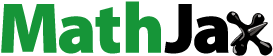
ABSTRACT
Ganoderma lucidum possesses anti-inflammatory, antioxidant, and immunomodulatory properties. We evaluated the anti-inflammatory and anti-melanogenesis effects of G. lucidum alone (S1), G. lucidum and Polygonatum odoratum (S2), and G. lucidum and P. ginseng (S3) using an ultraviolet B-irradiated HaCaT cell model and a prostaglandin 2 (PGE2)-treated B16-F10 melanocyte model. S3 inhibited PGE-2 and LL-37 secretion in HaCaT cells and melanin and tyrosinase expression in B16-F10 cells. Furthermore, LC/MS/MS results revealed that the levels of 70 components were higher in S3 than in S1 and S2. These results demonstrate that G. lucidum and P. ginseng co-culture fermentation broth possess in vitro anti-inflammatory and anti-melanogenesis effects; it can be used in the skin care industry.
Introduction
Inflammation is a complex biological response of the body to harmful stimuli. Ultraviolet (UV) rays are a major cause of skin inflammation, which leads to irreversible cell damage, photoaging, and pigmentation. UVB irradiation directly attacks DNA and generates an excessive amount of reactive oxygen species, which trigger the formation of inflammatory signaling cascades in skin cells, inducing the production of proinflammatory cytokines such as cycde-2 (COX-2) and prostaglandin 2 (PGE-2).[Citation1,Citation2] Inhibition of the production of these inflammatory molecules is a potential strategy against inflammatory diseases. PGE-2 is a major factor involved in all types of skin inflammation, which can stimulate melanocyte synthesis, increase melanin secretion, and induce pigmentation[Citation3]. In humans, melanin biosynthesis occurs in the melanocytes and tyrosinase is a rate-limiting enzyme during melanin biosynthesis. A safe and effective tyrosinase inhibitor can be a good candidate to treat hyperpigmentation.[Citation2]
Ganoderma lucidum is a fungus belonging to the family Ganodermataceae in the division Basidiomycota including umbrella fungi. Ganoderma lucidum produces a wide range of bioactive compounds, including polysaccharides, triterpenoids, phenols, steroids, vitamins, amino acids, and nucleosides during growth in fruiting bodies, mycelia, and spores.[Citation4,Citation5] Previous studies have shown that G. lucidum has anti-inflammatory,[Citation6,Citation7] antioxidant,[Citation8] anti-aging,[Citation9,Citation10] antibacterial,[Citation11] and anti-melanogenesis effects,[Citation12,Citation13] and it has application prospects in the field of skin care.[Citation14,Citation15]
At present, the main techniques used to obtain G. lucidum-derived components involve artificial cultivation and liquid fermentation. Compared to artificial cultivation, liquid-based deep fermentation technology has the advantages of short production cycles, independent of environmental influences, stable product quality, high yield, and controllable process. It constitutes a feasible approach to develop and use G. lucidum resources at a large scale in modern times.[Citation16] Therefore, it is worth investigating how to improve the utilization of G. lucidum by means of fermentation.
Compounds from G. lucidum has been demonstrated the ability for inhibiting tyrosinase (a key enzyme in melanogenesis) activity.[Citation17,Citation18] Panax ginseng exerts a whitening effect by inhibiting the transcription factors or signaling pathways involved in the formation of melanin[Citation19] Polygonatum odoratum can inhibit tyrosinase activity and control skin hyperpigmentation.[Citation20] We used liquid bidirectional fermentation technology and added Polygonatum odoratum and Panax ginseng to G. lucidum fermentation medium to improve the bioactivity of the fermentation liquid. The activity of PGE-2 and LL-37 in HaCaT cells showing high expression of inflammatory factors was evaluated after UVB irradiation to study the anti-inflammatory effects of G. lucidum fermentation broth (S1), G. lucidum and P. odoratum fermentation broth (S2), and G. lucidum and P. ginseng fermentation broth (S3). Subsequently, a B16-F10 melanocyte model was established via PGE-2 induction to study the inhibitory effects of S1, S2, and S3 on pigments after inflammation. Finally, the components of S1, S2, and S3 were detected and quantitatively analyzed using liquid chromatography (LC)/mass spectrometry (MS) technology to study the mechanisms associated with the increase in activity. This study provides a theoretical support for the inhibitory effect of G. lucidum fermentation liquid on inflammation and melanin.
Materials and methods
Fungal strains and materials
Ganoderma lucidum (CICC14023) strain was obtained from the China Center of Industrial Culture Collection (CICC). Polygonatum odoratum was purchased from Tongrentang (Beijing, China), and P. ginseng was purchased from Anguo (Henan, China). HaCaT cells and B16-F10 mouse melanoma cells were purchased from the National laboratory cell resource-sharing platform (NICR, Beijing, China). Dulbecco’s modified Eagle’s medium (DMEM), penicillin–streptomycin (PS), heat-inactivated fetal bovine serum (FBS), and trypsin EDTA Solution A (0.25% trypsin and 0.02% EDTA) were obtained from Gibco (Thermo Fisher Scientific, Waltham, MA, USA). Human PGE2 enzyme-linked immunosorbent assay (ELISA) kit was obtained from Biotechwell (Shanghai, China). Human LL-37 ELISA kit was obtained from Cusabio (Wuhan, China). Cyclooxygenase 2 (COX-2) kit was obtained from Beyotime Biotechnology (Shanghai, China).
Media and fermentation conditions
The mycelium of G. lucidum was preserved in Beijing Key Laboratory of Plant Resources Research and Development (Beijing Technology and Business University, Beijing, China) at 4°C. The seed fermentation medium for G. lucidum comprised 5 g/L glucose, 7.5 g/L soya peptone, 45 g/L cassava flour, 2.5 g/L yeast extract powder, 0.5 g/L MgSO4 · 7H2O, 1 g/L KH2PO4, and 0.05 g/L vitamin B1 (VB1). Mycelia were cut into 1-cm2 sections and inoculated into liquid fermentation medium. The mycelia were cultured at 30°C with 160 rpm shaking for 1 week.
The fermentation medium for S1 was the same as the seed medium. The fermentation medium for S2 comprised 10 g/L P. odoratum powder, 5 g/L glucose, 7.5 g/L soya peptone, 45 g/L cassava flour, 1 g/L KH2PO4, 2.5 g/L yeast extract powder, 0.5 g/L MgSO4 · 7H2O, and 0.05 g/L VB1. The fermentation medium for S3 comprised 10 g/L P. ginseng powder, 5 g/L glucose, 7.5 g/L soya peptone, 45 g/L cassava flour, 1 g/L KH2PO4, 2.5 g/L yeast extract powder, 0.5 g/L MgSO4 · 7H2O, and 0.05 g/L VB1. The fermentation medium was sterilized at 121°C and then inoculated with G. lucidum seed fermentation broth, following incubation in a 30°C and 200-rpm shaker for 1 week.
After 7 d of fermentation, the fermentation liquid was ultrasonicated at 60°C for 30 min. The filtrates of S1, S2, and S3 were filtered through a 0.22-μm micropore filter (Junhui, Shandong, China). The filtrate was collected and lyophilized using a freeze dryer (−80°C) for 48 h before storage.
Measurement of COX-2 activity
The effect of S1, S2, and S3 on cyclooxygenase activity was determined using the COX-2 Inhibitor Screening Kit according to the manufacturer’s instructions. A blank control group, 100% enzyme activity control group, positive inhibitor control group, and sample group were set up. Cox-2 assay buffer, COX-2 Cofactor working solution, COX-2 working solution, sample, and Celecoxib solution were added to a 96-well plate according to the operating instructions and incubated at 37°C for 10 min. Subsequently, 5 µL of COX-2 probe and 5 µL of COX-2 substrate were added. Fluorescence intensity was determined after incubating the samples at 37°C for 5 min away from light. The excitation wavelength was 560 nm and the emission wavelength was 590 nm. The rate of inhibition of each sample was calculated as follows:
where, RFU is the relative fluorescence unit.
Cell culture
HaCaT cells were cultured in DMEM containing 10% FBS and 1% PS at 37°C and 5% CO2 until 80%–90% confluence was reached, and then subcultured. The culture medium was removed, and the cultures were washed twice with PBS. Conventional digestion was performed using 0.25% trypsin for 8 min, and then digestion was rapidly terminated using culture medium. After centrifugation at 1,000 rcf for 5 min, the cells were suspended in culture medium for further culturing. The cultured cells were used in experiments when they reached approximately 80% confluence.
B16-F10 mouse melanoma cells were cultured in DMEM containing 10% FBS and 1% PS at 37°C and 5% CO2 until 80%–90% confluence was attained, and then subcultured. Conventional digestion was performed using 0.25% trypsin for 2 min, and then digestion was rapidly terminated using culture medium. After centrifugation, the cells were suspended in culture medium for further culturing. The cultured cells were used for experiments when they reached approximately 80% confluence.
Cell model
HaCaT cells were inoculated in six-well plates at a density of 6 × 105 cells/well. When HaCaT cells reached 80%–90% confluence, the culture medium was removed, and the culture was washed twice with PBS. Subsequently, HaCaT cells were covered with PBS and irradiated with 25, 50, 75, 100, and 125 mJ/cm2 using ultraviolet phototherapy (Kernel, Nanjing, China). PBS was removed from the wells immediately after irradiation, and an appropriate amount of serum-free medium was added. The culture was incubated at 37°C in a 5% CO2 environment for 24 h. The cell survival rate and the expression level of PGE-2 were detected under different UVB irradiation doses to determine the best radiation dose of UVB.
B16-F10 cells were inoculated in 24-well plates at a density of 3 × 105 cells/well for 24 h. PGE-2 solution (100, 80, 40, 20, 10, or 5 μM) was added following culturing for 36 h. The cell survival rate and melanin content under different concentrations of PGE-2 were detected to determine the optimal induction concentration of PGE-2.
Cell viability assay
Cell viability was determined using the cell counting kit-8 (CCK-8) assay. HaCaT cells were pretreated with different doses of UVB or different concentrations of S1, S2, and S3. After incubation at 5% CO2 and 37°C for 24 h, the CCK-8 solution was added to the cells under dark conditions. Subsequently, the CCK-8 reaction was performed at 37°C for 1 h, and the absorbance of the samples was measured at 450 nm using a microplate reader (TECAN; Männedorf, Switzerland) to calculate the cell survival rate.
B16-F10 cells were pretreated with various concentrations of PGE2, S1, S2, and S3. After incubation at 5% CO2 and 37°C for 24 h, the CCK-8 solution was added under dark conditions. Subsequently, the reaction was performed at 37°C for 1 h, and the absorbance of the samples was measured at 450 nm using a microplate reader to calculate the cell survival rate.
Measurement of relative melanin content
Two vials of B16-F10 cells showing logarithmic growth were subjected to subculturing. Two vials of cells were added to a medium containing 100 μM PGE-2, and the other two vials were added to a medium without PGE-2 and cultured at 37°C and 5% CO2 environment for 24 h. Subsequently, B16-F10 cells at two culture conditions were inoculated in 24-well plates for overnight culture. Fermentation broths were added, and PGE-2-free medium was used as a blank control. The melanin content was detected after 36 h of culture in an incubator at 37°C and 5% CO2.
The collected B16-F10 cells were centrifuged at 4°C and 1,000 rpm for 5 min. After adding 300 µL of 1 M NaOH cracking solution (including 10% dimethyl sulfoxide), the cultures were placed in a water bath at 80°C for 30 min. After allowing the samples to stand for 30 min, their absorbance was measured at 475 nm. The total protein concentration was measured using a bicinchoninic acid assay kit.The relative melanin content was calculated using to the following formula: The relative melanin content = (OD475sample/protein concentration)/(OD475control/protein concentration) × 100.
Measurement of tyrosinase level
B16-f10 cells were cultured and treated with samples as described in 2.7, and cultured for 36 h in an incubator at 37°C with 5% CO2. The collected B16-F10 cells were centrifuged at 4°C and 1,000 rpm for 5 min, and 200 μL of the lysate was added to each tube of cells to lyse the cells. Thereafter, the supernatant was collected after centrifugation at 4°C and 1,000 rpm for 10 min to determine the tyrosinase level. A mice tyrosinase (TyR) ELISA kit was used to measure the tyrosinase level according to the kit instructions, and the OD value at 450 nm was measured using a microplate reader. The tyrosinase level was calculated according to the generated curve.
Measurement of the PGE2 and LL-37 levels using ELISA
HaCaT cells were assigned to the blank control group, UVB irradiation group, and experimental group (sample solution added after UVB irradiation). HaCaT cells were inoculated in six-well plates at a density of 6 × 105 cells/well. When the cells reached 80%–90% confluence, the culture medium was removed, and cultures were washed with 1 mL PBS twice. Subsequently, 1 mL of PBS was added to cover the cells in each well before UVB radiation. The PBS buffer in the wells was removed at the end of irradiation, and an appropriate amount of serum-free medium or sample solution was added. The cultures were incubated at 37°C and 5% CO2 for 24 h. The supernatant was collected for testing. An ELISA kit was used to detect the expression of PGE-2 and LL-37.
Analysis of the compounds in S1, S2, and S3
The 150 compounds present in S1, S2, and S3 were analyzed using LC-MS/MS. S1, S2, and S3 were dissolved in 0.1% formic acid (FA) and water, and then diluted 30 times via centrifugation for sample analysis. The chromatography separation conditions were as follows: chromatographic column, T3 1.8 μm, 100 × 2.1 mm; mobile phase, A: water (0.1% FA), B: acetonitrile (0.1% FA); column temperature, 40°C; flow rate, 0.3 mL/min; injection volume, 1 µL; elution procedure, as described in ; detection time, 20 min.
Table 1. Gradient elution procedure.
The mass spectrometry analytical conditions were as follows: ion source, Turbo V; ionization mode, electrospray ionization, positive and negative ion-switching mode; ion source parameters, IS voltage: 5500.0 V, Curtain Gas: 35.0 psi, ion source gas 1: 60 psi, ion source gas 2: 60 psi, ion source temperature: 600°C, collision gas: medium.
Data analysis
The data were analyzed using a one-way analysis of variance with IBM SPSS Statistics 26.0 software (IBM, Armonk, NY, USA). Values with p < .05 were considered significant.
Results and discussion
Inhibition of COX-2 activity
Skin inflammation is closely related to the secretion of COX-2.[Citation21] The expression of COX-2 in the normal physiological state is almost absent or negligible; however, COX-2 expression is induced when skin inflammation occurs. Therefore, the anti-inflammatory effect of the samples was evaluated based on the inhibition rate of COX-2 activity. The inhibitory effects of S1, S2, and S3 on in vitro COX-2 activity were determined. S1, S2, and S3 inhibited COX-2 activity at a concentration of 2%, 4%, and 10%, respectively, in a concentration-dependent manner, indicating that S1, S2, and S3 contain bioactive compounds that inhibit COX-2 activity ().
UVB-induced inflammation model
Firstly, the effect of different doses of UVB irradiation on the viability of HaCaT cells was measured using the CCK-8 assay (). When the UVB dose was 25, 50, 75, and 100 mJ/cm2, the cell survival rate was >80%. The cell survival rate decreased with an increase in UVB intensity. The cell survival rate was 75.02% after irradiation at 125 mJ/cm2. Therefore, the UVB dose associated with a cell survival rate of >80% was selected as the optimal dose.
PGE-2 production increased significantly at a UVB irradiation dose of 50–100 mJ/cm2 compared with that in the non-UVB group (p < .01; ). The PGE-2 level was 269.52 pg/mL at 100 mJ/cm2 UVB irradiation. UVB irradiation considerably induced the secretion of the inflammatory factor PGE-2. Therefore, the optimal irradiation dose for developing a UVB-induced inflammation model is 100 mJ/cm2.
HaCaT cell viability after sample treatment
The effect of S1, S2, and S3 on the viability of HaCaT cells was measured. The cell survival rate decreased with increasing concentrations of the three samples (). S1 and S3 did not show toxicity in HaCaT cells at 250 μg/mL. S2 did not show toxicity in HaCaT cells at 100 μg/mL. Therefore, S1, S2, and S3 were used at 100, 50, and 25 μg/mL in the subsequent experiment.
Effect of S1, S2, and S3 on PGE-2 and LL-37 levels
As a major factor in skin inflammation[Citation22] and UV-induced inflammatory responses, PGE-2 can induce pigmentation by stimulating melanocyte synthesis and melanin secretion. Meanwhile, LL-37 mediates innate immunity in skin and plays an important role in skin barrier function and inflammation[Citation23] by inhibiting the expression of the UVB radiation-associated human keratinocyte nuclear factor NF-κB and, thereby potentially inhibiting COX-2 synthesis. Thus, LL-37 is speculated to inhibit NF-κB expression, thereby inhibiting both COX-2 synthesis and PGE-2 expression.[Citation23]
Compared with that in the non-UVB group (BC), the secretion level of PGE-2 in the UVB group was significantly increased (p < .01; ), indicating that the UVB stimulation conditions were effective in this experiment. UVB irradiation significantly promoted the secretion of PGE-2. Compared to that in the UVB group (NC), the secretion of PGE-2 after treatment with S1 and S2 was decreased at a concentration of 100 μg/mL; however, there was no significant difference. The secretion of PGE-2 in HaCaT cells was decreased after S3 treatment (p < .01). Therefore, S3 significantly inhibited the activity of PGE-2 and had an anti-inflammatory effect.
Figure 5. Effect of S1, S2, and S3 at different concentrations on PGE-2 production. PGE-2, prostaglandin E2; S1, Ganoderma lucidum fermentation broth; S2, G. lucidum and Polygonatum odoratum fermentation broth; S3, G. lucidum and Panax ginseng fermentation broth. *P < 0.05, **P < 0.01.
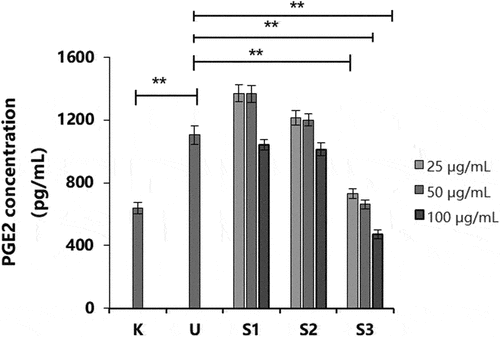
Compared with the UVB group (NC), the S1 and S3 treatments inhibited LL-37 secretion at 100 μg/mL (p < .05; ). However, the S1 treatment significantly increased LL-37 secretion at a concentration of 50 μg/mL (p < .01). Treatment with S3 inhibited the increase in PGE-2 level and regulated the secretion of the antibacterial peptide LL-37. The aforementioned experiments showed that UVB irradiation could stimulate HaCaT cells to produce the inflammatory factor PGE-2, thus inducing the increased secretion of antibacterial peptide LL-37. S3 treatment inhibited the production of PGE-2 and regulated the secretion of antibacterial peptide LL-37 to a normal level, which had an anti-inflammatory effect.
PGE-2-induced melanocyte model
The effect of different concentrations of PGE-2 on the viability of B16-F10 cells was determined using the CCK-8 assay. When the PGE-2 concentration was 10, 20, 40, or 80 μM, no toxicity was observed in B16-F10 cells, and the cell survival rate was >80% (). The melanin content in B16-F10 cells increased with an increase in PGE-2 concentration (). When the PGE-2 concentration was 100 μM, the melanin content was the highest (129.39% ± 15.02%). Therefore, PGE-2 at a concentration of 100 μM was used to induce the production of melanin in B16-F10 cells.
Cell viability after sample treatment and inhibition of cellular melanin synthesis
PGE-2 is a lipid mediator released by keratinocytes in response to UV radiation in the skin and plays an important role in inflammation and immunity.[Citation24] PGE-2 affects the expression of cyclic adenosine monophosphate (cAMP) in melanocytes via the relative level or activity of EP receptors, and increased cAMP expression results in post-inflammatory hyperpigmentation.[Citation25]
B16-F10 cells treated with S1, S2, and S3 at 50 and 100 μg/mL did not show a considerable reduction in cell viability (). The concentration at which the cell survival rate was >80% was selected as the optimal concentration. Therefore, a concentration of 100 μg/mL was used to determine the effects of the three samples on PGE-2-induced melanin content. The PGE-2-induced melanin content significantly increased by 66.67% (p < .05; ). At a concentration of 100 μg/mL, S1 and S3 inhibited melanin production in PGE-2-stimulated B16-F10 cells (p < .01). In contrast, S2 treatment increased melanin synthesis (p < .01).
Effects of S1, S2, and S3 on tyrosinase level
We further studied the effects of S1, S2, and S3 on the tyrosinase level. At 100 μg/mL, the S1 and S3 treatments significantly reduced the tyrosinase level (p < .01; ). However, the S2 treatment promoted the synthesis of tyrosinase. Our experimental results further showed that S3 had anti-melanogenesis effect by inhibiting the expression of tyrosinase.
Chemical composition of S1, S2, and S3
To determine the mechanism associated with the increase in anti-inflammatory and anti-melanogenesis activities, 150 compounds in S1, S2, and S3 were quantitatively analyzed using LC-MS/MS, and 112 compounds were detected in total (). In this experiment, the linear relationship of compounds was good and in the range of 1–1000 ng/mL (r > 0.995). Most compounds showed good stability after three injections (relative standard deviation < 5%). The results showed that the three samples were rich in carbohydrates, vitamins, amino acids, proteins, nucleotides, antibiotics, trace elements, and other compounds (). Among them, 6 types of carbohydrates, 19 types of vitamins, 49 types of amino acids, 26 types of nucleotides, 1 type of antibiotic, and 34 types of other compounds were identified.
Table 2. Carbohydrate compositions of S1, S2, and S3.
Table 3. Vitamin contents in S1, S2, and S3.
Table 4. Amino acid contents in S1, S2, and S3.
Table 5. Nucleotide composition of S1, S2, and S3.
Table 6. Antibiotic contents in S1, S2, and S3.
Table 7. Composition of other compounds in S1, S2, and S3.
Skin pigmentation can be reduced by many substances, the most common being niacinamide, vitamin C, arbutin, kojic acid, alpha hydroxyl acid, and glutathione,[Citation26] and nicotinamide has been reported to reduce melanin accumulation in the cuticle by reducing the transport of melanin to the extracellular layer.[Citation27] Arbutin, kojic acid, fruit acid, and glutathione can inhibit tyrosinase, thereby reducing the synthesis of melanin by melanocytes,[Citation28] and glutathione can be hydrolyzed to cysteine, which predisposes the melanin synthesis pathway to the production of lighter-colored melanin.[Citation29] Additionally, vitamin C can reduce dopamine quinone to dopamine, which blocks the oxidation chain of melanin production and inhibits the formation of melanin. The LC-MS/MS analysis showed that the contents of 70 components in S3 were higher than those in the S1 and S2. Among them, the contents of L-glutathione, vitamin C, niacinamide, citric acid, isocitrate, lactic acid, DL-malic acid, and other constituents were considerably increased, which had the effect of reducing skin pigmentation. Therefore, the increase of these whitening active ingredients may promote the inhibition of melanin by S3. It is necessary to analyze further the active ingredients after fermentation to study the mechanism associated with the increased bioactivity of S3. Together, these results provide a basis for the application of G. lucidum and P. ginseng co-culture fermentation liquid in anti-inflammatory and anti-melanogenesis skin care products.
Conclusion
In this study, a G. lucidum strain was cultivated together with P. odoratum or P. ginseng in bidirectional liquid fermentation to improve the activity of the fermentation broth. Treatment with S1, S2, and S3 showed that S3 inhibited the production of PGE2 and regulated the secretion of antibacterial peptide LL-37, which had a significant anti-inflammatory effect in HaCaT cells. Additionally, S3 was found to inhibit melanin production in B16-F10 melanoma tumor cells stimulated by PGE-2, indicating that G. lucidum and P. ginseng co-culture fermentation liquid possesses in vitro anti-inflammatory and anti-melanogenesis effects. Moreover, the inhibitory effect of S3 on melanin synthesis may be related to the enhancement of whitening active ingredients such as L-glutathione, vitamin C, niacinamide, and alpha hydroxyl acid. The novel effects reported herein provide a theoretical support for the development of new raw materials for skin care products.
Acknowledgments
We would like to thank Editage (www.editage.cn) for English language editing.
Disclosure statement
No potential conflict of interest was reported by the author(s).
Data availability statement
The raw data required to reproduce these findings cannot be shared at this time as the data also forms part of an ongoing study.
Additional information
Funding
References
- Kunchana, K.; Jarisarapurin, W.; Chularojmontri, L.; Wattanapitayakul, S. K. Potential use of amla (Phyllanthus Emblica L.) Fruit extract to protect skin keratinocytes from inflammation and apoptosis after UVB irradiation. Antioxidants. 2021, 10, 703. DOI: 10.3390/antiox10050703.
- Wang, L.; Oh, J. Y.; Jayawardena, T. U.; Jeon, Y. J.; Ryu, B. Anti-inflammatory and anti-melanogenesis activities of sulfated polysaccharides isolated from Hizikia Fusiforme: Short communication. Int. J. Biol. Macromol. 2020, 142, 545–550. DOI: 10.1016/j.ijbiomac.2019.09.128.
- Fu, C.; Chen, J.; Lu, J.; Yi, L.; Tong, X.; Kang, L.; Pei, S.; Ouyang, Y.; Jiang, L.; Ding, Y.; et al. Roles of inflammation factors in melanogenesis (Review). Mol. Med. Rep. 2020, 21, 1421–1430. DOI: 10.3892/mmr.2020.10950.
- Chaturvedi, V. K.; Agarwal, S.; Gupta, K. K.; Ramteke, P. W.; Singh, M. P. Medicinal mushroom: boon for therapeutic applications. 3 Biotech. 2018, 8, 334. DOI: 10.1007/s13205-018-1358-0.
- Boh, B.; Berovic, M.; Zhang, J.; Zhi-Bin, L. Ganoderma Lucidum and its pharmaceutically active compounds. Biotechnol. Annu. Rev. 2007, 13, 265–301. DOI: 10.1016/s1387-2656(07)13010-6.
- Wu, Y. L.; Han, F.; Luan, S. S.; Ai, R.; Zhang, P.; Li, H.; Chen, L. X. Triterpenoids from Ganoderma Lucidum and their potential anti-inflammatory effects. J. Agric. Food Chem. 2019, 67, 5147–5158. DOI: 10.1021/acs.jafc.9b01195.
- Lu, S. Y.; Peng, X. R.; Dong, J. R.; Yan, H.; Kong, Q. H.; Shi, Q. Q.; Li, D. S.; Zhou, L.; Li, Z. R.; Qiu, M. H. Aromatic constituents from Ganoderma Lucidum and their neuroprotective and anti-inflammatory activities. Fitoterapia. 2019, 134, 58–64. DOI: 10.1016/j.fitote.2019.01.013.
- Abate, M.; Pepe, G.; Randino, R.; Pisanti, S.; Basilicata, M. G.; Covelli, V.; Bifulco, M.; Cabri, W.; D’Ursi, A. M.; Campiglia, P.; et al. Ganoderma Lucidum ethanol extracts enhance re-epithelialization and prevent keratinocytes from free-radical Injury. Pharmaceuticals. 2020, 13, 224. DOI: 10.3390/ph13090224.
- Cuong, V. T.; Chen, W.; Shi, J.; Zhang, M.; Yang, H.; Wang, N.; Yang, S.; Li, J.; Yang, P.; Fei, J. The anti-oxidation and anti-aging effects of Ganoderma Lucidum in Caenorhabditis Elegans. Exp. Gerontol. 2019, 117, 99–105. DOI: 10.1016/j.exger.2018.11.016.
- Wang, J.; Cao, B.; Zhao, H.; Feng, J. Emerging roles of Ganoderma Lucidum in anti-aging. Aging Dis. 2017, 8, 691–707. DOI: 10.14336/ad.2017.0410.
- Jiao, C.; Xie, Y.; Yun, H.; Liang, H.; He, C.; Jiang, A.; Wu, Q.; Yang, B. B. The effect of Ganoderma Lucidum spore oil in early skin wound healing: interactions of skin microbiota and inflammation. Aging. 2020, 12, 14125–14140. DOI: 10.18632/aging.103412.
- Hu, S.; Huang, J.; Pei, S.; Ouyang, Y.; Ding, Y.; Jiang, L.; Lu, J.; Kang, L.; Huang, L.; Xiang, H.; et al. Ganoderma Lucidum polysaccharide inhibits UVB-induced melanogenesis by antagonizing cAMP/PKA and ROS/MAPK signaling pathways. J. Cell. Physiol. 2019, 234, 7330–7340. DOI: 10.1002/jcp.27492.
- Jiang, L.; Huang, J.; Lu, J.; Hu, S.; Pei, S.; Ouyang, Y.; Ding, Y.; Hu, Y.; Kang, L.; Huang, L.; et al. Ganoderma Lucidum polysaccharide reduces melanogenesis by inhibiting the paracrine effects of keratinocytes and fibroblasts via IL-6/STAT3/FGF2 pathway. J. Cell. Physiol. 2019, 234, 22799–22808. DOI: 10.1002/jcp.28844.
- Yin, Z.; Yang, B.; Ren, H. Preventive and therapeutic effect of Ganoderma (Lingzhi) on skin diseases and care. Adv. Exp. Med. Biol. 2019, 1182, 311–321. DOI: 10.1007/978-981-32-9421-9_14.
- Ahmad, R.; Riaz, M.; Khan, A.; Aljamea, A.; Algheryafi, M.; Sewaket, D.; Alqathama, A. Ganoderma Lucidum (Reishi) an edible mushroom; a comprehensive and critical review of its nutritional, cosmeceutical, mycochemical, pharmacological, clinical, and toxicological properties. Phytother Res. 2021, 35, 6030–6062. DOI: 10.1002/ptr.7215.
- Feng, J.; Feng, N.; Tang, Q.; Liu, Y.; Tang, C.; Zhou, S.; Wang, J.; Tan, Y.; Zhang, J.; Lin, C. C. Development and optimization of the triterpenoid and sterol production process with lingzhi or reishi medicinal mushroom, Ganoderma Lucidum Strain G0017 (Agaricomycetes), in liquid submerged fermentation at large scale. Int. J. Med. Mushrooms. 2021, 23, 43–53. DOI: 10.1615/IntJMedMushrooms.2021037830.
- Lai, Y. J.; Hsu, K. D.; Huang, T. J.; Hsieh, C. W.; Chan, Y. H.; Cheng, K. C. Anti-melanogenic effect from submerged mycelial cultures of ganoderma weberianum. Mycobiology. 2019, 47, 112–119. DOI: 10.1080/12298093.2019.1568680.
- Hsu, K. D.; Chen, H. J.; Wang, C. S.; Lum, C. C.; Wu, S. P.; Lin, S. P.; Cheng, K. C. Extract of ganoderma formosanum mycelium as a highly potent tyrosinase inhibitor. Sci. Rep. 2016, 6, 32854. DOI: 10.1038/srep32854.
- Meng, H.; Liu, X. K.; Li, J. R.; Bao, T. Y.; Yi, F. Bibliometric analysis of the effects of ginseng on skin. J. Cosmet. Dermatol. 2022, 21, 99–107. DOI: 10.1111/jocd.14450.
- Ye, Y.; Chou, G. X.; Mu, D. D.; Wang, H.; Chu, J. H.; Leung, A. K.; Fong, W. F.; Yu, Z. L. Screening of Chinese herbal medicines for antityrosinase activity in a cell free system and B16 cells. J. Ethnopharmacol. 2010, 129, 387–390. DOI: 10.1016/j.jep.2010.04.009.
- Rundhaug, J. E.; Fischer, S. M. Cyclo-oxygenase-2 plays a critical role in UV-induced skin carcinogenesis. Photochem. Photobiol. 2008, 84, 322–329. DOI: 10.1111/j.1751-1097.2007.00261.x.
- Heard, C. M. An ex vivo skin model to probe modulation of local cutaneous arachidonic acid inflammation pathway. J. Biol. Methods. 2020, 7, e138. DOI: 10.14440/jbm.2020.319.
- Xhindoli, D.; Pacor, S.; Benincasa, M.; Scocchi, M.; Gennaro, R.; Tossi, A. The human cathelicidin LL-37–A pore-forming antibacterial peptide and host-cell modulator. Biochim. Biophys. Acta. 2016, 1858, 546–566. DOI: 10.1016/j.bbamem.2015.11.003.
- Wan, M.; Tang, X.; Rekha, R. S.; Muvva, S.; Brighenti, S.; Agerberth, B.; Haeggström, J. Z. Prostaglandin E(2) suppresses hCAP18/LL-37 expression in human macrophages via EP2/EP4: implications for treatment of Mycobacterium Tuberculosis infection. FASEB J. 2018, 32, 2827–2840. DOI: 10.1096/fj.201701308.
- Starner, R. J.; McClelland, L.; Abdel-Malek, Z.; Fricke, A.; Scott, G. PGE(2) Is a UVR-inducible autocrine factor for human melanocytes that stimulates tyrosinase activation. Exp Dermatol. 2010, 19, 682–684. DOI: 10.1111/j.1600-0625.2010.01074.x.
- Kanlayavattanakul, M.; Lourith, N. Plants and natural products for the treatment of skin hyperpigmentation - a review. Planta Med. 2018, 84, 988–1006. DOI: 10.1055/a-0583-0410.
- Fania, L.; Mazzanti, C.; Campione, E.; Candi, E.; Abeni, D.; Dellambra, E. Role of nicotinamide in genomic stability and skin cancer chemoprevention. Int. J. Mol. Sci. 2019, 20, 5946. DOI: 10.3390/ijms20235946.
- Gillbro, J. M.; Olsson, M. J. The melanogenesis and mechanisms of skin-lightening agents–existing and new approaches. Int. J. Cosmet. Sci. 2011, 33, 210–221. DOI: 10.1111/j.1468-2494.2010.00616.x.
- Dilokthornsakul, W.; Dhippayom, T.; Dilokthornsakul, P. The clinical effect of glutathione on skin color and other related skin conditions: a systematic review. J. Cosmet. Dermatol. 2019, 18, 728–737. DOI: 10.1111/jocd.12910.