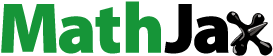
ABSTRACT
Aquatic by-products during fish processing cause environmental pollution and increase disposal costs. Cuttlefish skin is one of the major by-products of cuttlefish (Sepia pharaonis) processing and is generally thrown as a by-product. It can cause severe environmental problems and odor. It has been discovered that cuttlefish skin can be an excellent resource for producing attractive amounts of collagen. This study optimized collagen extraction conditions using response surface methodology (RSM). In addition, SDS-PAGE, specific charge, and scanning electron microscopy (SEM) characteristics of extracted collagen were evaluated. The results showed that the optimal extraction conditions for cuttlefish skin collagen are pH 1.5, 20 mg/L (solid-liquid ratio), 15 U/mg (Pepsin), and the average extraction rate can be obtained as 8.79%. The results of LC/MS/MS analysis of the collagen samples extracted in this study showed that the main m/z signal of proline produced by mass spectrometry was between 2000–4000. Collagen extracted from the cuttlefish skin is type I collagen, which consists of 2 α chains and 1 β chain (α2, α1, β). SEM analysis of collagen confirmed the presence of collagen fibrils in the cuttlefish skin similar to previous reports. Using the response surface methodology, optimal collagen extraction conditions pH 1.5, 20 mg/L (solid-liquid ratio), and 15 U/mg (Pepsin) can be obtained to recycle and utilize by-products.
Introduction
The aquatic fishery is the major economy of many countries worldwide, producing 60% of associated by-products during the processing. The main by-products of the fish processing industry, including head shells, fish scales, visceral fish bones, and fish eggs produced in spawning, cause pollution and disposal problems .[Citation1] The by-products contain many proteins, gelatin, and other nutrients,[Citation2] which are generally processed into low-value feed or fertilizer .[Citation3] The biotechnological processes exploit these high-quality by-products and use them to synthesize preventative and bioactive substances .[Citation4]
The food, cosmetic and pharmaceutical industries have widely used gelatin extracted from cuttlefish skin. Cuttlefish gelatin is a protein obtained by partial hydrolysis of collagen, which can form a thermo-reversible elastic gel .[Citation5] Gelatin has many uses in the food industry as a gelling agent, thickening agent, foaming agent, and emulsifier for improving the texture and organoleptic qualities of pork or octopus sausage products .[Citation6,Citation7] Because of its good film-forming properties and barrier ability, it can be used to prepare biodegradable, edible film materials with high transparency and flexibility .[Citation8,Citation9] As well as with various herbs and spices[Citation10–12] plant essential oils[Citation13,Citation14] and other henna (L. inermis) extract gelatin films, used as natural preservation during beef storage .[Citation15] Since collagen, produced from bovine or porcine sources, has not been encouraged much due to animal diseases and beliefs, collagen derived from aquaculture processing is used as a substitute for mammalian collagen[Citation2] and in cosmetic products-related applications .[Citation16]
Xu et al. (2010) reported the isolation of marine collagen and its application in skin and bone tissue engineering .[Citation17] Marine collagen has antioxidant properties and has been used in skincare products to prevent and repair damage caused by factors such as ultraviolet rays and low humidity. In addition, when a fish or sponge is damaged, its body produces beneficial collagen that can be used in pharmaceutical and cosmetic preparations .[Citation18,Citation19] Jridi et al. (2015) reported that the gel of cuttlefish collagen has wound-healing activity and may be used as a wound-healing agent .[Citation20]
Sepia pharaonis (Ehrenberg, 1831) is a widespread species from East Africa to southern Japan .[Citation21] Nesis (1987) reported that S. pharaonisp is the most crucial catch in the northern Indian Ocean and South-East Asian Squadron fisheries .[Citation22] In Taiwan’s cuttlefish industry, S. pharaonisp is the common species with enhanced economic value. By-products of the processing of Sepia pharaonis, such as the skin (9% of the total fish weight), are rich in protein. Despite the apparent economic importance of this species, little is known about the reuse of processing by-products of S. pharaonisp in the past. Cuttlefish skin gelatin, chitosan, and polysaccharides are some value-added products produced from S. pharaonis processing .[Citation23,Citation24] Generally, the thick cuttlefish skin, about 50–70% of fish have been treated as waste in the home, fish processing and refrigerated factories .[Citation25] Recently there are developments in the production of cuttlefish skin collagen. In recent years, more and more studies on different alternative sources and new functions of collagen have been reported .[Citation2,Citation26] However, most studies used only a single condition to extract cuttlefish collagen, lacking a more systematic method to evaluate the extraction yield.
Previous studies on gelatin production from Sepia officinalis fish skin have demonstrated that this protein can be used as a potential biomaterial .[Citation27] The current study attempts to optimize the process variables to obtain the highest collagen yield per gram of cuttlefish skin. Various factors like pH (M), the solvent/solid (ml/g), and pepsin (U/mg) have been optimized to achieve the maximum yield of collagen from cuttlefish skin. The results obtained have been validated using response surface methodology (RSM) based on the Box- Behnken design (BBD) method and a quadratic model correlating the parameters. Interaction effects between the variables have been studied using contour plots. Interactions between variables have been studied with contour plots. The extracted collagen samples were later subjected to various physicochemical analyses to confirm their composition and characterization.
Material and method
Materials and chemicals
Cuttlefish (Sepia pharaonis) skin collected from a local cuttlefish processing (Hong Yu Foods Co., Ltd., Kaohsiung, Taiwan). The average size of cuttlefish caught in the winter season is about 53–59 cm in length and 20–31 cm in width, the wet weight when received is ~5 kg, and the dry weight of the cuttlefish bone is ~80 g. After gently incising the skin at the outer edge of the cuttlefish body with a knife, the entire skin can be separated from the body. The cuttlefish skin was kept in ice during transportation and stored at −80°C until use. All chemicals, reagents, and porcine gastric pepsin (EC 3.4.23.1) were analytical grade and obtained from Sigma-Aldrich Chemical Company (St. Louis, MO, U.S.A).
Pre-treatment and extraction procedure
Pre-treatment and extraction methods have referred by Tan and Chang[Citation28] with some modifications. According to the three-factor experimental design, different concentrations of HCl (pH 1.3, 1.5, 1.7) have configured first and then mixed with three different concentrations of solid/solid ratio (10, 20, 30 ml/g) and three different concentrations of pepsin (5, 15, 25 U/mg) and homogenized for 5 minutes. The supernatant was then mixed with ground NaCl (adjusted to 0.9 M) and subjected to a salting-out reaction. The solution, after salting out, has centrifuged at 4°C for 15 min at 15000 × g, and the precipitate has stored for further use. Since the proteins obtained by the above method may be a mixture of different proteins, dialysis has performed. After washing with distilled water for 30 minutes before dialysis to remove the source preservation solution, the dialysis membrane was dialyzed with 0.5 M acetic acid for about 24 hours, during which the buffer solution changed at 2–3 hours, 4–5 hours until overnight. Finally, the dialyzed samples were placed in centrifuge tubes, pre-chilled at −80°C (24 hours), and then freeze-dried (48 hours).
Response surface methodology
A three-factor-three-level BBD with three replications at the center point and 15 experiments to evaluate the effects of extraction conditions used for cuttlefish skin collagen (). The coded values were correlated with the actual values as indicated in the equation.
Table 1. Coded values and independent variables used for optimization.
Where xi, xm, and di denote the variable’s value in the actual unit of the ith observation, the average of the highest and lowest variable values, and the difference between the highest and lowest variable values, respectively. After performing all experimental runs, the second-order polynomial equation is applied to fit the experimental response of the coded variables as shown in Equation 1.
Yn denotes the predicted response, Xi (Xj) denotes the infinite-scale coded value of the independent variable xi (xj), β0 denotes the model constant (intercept), βi denotes the linear regression coefficient.
βij denotes the fork product regression coefficient, βii denotes the quadratic regression coefficient. RSM is a statistical tool used to build an empirical model and to find the best set of variables for the desired response .[Citation29] It includes three-level factorial design, central composite design (CCD), Box-Behnken design (BBD), and D-optimal design. Box-Behnken (BBD) experimental design has excellent predictability among all these designs. Box Behnken Design (BBD) is employed in this work by varying three variables at three levels (−1, 0, +1). Among them, (0) was considered as the central point, (−1) as low level (below central point), and (+1) as high level (above central point). Independent variables and its different choice of levels are shown in . The range of these variables has secured from the results of the initial experiments. To evaluate the effect of independent variables on collagen extraction, 15 experiments have performed in triplicate, and the independent variables A (pH), B (solvent/solid), and C (Pepsin) have designed. The efficiency of the model and the statistical significance were analyzed using F-test and the R-test. The effect of the individual variable was analyzed by performing a detailed analysis of variance (ANOVA) on the coded level of variables. The RSM and experiments design have been done using Design-Expert, (Stat-Ease, Inc., Minneapolis, MN).
Protein estimation
Based on the modified Lowry’s method, collagen yield has quantified using UV–VIS spectrophotometer (Ultraviolet–Visible spectroscopy, Hitachi) at 650 nm .[Citation30]
Analysis of the charge ratio of collagen from cuttlefish skin
The mass to charge ratio of collagen from cuttlefish skin were analyzed according to the method by Jinal et al (2020) .[Citation31] The LC column has injected with a 3 µl sample. The mobile phase A was water with 0.1% formic acid (FA), and mobile phase B was 10% water with 90% acetonitrile and 0.1% FA. The LC running run time was 30 minutes at a flow rate of 0.2 ml/min using a detector, the chromatogram formation was determined, and the components’ mass relationships were examined.
Electrophoretic analysis
The stacking gel (4%) was prepared using 30:0.8% acrylamide: bis-acrylamide, Tris-HCl (pH 6.8), 20% SDS, 10% ammonium persulfate and TEMED. The separating gel (8%) prepared using 30:0.8% acrylamide: bisacrylamide, Tris-HCl (pH 8.8), 20% SDS, 10% ammonium persulfate and TEMED. The 3× sample loading dye composition was 25% glycine, 20% SDS, 5% β-mercaptoethanol, and 0.1% bromophenol blue. After the completion of electrophoresis, the gel was visualized using Coomassie Brilliant Blue R-250 staining solution. The SDS-PAGE gel was run with the help of Mini-PROTEAN electrophoretic system.
SEM analysis of cuttlefish skin collagen
SEM (Scanning Electron Microscope) is used to analyze the surface structure of extracted collagen. The sample was coated with gold using Auto fine ion coater (JEOL JFC-1600), and the surface structure was viewed under a scanning electron microscope (JEOL JSM-638OLA) using 20 Kv as the accelerating voltage.
Results and discussion
RSM model of collagen extraction from cuttlefish skin
To find the optimal (highest yield) reaction conditions, a triple-water quasi-factor experimental design (BBD) was conducted to investigate the effect of the target function (yield (Y)) of collagen extraction from cuttlefish skin based on the experimental conditions with pH (A), solid-liquid ratio (B), and pepsin (C). shows a total of 15 runs and their responses using the BBD experiment, where the collagen yield varied between 9.38% and 4.84%. The highest collagen yield was run number 11 (). In summary, the highest yields were obtained for pH 1.5, 20 mL/g (solid-liquid ratio) and 15 U/mg (pepsin) (8.36, 8.64, 9.38%, and average 8.79%). The higher collagen yield (%) means that the designed reaction conditions are favorable for maximum extraction. Therefore, if the coefficients of the respective variables are positive in the regression calculations, it means that they are favorable for collagen extraction. The following equation can be obtained from the complex regression analysis table.
Table 2. Experimental observations of independent variable in Box-Behnken design.
In addition, the ANOVA table () and the analysis table show that the F-value of the regression model is 57.55 and the p-value is 0.0002, which means that the regression model is significant. To check the validity of the regression model, the F-value for lack of fit have calculated as 2.17 with a p-value greater than 0.05, indicating no lack of degree. Therefore, the model is acceptable. In addition, the check coefficient R2 of 0.9905 is very close to 1, which shows that the response surface evolution model can adequately describe the system and indicates that the experimental data are consistent with the regression results. In addition, the predicted R2 of 0.8784 is in reasonable agreement with the adjusted R2 of 0.9733. These results also support the adequacy of this regression model ().
Table 3. ANOVA of response surface quadratic model.
Table 4. Test and analysis of the consistency between test data and regression results.
From the ANOVA table (), it can be seen that there is a significant difference (p < .005) in the solid-liquid ratio (B) in the linear term with the highest F value and the lowest p-value, which represents the most significant effect. In the secondary items, A2, B2, and C2 also reached significant levels (p < .05), indicating that doubling the value would significantly affect the collagen yield. The crossover terms (AB, AC, BC) did not reach significant levels, indicating that the interaction between them was not significant in terms of yield. This quadratic regression equation describes the relationship between the above operating parameters, cuttlefish skin’s collagen yield, and the response surface and contour plots. The effect of solid-liquid ratio (B), which was significant in collagen yield, was investigated together with the effect of pH (A) and pepsin (C) on collagen yield, and the results are shown in .
Figure 1. Contour plots of process variables of collagen extraction variables from cuttlefish. 1A. pH (A) and solvent/solid (B); 1B. pH (A) and pepsin (C); 1C. solvent/solid (B) and pepsin (C).
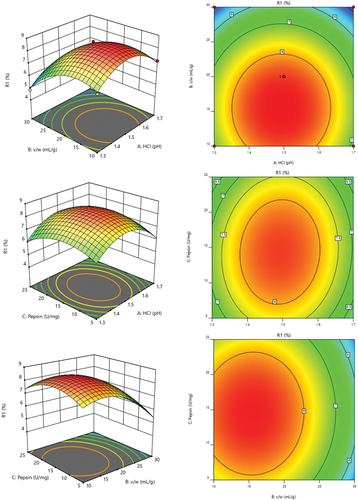
Further analysis of the effect of pH, solid-liquid ratio, and pepsin application on RSM () have performed. The normal plot of residuals (Figure S1A is used to analyze the best visual graph to determine whether the data obeys the normal distribution or not. Therefore, the residual normality graph can be used to see the numerical status of this research to determine the numerical correctness. The numerical performance of Residuals vs. Predicted (Figure S1B) have evaluated. In the Residuals usually contains much unknown information behind the model fitting, if the pattern is correct, the plot should show a random distribution. Box-Cox for power transforms (Figure S1C) is the most common model in the world. Box-cox transformation is a generalized method proposed by Box and Cox in 1964. It is also a common data transformation in statistical modeling, which is usually used in cases where the continuous response variation does not satisfy the normal distribution. Box-cox variation can significantly improve the normality, symmetry, and equality of variance of the data, therefore, Figure S1A shows the Box-cox transformation under the calculation of statistical software. In the part where Cook’s distance values are presented(Figure S1D), after calculating the leverage values of the observations, it is found that those views with larger leverage values should be considered as potential threats to the stability of the model, and then the image of Cook’s distance can be used to measure the impact of an observation on the model. When the leverage value is large and the absolute value of the standardized residuals is also large, the Cook’s distance will be large. When using linear regression, it is common to make a scatter convexity of the Cook distance and the fitted value of each observation or to make a scatter plot of the leverage and the standardized residuals to determine the outliers. In short, the values of the model show an effect of the observations on the model, and it shows that the effect on the yield can be explored by setting the three factors mentioned above.
Charge-to-mass ratio analysis of cuttlefish skin collagen
The protein sequence information was obtained by tandem mass spectrometry. After protein hydrolysis, the sample solution injected into the mass spectrometer to sort the different peptides so that only one selected peptide was analyzed subsequently. Figure S2 shows the initial detection of the matrix blank, and the signal intensity displayed on the Y-axis can confirm the basic status of the analytical equipment. The mass spectrometric analysis of collagen results showed that the main m/z signals were generated in the range of 2000–4000.
SDS PAGE analysis of collagen from cuttlefish skin
Freeze-dried collagen samples were analyzed by electrophoresis, and the result have shown in . The electrophoresis pattern of collagen consists of three protein chains, namely α1, α2, and β chains. On detailed analysis, α1 and α2 chains have a molecular weight of 75–130 KD, while β chains have molecular weights above 200 KD, which are different from other collagen sources .[Citation32] The electrophoretic profile of the collagen is approximately the same as standard type I collagen .[Citation33] In comparison, it has been observed to be similar to the typical type I collagen. The absence of other staining bands on the spectrum shows that the collagen samples extracted in this test were of high purity. In general, collagen obtained from fish living in a cold environment has a lower hydroxyproline content and its thermal stability is lower than that obtained from fish living in a warm environment .[Citation34] The thermal transition or denaturation temperature varies depending on the fish, habitat temperature, where the fish live, season, and age .[Citation35] On the other hand, collagen’s stability is known to depend on the environment and body temperature .[Citation36] Therefore, collagen from S. pharaonis skin has higher thermal stability, related to the collagen from other members in this species.
Figure 2. Electrophoretic comparative study pattern of collagen extracted from the cuttlefish skin. L1- Pre-stained protein marker, L2- Collagen dissolved in water (4 mg/mL).
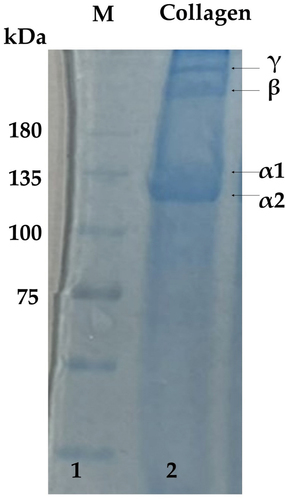
In the present study, the presence of α1, α2, and β chains was recorded by SDS-PAGE. The composition of the skin collagen has found to be similar to previous studies. The inter-and intramolecular cross-linking components found in the collagen of S. pharaonis skin were similar to those of S. maculatus[Citation37] and single-eyed puffer fish skin .[Citation38] The presence of two distinct subunits (α1 and α2) suggests that the major collagen from walleye pollock (Theragra chalcogramma) skin may be type I collagen .[Citation33] This is consistent with the present study, in which molecular weights between 102 and 110 kDa were observed, similar to type I collagen. This study also makes similar observations to type I collagen extracted from aquaponics-raised skin .[Citation39] Type I collagen consists of two identical α chains and one β chain, while type II comprises three identical α chains. Type I collagen is commonly found in higher animals, such as skin, bones, tendons, etc. It is the most abundant and commercially important type of collagen among all types of collagen and is widely used in biomedical, pharmaceutical, food, and cosmetic industries .[Citation40] Since by-products of fish processing cause environmental issues, extraction of collagen from fish skin can reduce the severity of the issue and act as an additional income for the fish industry.[Citation41] It can also increase the diversity of collagen choices to provide consumers with different needs.
Collagen accounts for about 20% of the total mammalian protein and is a very important protein in the human body. It is associated with connective tissue and is composed of 19 different amino acids in a tight triple helix, each chain being over 1400 amino acids long .[Citation42] Among them, glycine and proline or hydroxyproline are the main types of amino acids in the collagen chain.
Since more than 60% of fish processing by-products are waste, these large amounts of fish by-products can cause serious pollution and disposal problems. These fish wastes are rich in proteins, which enzymes can hydrolyze to obtain peptides, which have good nutritional composition, biological activity, and antioxidant activity, and on the other hand, can solve the pollution and disposal problems. Therefore, this project not only solves the waste problem but also maximizes the utilization of cuttlefish by extracting collagen from the cuttlefish skin. The collagen contained in a large number of by-products of aquatic processing is the best source of natural antioxidants, such as Alaskan cod skin (Gadus macrocephalus), longitudinal flute skin, flounder fish skin, cod skin, squid skin, Wuguo fish skin, grouper skin, puffer skin, and river pellet skin. Kristinsson et al. (2000) reported that fish protein hydrolyzate is considered the most important source of protein and bioactive peptides, and the production and functional properties of fish protein hydrolyzate were discussed .[Citation43]
Studies have reported that the ability of collagen to scavenge DPPH radicals may be related to the electrons and protons provided by the amino acids or peptides contained in collagen after hydrolysis and the ability to scavenge DPPH increases with increasing concentration .[Citation44] Je et al. (2008) showed that Pro, Leu, Ala, and Tyr contained in the peptide fragment are the key scavengers of free radicals .[Citation45] In addition, researchers revealed that peptides with aromatic amino acids (His, Met, Cys, Pro, Leu, and Val) are antioxidant peptides with effective free radical scavenging activity .[Citation46] You et al. (2010)[Citation47] showed that the DPPH radicals scavenged by the pepsin hydrolysis for 0.5 hours were 88.2%, which was 9% (80.8%) higher than that without the enzyme, and there was no significant difference during the 2-hour process .[Citation48] It was pointed out that squid skin gelatin hydrolyzate has a higher iron reduction ability than tuna skin and halibut skin hydrolyzate, and different enzyme peptide cutoffs also affect its antioxidant activity. Generally, if peptides rich in hydrophobic amino acids, can inhibit lipids and act as proton donors for peroxyl radicals and chelators for metal ions .[Citation48]
SEM analysis of cuttlefish skin collagen
The raw cuttlefish skin (Figure S3A), freeze-dried collagen (Figure S3B) extracted, and collagen extracted after pepsin/acid homogenization from cuttlefish skin (Figure S3C) have analyzed by SEM. At a magnification of 200x, the collagen extracted from the experiment showed a lamellar fibrous and reticular structure. Therefore, the structural integrity of the collagen surface obtained by this extraction method have confirmed. Although the collagen molecules treated with pepsin became smaller, the reticular fibrous structure was still maintained and not damaged by pepsin. In manufacturing biomedical materials, the small and tightly packed reticular fibrous structure and pores are considered to have very good water retention properties .[Citation49] Electron micrographs (Figure S3C) show random entanglement of the coil-like structures, indicating the fibrillar nature of collagen as reported in the literature .[Citation39,Citation50] At higher magnification, the coil-like protofibrils were found to be interconnected sheets. The space between the interlocking sheets gives the collagen porosity, which will facilitate the incorporation of any value-added chemicals such as drugs for further applications. Therefore, the collagen of cuttlefish skin should have a certain potential for future applications.
Conclusion
Under optimal conditions, the maximum collagen yield of cuttlefish skin was 8.79%. This study showed that the collagen yield was increased at pH 1.5, 20 mL/g (solid-liquid ratio), and 15 U/mg (pepsin). The optimal conditions (pH, solid-liquid ratio and pepsin) for obtaining the highest collagen yield (per gram of fish skin) were determined using Box Behnken Design response surface methodology. Each of the three variables was found to have a significant effect on collagen extraction from cuttlefish skin, and positive correlations were observed between all these variables. The R2 value of the mathematical model obtained was 0.9905, and the p-value was 0.0002, indicating that the predicted values of collagen production from cuttlefish skin were in good agreement with the experimental values, confirming the good generalization of the mathematical model. The collagen extracted from cuttlefish was the type I collagen, which consisted of two α-chains and one β-fold (α2, α1, β). SEM analysis of collagen surface confirmed the presence of collagenous protofibrils in the cuttlefish skin waste.
Supplemental Material
Download ()Acknowledgments
The author would thank all those who volunteered for assisting with the study. The authors declare no conflicts of interest.
Disclosure statement
The authors declare no conflict of interest.
Supplementary material
Supplemental data for this article can be accessed online at https://doi.org/10.1080/10942912.2022.2127762
Additional information
Funding
References
- Dekkers, E.; Raghavan, S.; Kristinsson, H. G.; Marshall, M. R. Oxidative Stability of Mahi Mahi Red Muscle Dipped in Tilapia Protein Hydrolysates. Food Chem. 2011, 124(2), 640–645. DOI: 10.1016/j.foodchem.2010.06.088.
- Lin, L.; Regenstein, J. M.; Lv, S.; Lu, J.; Jiang, S. An Overview of Gelatin Derived from Aquatic Animals: Properties and Modification. Trends Food Sci. Technol. 2017, 68, 102–112. DOI: 10.1016/j.tifs.2017.08.012.
- Hsu, K. C. Purification of Antioxidative Peptides Prepared from Enzymatic Hydrolysates of Tuna Dark Muscle by-product. Food Chem. 2010, 122(1), 42–48. DOI: 10.1016/j.foodchem.2010.02.013.
- Wang, C.-H.; Doan, C. T.; Nguyen, V. B.; Nguyen, A. D.; Wang, S.-L. Reclamation of Fishery Processing Waste: A Mini-Review Molecules. 24(12). 2019, 2234. doi:10.3390/molecules24122234.
- Jridi, M.; Souissi, N.; Mbarek, A.; Chadeyron, G.; Kammoun, M.; Nasri, M. Comparative Study of physico-mechanical and Antioxidant Properties of Edible Gelatin Films from the Skin of Cuttlefish. Int. J. Biol. Macromol. 2013, 61, 17–25. DOI: 10.1016/j.ijbiomac.2013.06.040.
- Jridi, M.; Abdelhedi, O.; Souissi, N.; Kammoun, M.; Nasri, M.; Ayadi, M. A. Improvement of the Physicochemical, Textural and Sensory Properties of Meat Sausage by Edible Cuttlefish Gelatin Addition. Food Biosci. 2015, 12, 67–72. DOI: 10.1016/j.fbio.2015.07.007.
- Souissi, N.; Jridi, M.; Nasri, R.; BenSlama, R.; Njeh, M.; Nasri, M. Effects of the Edible Cuttlefish Gelatin on Textural, Sensorial and Physicochemical Quality of Octopus Sausage. LWT - Food Sci. Technol. 2016, 65, 18–24. DOI: 10.1016/j.lwt.2015.07.051.
- Kchaou, H.; Jridi, M.; Benbettaieb, N.; Debeaufort, F.; Nasri, M. Bioactive Films Based on Cuttlefish (Sepia Officinalis) Skin Gelatin Incorporated with Cuttlefish Protein Hydrolysates: Physicochemical Characterization and Antioxidant Properties. Food Packag. Shelf Life. 2020, 24, 100477. DOI: 10.1016/j.fpsl.2020.100477.
- Kchaou, H.; Jridi, M.; Abdelhedi, O.; Nasreddine, B.; Karbowiak, T.; Nasri, M.; Debeaufort, F. Development and Characterization of Cuttlefish (Sepia Officinalis) Skin gelatin-protein Isolate Blend Films. Int. J. Biol. Macromol. 2017, 105, 1491–1500. DOI: 10.1016/j.ijbiomac.2017.06.056.
- Valdés, A.; Mellinas, A. C.; Ramos, M.; Burgos, N.; Jiménez, A.; Garrigós, M. C. Use of Herbs, Spices and Their Bioactive Compounds in Active Food Packaging. RSC Adv. 2015, 5(50), 40324–40335. DOI: 10.1039/c4ra17286h.
- Hoque, M. S.; Benjakul, S.; Prodpran, T. Properties of Film from Cuttlefish (Sepia Pharaonis) Skin Gelatin Incorporated with Cinnamon, Clove and Star Anise Extracts. Food Hydrocoll. 2011, 25(5), 1085–1097. DOI: 10.1016/j.foodhyd.2010.10.005.
- Bitencourt, C. M.; Fávaro-Trindade, C. S.; Sobral, P. J. A.; Carvalho, R. A. Gelatin-based Films Additivated with Curcuma Ethanol Extract: Antioxidant Activity and Physical Properties of Films. Food Hydrocoll. 2014, 40, 145–152. DOI: 10.1016/j.foodhyd.2014.02.014.
- Tongnuanchan, P.; Benjakul, S.; Prodpran, T. Physico-chemical Properties, Morphology and Antioxidant Activity of Film from Fish Skin Gelatin Incorporated with Root Essential Oils. J. Food Eng. 2013, 117(3), 350–360. DOI: 10.1016/j.jfoodeng.2013.03.005.
- Tongnuanchan, P.; Benjakul, S.; Prodpran, T. Properties and Antioxidant Activity of Fish Skin Gelatin Film Incorporated with Citrus Essential Oils. Food Chem. 2012, 134(3), 1571–1579. DOI: 10.1016/j.foodchem.2012.03.094.
- Jridi, M.; Mora, L.; Souissi, N.; Aristoy, M.-C.; Nasri, M.; Toldrá, F. Effects of Active Gelatin Coated with Henna (L. Inermis) Extract on Beef Meat Quality during Chilled Storage. Food Control. 2018, 84, 238–245. DOI: 10.1016/j.foodcont.2017.07.041.
- Kim, S. K. Marine Cosmeceuticals. J. Cosmet. Dermatol. 2014, 13(1), 56–67. DOI: 10.1111/JOCD.12057.
- Xu, Y. J.; Han, X. L.; Li, Y. Effect of Marine Collagen Peptides on Long Bone Development in Growing Rats. J. Sci. Food Agric. 2010, 90(9), 1485–1491. DOI: 10.1002/JSFA.3972.
- Kim, S. K.; Mendis, E. Bioactive Compounds from Marine Processing Byproducts - A Review. Food Res. Int. 2006, 39(4), 383–393. DOI: 10.1016/J.FOODRES.2005.10.010.
- Swatschek, D.; Schatton, W.; Kellermann, J.; Müller, W. E. G.; Kreuter, J. Marine Sponge Collagen: Isolation, Characterization and Effects on the Skin Parameters surface-pH, Moisture and Sebum. Eur. J. Pharm. Biopharm. 2002, 53(1), 107–113. DOI: 10.1016/S0939-6411(01).
- Jridi, M.; Bardaa, S.; Moalla, D.; Rebaii, T.; Souissi, N.; Sahnoun, Z.; Nasri, M. Microstructure, Rheological and Wound Healing Properties of collagen-based Gel from Cuttlefish Skin. Int. J. Biol. Macromol. 2015, 77, 369–374. DOI: 10.1016/J.IJBIOMAC.2015.03.020.
- Roper, C. F. E.; Sweeney, M. J.; C, N. Cephalopods of the World: An Annotated and Illustrated Catalogue of Species of Interest to Fisheries; United Nations Development Programme; Food and Agricultural Organization of the United Nations, New York, NY, 1984
- NESIS, K. N.; Burgess, L. A. Cephalopods of the World: Squids, Cuttlefishes, Octopuses, and Allies.; T.F.H. Publications: Neptune City: N.J, 1987
- Aewsiri, T.; Benjakul, S.; Visessanguan, W. Functional Properties of Gelatin from Cuttlefish (Sepia Pharaonis) Skin as Affected by Bleaching Using Hydrogen Peroxide. Food Chem. 2009, 115(1), 243–249. DOI: 10.1016/j.foodchem.2008.12.012.
- Li, F.; Luo, P.; Liu, H. A. Potential Adjuvant Agent of Chemotherapy: Sepia Ink Polysaccharides. Mar. Drugs. 2018, 16. DOI: 10.3390/md16040106.
- Shanmugam, A.; Ramasamy, P.; Bharti, K.; Ramachandran, S.; Subhapradha, N.; Vairamani, S.; K, J. Isolation and Characterization of Collagen from the skin of Sepia Pharaonis (Ehrenberg, 1831). Int J Curr Res 2011, 3(6), 107–111.
- Subhan, F.; Hussain, Z.; Tauseef, I.; Shehzad, A.; Wahid, F. A Review on Recent Advances and Applications of Fish Collagen. Crit. Rev. Food Sci. Nutr. 2021, 61(6), 1027–1037. DOI: 10.1080/10408398.2020.1751585.
- Jridi, M.; Nasri, R.; Lassoued, I.; Souissi, N.; Mbarek, A.; Barkia, A.; Nasri, M. Chemical and Biophysical Properties of Gelatins Extracted from alkali-pretreated Skin of Cuttlefish (Sepia Officinalis) Using Pepsin. Food Res. Int. 2013, 54(2), 1680–1687. DOI: 10.1016/j.foodres.2013.09.026.
- Tan, Y.; Chang, S. K. C. Isolation and Characterization of Collagen Extracted from Channel Catfish (Ictalurus Punctatus) Skin. Food Chem. 2018, 242, 147–155. DOI: 10.1016/J.FOODCHEM.2017.09.013.
- Arumugam, G. K. S.; Sharma, D.; Balakrishnan, R. M.; Ettiyappan, J. B. P. Extraction, Optimization and Characterization of Collagen from Sole Fish Skin. Sustain Chem Pharm. 2018, 9, 19–26. DOI: 10.1016/J.SCP.2018.04.003.
- Kiew, P. L.; Don, M. M. Modified Lowry’s Method for Acid and Pepsin Soluble Collagen Measurement from Clarias Species Muscles. Open Access Sci Rep. 2013, 2, 1–5.
- Jinal, H. N.; Sakthivel, K.; Amaresan, N. Characterisation of Antagonistic Bacillus Paralicheniformis (Strain EAL) by LC–MS, Antimicrobial Peptide Genes, and ISR Determinants. Antonie Van Leeuwenhoek. 2020, 113(8), 1167–1177. DOI: 10.1007/S10482-020-01423-4.
- Krishnamoorthi, J.; Ramasamy, P.; Shanmugam, V.; Shanmugam, A. Isolation and Partial Characterization of Collagen from Outer Skin of Sepia Pharaonis (Ehrenberg, 1831) from Puducherry Coast. Biochem. Biophys. Rep. 2017, 10, 39–45. DOI: 10.1016/J.BBREP.2017.02.006.
- Yan, M.; Li, B.; Zhao, X.; Ren, G.; Zhuang, Y.; Hou, H.; Zhang, X.; Chen, L.; Fan, Y. Characterization of acid-soluble Collagen from the Skin of Walleye Pollock (Theragra Chalcogramma). Food Chem. 2008, 107(4), 1581–1586. DOI: 10.1016/J.FOODCHEM.2007.10.027.
- Muyonga, J. H.; Cole, C. G. B.; Duodu, K. G. Characterisation of Acid Soluble Collagen from Skins of Young and Adult Nile Perch (Lates Niloticus). Food Chem. 2004, 85(1), 81–89. DOI: 10.1016/J.FOODCHEM.2003.06.006.
- Duan, R.; Zhang, J.; Du, X.; Yao, X.; Konno, K. Properties of Collagen from Skin, Scale and Bone of Carp (Cyprinus Carpio). Food Chem. 2009, 112(3), 702–706. DOI: 10.1016/J.FOODCHEM.2008.06.020.
- Rigby, B. J. Amino-acid Composition and Thermal Stability of the Skin Collagen of the Antarctic Ice-fish. Nat. 1968, 219(5150), 166–167. DOI: 10.1038/219166a0.
- Kittiphattanabawon, P.; Benjakul, S.; Visessanguan, W.; Kishimura, H.; Shahidi, F. Isolation and Characterisation of Collagen from the Skin of Brownbanded Bamboo Shark (Chiloscyllium Punctatum). Food Chem. 2010, 119(4), 1519–1526. DOI: 10.1016/J.FOODCHEM.2009.09.037.
- Nagai, T.; Araki, Y.; Suzuki, N. Collagen of the Skin of Ocellate Puffer Fish (Takifugu Rubripes). Food Chem. 2002, 78(2), 173–177. DOI: 10.1016/S0308-8146(01)00396-X.
- Wang, L.; Liang, Q.; Wang, Z.; Xu, J.; Liu, Y.; Ma, H. Preparation and Characterisation of Type I and V Collagens from the Skin of Amur Sturgeon (Acipenser Schrenckii). Food Chem. 2014, 148, 410–414. DOI: 10.1016/J.FOODCHEM.2013.10.074.
- Liu, D.; Liang, L.; Regenstein, J. M.; Zhou, P. Extraction and Characterisation of pepsin-solubilised Collagen from Fins, Scales, Skins, Bones and Swim Bladders of Bighead Carp (Hypophthalmichthys Nobilis). Food Chem. 2012, 133(4), 1441–1448. DOI: 10.1016/J.FOODCHEM.2012.02.032.
- Kittiphattanabawon, P.; Benjakul, S.; Visessanguan, W.; Nagai, T.; Tanaka, M. Characterisation of acid-soluble Collagen from Skin and Bone of Bigeye Snapper (Priacanthus Tayenus). Food Chem. 2005, 89, 363–372. DOI: 10.1016/J.FOODCHEM.2004.02.042.
- Goodsell, D.; Collagen Available online: https://pdb101.rcsb.org/motm/4 (accessed on Feb 10, 2022).
- Kristinsson, H. G.; Rasco, B. A. Fish Protein Hydrolysates: Production, Biochemical, and Functional Properties. Crit. Rev. Food Sci. Nutr. 2000, 40, 43–81. DOI: 10.1080/10408690091189266.
- Yang, J. I.; Ho, H. Y.; Chu, Y. J.; Chow, C. J. Characteristic and Antioxidant Activity of Retorted Gelatin Hydrolysates from Cobia (Rachycentron Canadum) Skin. Food Chem. 2008, 110(1), 128–136. DOI: 10.1016/J.FOODCHEM.2008.01.072.
- Je, J. Y.; Qian, Z. J.; Lee, S. H.; Byun, H. G.; Kim, S. K. Purification and Antioxidant Properties of Bigeye Tuna (Thunnus Obesus) Dark Muscle Peptide on Free Radical-Mediated Oxidative Systems. J. Med. Food. 2008, 11(4), 629–637. DOI: 10.1089/JMF.2007.0114.
- Mendis, E.; Rajapakse, N.; Kim, S. K. Antioxidant Properties of a Radical-Scavenging Peptide Purified from Enzymatically Prepared Fish Skin Gelatin Hydrolysate. J. Agric. Food Chem. 2004, 53(3), 581–587. DOI: 10.1021/JF048877V.
- You, L.; Zhao, M.; Regenstein, J. M.; Ren, J. Changes in the Antioxidant Activity of Loach (Misgurnus Anguillicaudatus) Protein Hydrolysates during a Simulated Gastrointestinal Digestion. Food Chem. 2010, 120(3), 810–816. DOI: 10.1016/J.FOODCHEM.2009.11.018.
- Alemán, A.; Giménez, B.; Pérez-Santin, E.; Gómez-Guillén, M. C.; Montero, P. Contribution of Leu and Hyp Residues to Antioxidant and ACE-inhibitory Activities of Peptide Sequences Isolated from Squid Gelatin Hydrolysate. Food Chem. 2011, 125(2), 334–341. DOI: 10.1016/J.FOODCHEM.2010.08.058.
- Tamilmozhi, S.; Veeruraj, A.; Arumugam, M. Isolation and Characterization of Acid and pepsin-solubilized Collagen from the Skin of Sailfish (Istiophorus Platypterus). Food Res. Int. 2013, 54(2), 1499–1505. DOI: 10.1016/J.FOODRES.2013.10.002.
- Sankar, S.; Sekar, S.; Mohan, R.; Rani, S.; Sundaraseelan, J.; Sastry, T. P. Preparation and Partial Characterization of Collagen Sheet from Fish (Lates Calcarifer) Scales. Int. J. Biol. Macromol. 2008, 42(1), 6–9. DOI: 10.1016/J.IJBIOMAC.2007.08.003.