-factor-three-level experimental design and results of BBD.
where Y is the prediction response (crude polysaccharide extraction rate), Xi, Xj are the separate variables (as reflected in the ). β0, βi, βii and βij are the constant regression coefficients of the model.
Isolation and purification of polysaccharides
ADP was extracted according to the above extraction method. The ADP solution (25 mg/mL, 10 mL) was purified by a DEAE-52 cellulose column and eluted with pure water and NaCl (0.1 M and 0.3 M) solution (1 mL/min), respectively.[Citation19] The respective eluates were concentrated by rotary evaporation, dialyzed and lyophilized to give ADP-H, ADP-0.1 and ADP-0.3.
Structural characterization of ADP
FT-IR analysis
ADP, ADP-H, ADP-0.1 and ADP-0.3 were evenly mixed with spectral KBr solids and grounded into fine powder in a mortar. Transparent flakes were pressed in a mold and analyzed by FT-IR (Perkin Elmer S-100) spectroscopy.[Citation20]
Molecular weight
The molecular weight (Mw) of each component was performed as illustrated in the reference, with slight modification.[Citation21] The mobile phase was water, 20 μL injection volume, 0.6 mL/min elution flow rate, HPGPC (Agilent 1200), ELSD detector and TSK gel G3000 PWXL (300 mm × 7.8 mm, 10 mm) and TSK gel G4000 PWXL (300 mm × 7.8 mm, 10 mm) gel columns to determine the molecular weight of samples. T5000, T12000, T50000, T150000, and T270000 dextran standards were used to make 4 mg/mL standard samples. The molecular weight of the polysaccharide was computed using a standard curve and a sample of the same concentration.[Citation22]
Monosaccharide composition analysis
The monosaccharide compositions of ADP, ADP-H, ADP-0.1 and ADP-0.3 were determined by PMP derivatization.[Citation23] Firstly, the monosaccharide standard (10 mg) and 2 M trifluoroacetic acid (3 mL) were put in 110°C for hydrolysis for 6 h, and an appropriate amount of methanol was added for decompression concentration to removed the excess acid and Agilent 1260 Infinity HPLC instrument was used for analysis at 30°C.
Thermal analysis
The samples were heated of at a rate of 10°C/min from 20°C to 800°C and the results were measured using a DTG-60 thermogravimetric analyzer (Shimadzu Corporation, Japan). The samples were stored under a dynamic nitrogen atmosphere using a platinum crucible (50 ml/min).[Citation24]
Antioxidant activity of ADP
DPPH • scavenging activity
The DPPH • scavenging activities of ADP, ADP-H, ADP-0.1 and ADP-0.3 were determined using a reported method.[Citation25]
Hydroxyl radical scavenging assay
According to the methods stated in literature, the scavenging ability of polysaccharide on hydroxyl radical was investigated was studied.[Citation26]
Reducing power assay: The reducing power assay of the polysaccharides were determined using a reported method.[Citation27] Vc of the same mass concentration was used as positive control.
Results and discussion
Single factor experiment
Influence of ultrasonic extraction time on polysaccharide yield
As illustrated in , under the conditions of extraction temperature of 50°C, ultrasonic power of 270 W, the ratio of water to material of 20 mL/g, and extraction times of 2 times, the extraction yield of ADP showed a significant upward trend at an ultrasonic extraction temperature of 10°C ~ 70°C. After 30 min, the extraction yield increase inconspicuously. Because prolonged extraction time can be made polysaccharides dissolve better, but too long extraction time may also made polysaccharides degrade and increase the dissolution of impurities.[Citation28] Herein, extraction time around 30 min was thereby chosen for further study.
Influence of ultrasonic extraction temperature on polysaccharide yield
As detailed in figure , in this set of conditions of 30 min, 270 W, 20 mL/g and 2 times, the extraction rate increased from 7.25% to 11.14% when the ultrasonic extraction temperature ranged from 10°C to 70°C. This may be due to the higher solubility of polysaccharides due to high temperature, which could accelerate the intermolecular movement and make polysaccharides more soluble in water, allowing polysaccharides to be released from the inside of the cell to the external solvent. From 70°C to 80°C, this might be due to that at higher temperatures, the starch in the polysaccharide becomes dextrinised, making it more viscous and difficult to dissolve.[Citation29] Therefore, extraction temperature around 70°C was chosen for the next RSM analysis.
Influence of ultrasonic power on polysaccharide yield
As can be seen from , in the case of 70°C, 30 min, 20 mL/g and 2 times, the maximum extraction rate up to 11.22% when the power was 270 W, but the extraction rate decreased slightly after the power exceeded 270 W. This might be because high ultrasonic power will improve the mechanical effect of ultrasonic, and cells are effectively destroyed, thus improving the mass transfer efficiency, but the polysaccharide decomposition under high power, the yield is reduced.[Citation30] Hence, ultrasound powers around 270 W was selected for further optimization with a BBD.
Influence of the ratio of water to material on polysaccharide yield
As reflected in , at the ratio of water to material of 10 mL/g ~ 20 mL/g, the extraction rate of polysaccharide increased from 8.86% to 11.22% at the 70°C, 30 min, 270 W and 2 times, and then the extraction rate decreased slightly. This may because the ratio of liquid to the material is too small for water molecules to enter the aggregated powder and the polysaccharide cannot be completely dissolved in water.[Citation29] When the liquid material is relatively high, the extraction concentration and a density decrease, which is conducive to the better dissolution of water molecules in polysaccharides[Citation31;] However, if the liquid proportion is too high, the polysaccharide will be wasted in the process of processing.[Citation32]The ratio of water to material around 20 mL/g were optimum for further statistical optimization.
Influence of ultrasonic extraction times on polysaccharide yield
As shown in , under the conditions of 70°C, 30 min, 270 W and 20 mL/g, with the increase of extraction times from one to two times, the yield of polysaccharide increased significantly, reaching 11.22%. After that, the yield of polysaccharide began to decline, which might be due to the degradation of some polysaccharide caused by excessive extraction times. And from the perspective of energy consumption and time consumption, two extraction times are selected.[Citation33]
Optimization of BBD extraction conditions
29 runs were conducted for the BBD experiment to optimize the extraction conditions of ADP showed in . Using Design-Expert 8.0.6 software and multiple regression analysis, the response and variable values were demonstrated by the accompanying equation:
where, Y is the extraction yield of polysaccharides, X1, X2, X3 and X4 are the coding variables of extraction time, extraction temperature, the ratio of water to material and ultrasonic power, respectively. The significance and adaptability of the regression model was tested using Analysis of Variance (ANOVA) was used to test ().
Table 3. ANOVA of quadratic model for ultrasonic-assisted extraction (UAE) of ADP by response surface optimization and test the fitting of regression equation.
The significance of the regression model was assessed using F-text and the fit of the regression model was tested using ANOVA with a response surface quadratic model, as shown in . P-value was accustomed to determine the statistical significance of the coefficients and the strength of the interaction between the variables.[Citation34] The model F-value (2.90) was high and the model P (0.03) < 0.05, indicating a significant response of the model to the extraction rate. The P-value and F-values for the missing fit were 0.06 and 5.54 respectively, indicating that the model is reasonable. In addition, the validity of the model was determined by the coefficient of determination R2 (R2 = 0.74), pointing that there is a 25.65% probability that no independent parameters appear in the model. In addition, the coefficient of variation (CV) was 9.19%, which is low, indicating that the model has good accuracy and the experimental data is more reliable.[Citation35]
The linear term and the quadratic terms
,
,
,
had a considerable impact on the extraction rate of polysaccharides, according to the ANOVA. If the contour plot of the response surface is elliptical or saddle-shaped, the interactions between the variables of interest are substantial. When the contour plot is circular,[Citation36] the interactions between response surface strain variables can be neglected. As shown in , the interactions of
,
and
were significant. Design-Expert software determined the optimal extraction conditions for ADP (31.82 min, 73.91°C, 19.34 mL/g, 262.71 W). The yield of ADP was predicted by RSM to be 11.26%. The optimized extraction conditions were 32 min, 74°C, 19 mL/g, 270 W, the appropriate extraction rate of polysaccharides was 11.21 ± 0.12% (n = 3). As the actual yield largely matched the anticipate values, the RSM optimization method were verified to be effective as well as the model has applicability.
Isolation and purification of the samples
ADP was separated and purified by column chromatography on DEAE-Cellulose 52 column, the mobile phases were water and NaCl (0.1 mol/L and 0.3 mol/L), separately. The purified polysaccharides were labeled as ADP-H, ADP-0.1 and ADP-0.3.
FT-IR and glycoside bond analysis of the samples
As shown in , the absorption band located at around 3400 cm−1 was resulted from -OH tensile vibrations, at around 2926 cm−1was designated as the C-H stretching vibration,[Citation37] the band at around 1630 cm−1 was caused by -OH bending vibration,[Citation38] and the absorption band near 1420 cm−1 was designated as the characteristic absorption peak of =CH2, the absorption wavelength of ADP appeared near at 1100 cm−1 was resulted from C-O stretching vibration of alcohol hydroxyl group,[Citation39] the absorption band located at around 890 cm−1 was corresponded to ADP with β-glycosidic bonds, the absorption band located at about 850 cm−1 bespoken that the ADP involved α-glycosidic bonds.[Citation40] These results indicated that ADP, ADP-H, ADP-0.1 and ADP-0.3 have typical characteristic absorption peaks of polysaccharides.
Homogeneity and molecular weight of the samples
As shown in , the chromatogram showed that both ADP-H, ADP-0.1 and ADP-0.3 were single symmetric peaks, indicating that all the samples were homogeneous polysaccharides. The standard regression equation was (R2 = 0.9913), According to the standard regression equation, the molecular weights of ADP-H, ADP-0.1 and ADP-0.3 were 1.01 × 104 Da, 1.45 × 107 Da and 6.96 × 106 Da, respectively.
Monosaccharide composition analysis of the samples
The monosaccharide compositions of crude and purified polysaccharides were shown in and their composition ratios were shown in .
Figure 5. Monosaccharide composition of ADP, ADP-H, ADP-0.1 and ADP-0.3.
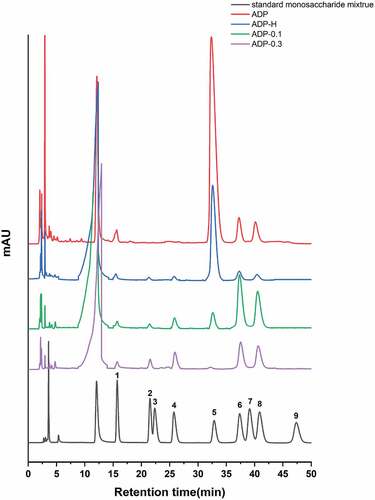
Table 4. Composition and proportion of polysaccharide monosaccharides.
Thermal characteristic
The thermal stability of biomolecules was an important characteristic of biological applications.[Citation41] It has been shown that the thermal stability of polysaccharides was usually assessed by thermogravimetric analysis (TGA), depending on changes in their structure and functional groups.[Citation25] The endothermic and exothermic conditions were shown in , presenting a three-step degradation mode. The first endothermic peak was about 20°C ~ 200°C, and the weight loss was 28.14%, which might be caused by the loss of water molecules in the structure of A. dahurica polysaccharides. The second endothermic peak was about 200°C ~ 450°C, and the weight loss is 33.66%, which may be due to thermal decomposition and the cracking of carbon and hydrogen chains. The third heat absorption peak is around 450°C ~ 800°C, with a weight loss of 12.15%, probably due to mass loss of other hard-to-degrade substances in the polysaccharide. ADP-H, ADP-0.1 and ADP-0.3 also showed the same trend. These showed that the degradation temperature of all samples were about 350°C and the total weight loss were 73.95%, 71.69%, 83.29% and 69.44% respectively, indicating that the ADP has good thermal stability.
Antioxidant activities of ADP, ADP-H, ADP-0.1 and ADP-0.3 in vitro
DPPH radical-scavenging activity
The DPPH radical has one electron and by adding a radical scavenger to accept a hydrogen ion or electron, the absorbance of DPPH decreases and the ability of the sample to scavenge radicals is evaluated.[Citation42] The higher the DPPH free radical inhibition rate, the stronger the scavenging ability. As reflected in , the DPPH free radical scavenging power of all samples was concentration-dependent but weaker than Vc. The scavenging ability of DPPH free radical was ADP > ADP-0.1 > ADP-0.3 > ADP-H, their IC50 values were 1.149, 5.537, 5.98 and 13.769, respectively. The purified polysaccharide components had almost no significant scavenging ability. This might be related to cellulose, organic acids and trace elements contained in crude polysaccharide extracts.[Citation43]
Hydroxyl radical scavenging activity
Hydroxyl radicals are strong oxidants that attack cellular phospholipids damaging cell membranes and damaging macromolecules such as lipids and nucleic acids.[Citation44] Scavenging hydroxyl radicals can effectively reduce oxidative damage and protect the organism. As reflected in , all samples had a dose-dependent hydroxyl radical scavenging capacity, with ADP having the highest scavenging capacity. However, ADP-H had a higher scavenging capacity than ADP-0.1 and ADP-0.3. The IC50 value of ADP was 2.289 mg/mL, the IC50 value of ADP-H, ADP-0.1 and ADP-0.3 were 3.532, 11.925 and 10.992, respectively. The crude polysaccharide extracts have significant antioxidant activity, but after further separation, the final purified polysaccharides showed moderate or low antioxidant activity, and the pigments, flavonoids, peptides, polyphenols and other antioxidant substances contained in crude polysaccharide extracts may also play an antioxidant role.[Citation45] Among the purified polysaccharides, the lower molecular weight polysaccharide fraction (ADP-H) had stronger antioxidant activity, this is consistent with Zhang’s conclusion that the molecular weight of the polysaccharides is too large to expose the antioxidant active components.[Citation46]
Ferric reducing power
The ferric reducing power of natural compounds scavenges free radicals in the body by generating electrons through their own reduction. This indicator is an important one to examine the antioxidant activity.[Citation47] As detailed in , the reducing ability of all samples was concentration-dependent. The reducing power was ADP-H > ADP > ADP-0.1 > ADP-0.3. The purified polysaccharides had a greater impact on the antioxidant effect, that might be mannose and glucose.[Citation16]
Conclusion
In this experiment, the ultrasonic-assisted extraction of polysaccharides from A. dahurica was optimized using the response surface method. The optimum process was achieved with an ultrasonic power of 270 W, extraction temperature of 74°C, extraction time of 32 min and the ratio of water to material 19 mL/g. The polysaccharide extraction yield was as high as 11.21 ± 0.12%. Three components (ADP-H, ADP-0.1, and ADP-0.3) were separated by DEAE-Cellulose 52 column chromatography with molecular weights of 1.01 × 104 Da, 1.45 × 107 Da and 6.96 × 106 Da, respectively. Through FI-TR, TGA and SEM analysis, the results showed that α-type glycosidic linkage and β-type glycosidic linkage existed in ADP, ADP-H, ADP-0.1 and ADP-0.3 have good thermal stability. Furthermore, the antioxidant test results showed that ADP, ADP-H, ADP-0.1 and ADP-0.3 had a certain ferric reducing power and scavenging activity on DPPH and hydroxyl free radicals.From the ferric reducing power and hydroxyl radical results, ADP-H had stronger antioxidant activity compared with other purified components, which may be related to its lower molecular weight. The monosaccharide combination containing mannose and glucose fractions had potent antioxidant activity. In conclusion, the ultrasonic-assisted extraction method can be applied to extract polysaccharides from A. dahurica.
Ethical approval
This study does not involve any human or animal testing.
Acknowledgments
This work was funded by the S & T Program of Hebei (No. H2021423057) and the Science and Technology Project of Hebei Education Department (No. ZD2022021).
Disclosure statement
No potential conflict of interest was reported by the author(s).
References
- Chinese Pharmacopoeia Commission. Pharmacopoeia of the People’s Republic of China (Part One); China Medical Science and Technology Press: Beijing, 2020.
- WU, X. S. Research Progress on Chemical Constituents and Pharmacological Activities of Yuanhu Zhitong Prescription. Chin. Traditional Herbal Drugs . 2015, 46(7),1081–1095.
- Zhou, Y.; Na, L. X. Research Progress of Homology of Radix Angelicae Dahurica [J]. Asia-Pacific Tradit. Med 2022, 18(3), 213–217.
- HU, Z. Y., and ZHOU, H. L. Research Progress on Extraction, Separation and Biological Activity of Polysaccharides from Angelica dahurica[J]. China Condiment. 2017, 42(12), 174-177.
- Wu, D. T.; Zhao, Y. X.; Guo, H.; Gan, R. Y.; Peng, L.; Gang, Z.; Liang, Z. Physicochemical and Biological Properties of Polysaccharides from Dictyophora Indusiata Prepared by Different Extraction Techniques[J]. Polymers. 2021, 13(14), 2357. DOI: 10.3390/polym13142357.
- Dobrinčić, A.; Pedisić, S.; Zorić, Z.; Jurin, M.; Roje, M.; Čož-Rakovac, R.; Dragović-Uzelac, V. Microwave Assisted Extraction and Pressurized Liquid Extraction of Sulfated Polysaccharides from Fucus Virsoides and Cystoseira Barbata. Foods. 2021, 10(7), 1481. DOI: 10.3390/foods10071481.
- Chen, X.; Zhao, L.; Hu, Q.; Xiao, J.; Kimatu, B. M.; Zheng, H. The structure–activity Mechanism of the Changes in the Physicochemical Properties of Flammulina Velutipes Polysaccharides during Ultrasonic Extraction. J. Sci. Food Agric. 2021. DOI: 10.1002/jsfa.11632.
- Jing, Y. S.; Zhang, R. J.; Li, L.; Zhang, D. S.; Liu, Y.; Wu, L. F.; Zheng, Y. G. Optimization of Ultrasonic-Assisted Extraction, Characterization, and Antioxidant Activities of Polysaccharides from Sojae Semen Praeparatum. Nat. Prod. Commun 2021, 16(5), 1934578X211020622.
- Shen, S. W.; Zhou, C.; Zeng, Y. B.; Zhang, H. T.; Hossen, M. A.; Dai, J. W.; Li, S. Q.; Qin, W.; Liu, Y. W. Structures, Physicochemical and Bioactive Properties of Polysaccharides Extracted from Panax Notoginseng Using ultrasonic/microwave-assisted Extraction. LWT. 2022, 154, 112446. DOI: 10.1016/j.lwt.2021.112446.
- Zou, X. L.; Liu, Y.; Tao, C.; Liu, Y. X.; Liu, M.; Wu, J.; Lv, Z. L. CO2 Supercritical Fluid Extraction and Characterization of Polysaccharide from Bamboo (Phyllostachys Heterocycla) Leaves. J. Food Meas 2018, 12(1), 35–44. DOI: 10.1007/s11694-017-9614-2.
- Huang, Q. Q.; Xiao, B.; Chen, S.; Zeng, J. Y.; T, J. J.; Wang, G.; Wang, W. M.; Zhang, Y. J. Effect of Enzyme-Assisted Extraction on the Chemical Properties and Antioxidant Activities of Polysaccharides Obtained from the Wood Ear Mushroom, Auricularia Auricula (Agaricomycetes). Int. J. Med. Mushrooms 2022, 24(2), 49–62. DOI: 10.1615/IntJMedMushrooms.2021041906.
- Guo, X. H.; Liu, S. H.; Wang, Z. K.; Zhang, G. X. Ultrasonic-assisted Extraction of Polysaccharide from Dendrobium Officinale: Kinetics, Thermodynamics and Optimization. Biochem. Eng 2022, 177, 108227. DOI: 10.1016/j.bej.2021.108227.
- Chemat, F.; Rombaut, N.; Sicaire, A. G.; Meullemiestre, A.; Fabiano-Tixier, A. S.; Abert-Vian, M. Ultrasound Assisted Extraction of Food and Natural Products. Mechanisms, Techniques, Combinations, Protocols and Applications. A Review. Ultrason. Sonochem 2017, 34, 540–560. DOI: 10.1016/j.ultsonch.2016.06.035.
- Zhang, Z. Y.; Hou, T. Y. Optimization of Ultrasound‐assisted Extraction of Polysaccharides from Perilla Seed Meal by Response Surface Methodology: Characterization and in Vitro Antioxidant Activities. J. Food Sci 2021, 86(2), 306–318. DOI: 10.1111/1750-3841.15597.
- Wang, Y. L.; Zhao, Y.; Andrae-Marobela, K.; Okatch, H.; Xiao, J. B. Tea Polysaccharides as Food Antioxidants: An Old Woman’s Tale? Food Chem 2013, 138(2–3), 1923–1927. DOI: 10.1016/j.foodchem.2012.09.145.
- Gu, J. Y.; Zhang, H. H.; Wen, C. T.; Zhang, J. X.; He, Y. Q.; Ma, H. L.; Duan, Y. Q. Purification, Characterization, Antioxidant and Immunological Activity of Polysaccharide from Sagittaria Sagittifolia L. Food Res. Int 2020, 136, 109345. DOI: 10.1016/j.foodres.2020.109345.
- Zhou, S. Y.; Huang, G. L.; Chen, G. Y. Extraction, Structural Analysis, Derivatization and Antioxidant Activity of Polysaccharide from Chinese Yam. Food Chem 2021, 361, 130089. DOI: 10.1016/j.foodchem.2021.130089.
- Chen, L.; Long, R.; Huang, G. L.; Huang, H. L. Extraction and Antioxidant Activities in Vivo of Pumpkin Polysaccharide. Ind. Crops Prod 2020, 146, 112199. DOI: 10.1016/j.indcrop.2020.112199.
- Luan, F.; Ji, Y. F.; Peng, L. X.; Liu, Q.; Cao, H. J.; Yang, Y.; He, X. R.; Zeng, N. Extraction, Purification, Structural Characteristics and Biological Properties of the Polysaccharides from Codonopsis Pilosula: A Review. Carbohydr. Polym 2021, 261, 117863. DOI: 10.1016/j.carbpol.2021.117863.
- Yin, Z. H.; Zhang, J. J.; Guo, Q. F.; Sun, K.; Chen, L.; Zhang, W.; Yang, B. C.; Kang, W. Y. Two Novel Heteroglycan with Coagulant Activity from Flowers of Cercis Chinensis Bunge. J. Mol. Struct. 2021, 1243, 130756. DOI: 10.1016/j.molstruc.2021.130756.
- Hu, H. B.; Liang, H. P.; Wang, Y. F.; Yuan, R. N.; Sun, J.; Zhang, L. L.; Lu, Y. N. Ultrasound‐Assisted Extraction of Water‐Soluble Polysaccharides from the Fruit of Acanthopanaxbrachypus: Physicochemical, Structural and Functional Properties. Chem. Biodivers. 2021, 18(6), e2000947. DOI: 10.1002/cbdv.202000947.
- Lv, Q. Q.; Cao, J. J.; Liu, R.; Chen, H. Q. Structural Characterization, α-amylase and α-glucosidase Inhibitory Activities of Polysaccharides from Wheat Bran. Food Chem 2020, 341, 128218. DOI: 10.1016/j.foodchem.2020.128218.
- Yan, J. K.; Yu, Y. B.; Wang, C.; Cai, W. D.; Wu, L. X.; Yang, Y.; Zhang, H. N. Production, Physicochemical Characteristics, and in Vitro Biological Activities of Polysaccharides Obtained from Fresh Bitter Gourd (Momordica Charantia L.) via Room Temperature Extraction Techniques. Food Chem 2021, 337, 127798. DOI: 10.1016/j.foodchem.2020.127798.
- Chen, G. J.; Fang, C. C.; Chen, X. H.; Wang, Z. R.; Liu, M.; Kan, J. Q. High-pressure ultrasonic-assisted Extraction of Polysaccharides from Mentha Haplocalyx: Structure, Functional and Biological Activities. Ind. Crops Prod 2019, 130, 273–284. DOI: 10.1016/j.indcrop.2018.12.086.
- Chen, Q. Y.; Wang, R. F.; Wang, Y.; An, X. P.; Liu, N.; Song, M.; Yang, Y. P.; Yin, N.; Qi, J. W. Characterization and Antioxidant Activity of Wheat Bran Polysaccharides Modified by Saccharomyces Cerevisiae and Bacillus Subtilis Fermentation. J. Cereal Sci 2021, 97, 103157. DOI: 10.1016/j.jcs.2020.103157.
- Niu, L. L.; Wu, Y. R.; Liu, H. P.; Wang, Q.; Li, M. Y.; Jia, Q. Optimization of Extraction Process, Characterization and Antioxidant Activities of Polysaccharide from Leucopaxillus Giganteus. J. Food Meas. Charact 2021, 15(3), 2842–2853. DOI: 10.1007/s11694-021-00865-2.
- Liu, X. X.; Liu, H. M.; Yan, Y. Y.; Fan, L. Y.; Yang, J. N.; Wang, X. D.; Qin, G. Y. Structural Characterization and Antioxidant Activity of Polysaccharides Extracted from Jujube Using Subcritical Water. LWT. 2020, 117, 108645. DOI: 10.1016/j.lwt.2019.108645.
- Liu, Y.; Qiang, M. L.; Sun, Z. G.; Du, Y. Q. Optimization of Ultrasonic Extraction of Polysaccharides from Hovenia Dulcis Peduncles and Their Antioxidant Potential. Int. J. Biol. Macromol 2015, 80, 350–357. DOI: 10.1016/j.ijbiomac.2015.06.054.
- Tekinşen, K. K.; Güner, A. Chemical Composition and Physicochemical Properties of Tubera Salep Produced from Some Orchidaceae Species. Food Chem 2010, 121(2), 468–471. DOI: 10.1016/j.foodchem.2009.12.066.
- Wang, J.; Zhang, M.; Fang, Z. X. Recent Development in Efficient Processing Technology for Edible Algae: A Review. Trends Food Sci. Technol 2019, 88, 251–259. DOI: 10.1016/j.tifs.2019.03.032.
- Tian, S. Y.; Hao, C. C.; Xu, G. K.; Yang, J. J.; Sun, R. G. Optimization Conditions for Extracting Polysaccharide from Angelica Sinensis and Its Antioxidant Activities. J. Food Compost. Anal 2017, 25(4), 766–775. DOI: 10.1016/j.jfda.2016.08.012.
- Wang, Y. Y.; Wang, X. Y.; Xiong, Y.; Fan, J. F.; Zheng, Z. H.; Li, Y. F.; Dong, L. L.; Zhao, Z. Y. Extraction Optimization, Separation and Antioxidant Activity of Luffa Cylindrica Polysaccharides. Food Bioproc. Tech 2019, 116, 98–104. DOI: 10.1016/j.fbp.2019.04.014.
- Wang, X. L.; Liu, D. M., and Duan, Y. Optimization of Extraction Technology of Polyphenols from Juglans Regia Branch by Response Surface Method and Study on Its Antioxidant Activity in Vitro. China Pharm 2017, 28(22), 3124–3128.
- Zhu, W. L.; Xue, X. P.; Zhang, Z. L. Ultrasonic-assisted Extraction, Structure and Antitumor Activity of Polysaccharide from Polygonum Multiflorum. Int. J. Biol. Macromol 2016, 91, 132–142. DOI: 10.1016/j.ijbiomac.2016.05.061.
- Qader, B. S.; Supeni, E. E.; Ariffin, M. K. A.; Talib, A. A. RSM Approach for Modeling and Optimization of Designing Parameters for Inclined Fins of Solar Air Heater. Renew. Energ. 2019, 136, 48–68. DOI: 10.1016/j.renene.2018.12.099.
- Qiao, D. L.; Hu, B.; Gan, D.; Sun, Y.; Ye, H.; Zeng, X. Extraction Optimized by Using Response Surface Methodology, Purification and Preliminary Characterization of Polysaccharides from Hyriopsis Cumingii. Carbohydr. Polym 2009, 76(3), 422–429. DOI: 10.1016/j.carbpol.2008.11.004.
- Liu, Y. T.; Huang, W. M.; Han, W. Y.; Li, C.; Zhang, Z. Q.; Hu, B.; Chen, S.; Cui, P. J.; Luo, S. M.; Tang, Z. Z., et al. Structure Characterization of Oudemansiella Radicata Polysaccharide and Preparation of Selenium Nanoparticles to Enhance the Antioxidant Activities. LWT. 2021, 146, 111469. DOI: 10.1016/j.lwt.2021.111469.
- Tang, Z. M.; Li, D.; Sun, H. L.; Guo, X.; Chen, Y. P.; Zhou, S. Quantitative Control of Active Targeting of Nanocarriers to Tumor Cells through Optimization of Folate Ligand Density. Biomaterials. 2014, 35(27), 8015–8027. DOI: 10.1016/j.biomaterials.2014.05.091.
- Liu, Y. L.; Liu, Z.; Wei, J. J.; Lan, Y. Q.; Yang, S. Y.; Jin, T.; Liu, Z. Evaluation and Prediction of the Safe Distance in Liquid Hydrogen Spill Accident. Process Saf. Environ. Prot 2021, 146, 1–8. DOI: 10.1016/j.psep.2020.08.037.
- Qiu, J. Q.; Zhang, H.; Wang, Z. Y. Ultrasonic Degradation of Polysaccharides from Auricularia Auricula and the Antioxidant Activity of Their Degradation Products. LWT. 2019, 113, 108266. DOI: 10.1016/j.lwt.2019.108266.
- Rozi, P.; Abuduwaili, A.; Mutailifu, P.; Gao, Y. H.; Rakhmanberdieva, R.; Aisa, H. A.; Yili, A. Sequential Extraction, Characterization and Antioxidant Activity of Polysaccharides from Fritillaria Pallidiflora Schrenk. Int. J. Biol. Macromol 2019, 131, 97–106. DOI: 10.1016/j.ijbiomac.2019.03.029.
- Zhao, Z. Y.; Xu, X. Q.; Ye, Q. W.; Dong, L. L. Ultrasound Extraction Optimization of Acanthopanax Senticosus Polysaccharides and Its Antioxidant Activity. Int. J. Biol. Macromol 2013, 59, 290–294. DOI: 10.1016/j.ijbiomac.2013.04.067.
- Sun, Y. X.; Liu, J. C.; Kennedy, J. F. Purification, Composition Analysis and Antioxidant Activity of Different Polysaccharide Conjugates (Apps) from the Fruiting Bodies of Auricularia Polytricha. Carbohydr. Polym 2010, 82(2), 299–304. DOI: 10.1016/j.carbpol.2010.04.056.
- Feng, S. L.; Cheng, H. R.; Xu, Z.; Feng, S. C.; Yuan, M.; Huang, Y.; Liao, J. Q.; Ding, C. B. Antioxidant and anti-aging Activities and Structural Elucidation of Polysaccharides from Panax Notoginseng Root. Biochem. 2019, 78, 189–199. DOI: 10.1016/j.procbio.2019.01.007.
- Fan, J. L.; Wu, Z. W.; Zhao, T. H.; Sun, Y.; Ye, H.; Xu, R. J.; Zeng, X. X. Characterization, Antioxidant and Hepatoprotective Activities of Polysaccharides from Ilex Latifolia Thunb. Carbohydr. Polym 2014, 101, 990–997. DOI: 10.1016/j.carbpol.2013.10.037.
- Zhang, Z. S.; Wang, X. M.; Mo, X. F.; Qi, H. M. Degradation and the Antioxidant Activity of Polysaccharide from Enteromorpha Linza. Carbohydr. Polym 2013, 92(2), 2084–2087. DOI: 10.1016/j.carbpol.2012.11.096.
- Yin, C. M.; Fan, X. Z.; Fan, Z.; Shi, D. F.; Gao, H. Optimization of enzymes-microwave-ultrasound Assisted Extraction of Lentinus Edodes Polysaccharides and Determination of Its Antioxidant Activity. Int. J. Biol. Macromol 2018, 111, 446–454. DOI: 10.1016/j.ijbiomac.2018.01.007.