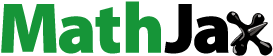
ABSTRACT
This study aimed to investigate the effects of salted shrimp on kimchi fermentation. Four types of kimchi (KWD, KWR, KWC, and KWY), based on the variety of salted shrimp (Detteugijeot, Red shrimp jeot, Chujeot, and Yukjeot, respectively), were prepared and stored at 4°C for 6 weeks. Amino nitrogen content in KWD was significantly higher after 4 weeks (P < .05). Arginine content decreased during the fermentation process. Red shrimp had the highest hypoxanthine content among the salted shrimp. However, there was no difference in kimchi hypoxanthine levels based upon the variety of shrimp. Hypoxanthine levels tended to increase during the fermentation process. The main metabolites were converted from sugars to amino acids as the fermentation progressed. The distribution of the microbial community differed with the variety of salted shrimp. In the sixth week, KWY was associated with an umami taste, possibly because the hypoxanthine content increased the most in KWY.
Introduction
Kimchi is a representative fermented food in Korea. It is made with cabbage or radish as the major ingredient and numerous spices such as garlic, ginger, red pepper powder, and jeotgal are added.[Citation1] Among these ingredients, jeotgal is a unique Korean fermented seafood product made from the whole meat or internal organs of fish and shellfish, fermented and salted for inhibition of decay, autolysis, and decomposition of the main ingredients by microbial activities.[Citation2] Salted shrimp (saeujeot) is the most commonly used jeotgal for making kimchi.[Citation3] According to the seasons, Saeujeot made from kuruma shrimp (Marsupenaeus japonicus) caught in the spring is called Detteugijeot, and Saeujeot made from red shrimp caught in summer is called Red shrimp jeot. Salted fish made from shrimp caught in June are called Yukjeot, and salted fish made from shrimp caught in autumn are called Chujeot.[Citation4,Citation5]
Sauces using seafood, such as jeotgal contain a variety of endogenous enzymes derived from the muscles and digestive tracts of raw sea animals.[Citation6] These enzymes promote fermentation even under high salinity conditions and increase the production of amino acids.[Citation7–9] Therefore, jeotgal has a high content of amino acids, which serves as a source of amino acids and imparts flavors such as umami.[Citation10] It was also found that jeotgal promotes the growth of lactic acid bacteria (LAB) essential for kimchi fermentation and produces functional substances such as gamma-aminobutyric acid (GABA) and mannitol.[Citation11] However, there are limited studies on the effects of specific jeotgal on kimchi quality and changes in ingredients during fermentation.
In previous studies, the effects of temperature, salinity, and nitrite on the preparation of salted seafood,[Citation12–14] quality characteristics of salted shrimp,[Citation15–17] biogenic amine content,[Citation18,Citation19] determination of toxic elements,[Citation20] and protein degradation properties[Citation7,Citation8] were examined. Notably, as a functional study, Peralta et al. (2008) described an increase in the free amino acid content, antioxidant effect, and changes in polyunsaturated fatty acids (PUFAs) of salted shrimp.[Citation21] In addition, as studies related to jeotgal and kimchi, research on the effect of salted fish when added to kimchi as a minor ingredient,[Citation1,Citation22–25] the effect of salted fish on the nitrite decomposition of kimchi,[Citation26] and the organic acid and nucleic acid content[Citation27] have been conducted. However, these studies only compared jeotgal and other ingredients, or each jeotgal was made from different raw materials, and the comparison within the salted shrimp varieties was insufficient.
The major objective of this study was to investigate the effects of salted shrimp (saeujeot) on kimchi fermentation. The correlation between microorganisms and metabolites and the effects of amino acids and nucleic acids on sensory evaluation could organically explain the effects of salted shrimp of four different varieties (Detteugijeot, Red shrimp jeot, Chujeot, and Yukjeot) on kimchi fermentation (KWD, KWR, KWC, and KWY, respectively). This is an important basis for an overall understanding of kimchi fermentation, changes in its components, and sensory properties.
Materials and methods
Experimental materials
Kimchi ingredients such as cabbage, garlic, ginger, and red pepper powders were purchased from Western Agricultural and Fishery Market in Gwangju (Republic of Korea). Salted shrimp of different varieties was obtained from Shinan Saeujeot (Shinan-gun, Republic of Korea) and fermented for 5 months at 5°C. The general features and characteristics of the salted shrimp are listed in . All the experimental analyses were performed using first-grade analytical reagents obtained from Daejung (Gyeonggi-do, Republic of Korea). Water and acetonitrile used for high-pressure liquid chromatography (HPLC) were of chromatographic grade (Merck, NJ, USA). The standard chemicals for analysis of amino acid and nucleotide (including hypoxanthine and inosine) were obtained from Agilent Technologies (Pal Alto, CA, USA) and Sigma-Aldrich (St. Louis, MO. USA), respectively.
Table 1. General features and characteristics of salted shrimp used to make kimchi.
Kimchi preparation
The kimchi cabbage was soaked in a 10% (w/v) pure salt solution for 18 h. The salted kimchi cabbage was then washed three times with water and drained for 2 h. The salted kimchi cabbage was cut into 3 × 3 cm pieces to prepare kimchi. The kimchi seasoning was prepared by mixing 16.7% garlic, 2.7% ginger, 23.3% red pepper powder, 20.0% salted shrimp, and 37.3% water. This seasoning was added to the salted kimchi cabbage at a ratio of 85:15 (salted cabbage: seasoning). The resultant test groups were labeled KWD (kimchi with Detteugijeot), KWR (kimchi with Red shrimp jeot), KWC (kimchi with Chujeot), and KWY (kimchi with Yukjeot). Each kimchi sample (500 g) was separately packed in a polyethylene (PE) film and sealed using a vacuum packaging machine (AZC-070, INTRISE, Ansan, Korea). The packed samples were stored at 4°C for 6 weeks, and their characteristics were analyzed at weekly intervals.
Analysis of pH and titratable acidity
Each sample was ground in a blender, and the extracted juice was used to measure pH and acidity. The pH and acidity values were measured using a TitroLine 5000 (SI Analytics GmbH, Mainz, Germany) at 24–26°C. The total acidity was titrated with 0.1 M NaOH until the endpoint pH of 8.3 was reached. Titratable acidity was calculated as the percentage of lactic acid produced
Analysis of amino nitrogen
The amino nitrogen (AN) content of the samples was measured using the formol method.[Citation28] Each 2 g sample was suspended in distilled water to make a final volume of 100 mL and then sonicated for 30 min. Twenty milliliters of sample extract was added to 20 mL of neutral formalin solution, mixed, and then titrated to a pH of 8.3 with 0.1 M NaOH. AN content was calculated using the following equation:
where A is the titrated volume of 0.1 M NaOH consumed by the sample (mL), B is the titrated volume of 0.1 M NaOH consumed by the blank reagent (mL), 1.4 is the AN content corresponding to milliliters of 0.1 M NaOH, and D is the dilution magnification.
Microbial analysis
Kimchi samples ground with a sterilized blender were aseptically transferred into a sterile filter bag and then squeezed out. The solutions were serially decimally diluted with 0.85% saline and then inoculated in triplicate on Coliform Count plates (Petrifilm™ CC plates, 3 M™, Seoul, Korea) for fecal coliforms, Aerobic Count plates (Petrifilm™ AC plates) for aerobic plate counts, and Lactic Acid Bacteria Count plates (Petrifilm™ LAB plates) for lactic acid bacteria (LAB). The CC plates were incubated at 35°C for 24 h, and the AC and LAB plates were incubated at 35°C for 48 h. Each microbial count was acquired from the mean of three measurements and expressed as log CFU/g.
Analysis of free amino acids and nucleotides
For free amino acid and nucleotide analyses, kimchi samples were homogenized and diluted. The filtered solutions were used as samples for further analysis. Free amino acids and nucleotides were analyzed using HPLC (Ultimate 3000, Thermo Dionex, USA) equipped with an Inno C18 column (4.6 × 150 mm, 5um particle size, Youngjin Biochro, Korea) and an Inno C18 column (4.6 × 250 mm, 5um particle size, Youngjin Biochro, Korea), respectively. The HPLC operational conditions for the free amino acids were as follows: mobile phase A, 40 mM sodium phosphate; and mobile phase B, 10:45:45 (v/v%) water, acetonitrile, and methanol. For nucleotides, mobile phase A was 0.05 M potassium phosphate, and mobile phase B was 90:10 (v/v%) potassium phosphate and methanol. The flow rates were 1.5 mL/min and 0.7 mL/min, respectively. The oven temperature was set at 40°C.
Microbial community analysis
Total DNA was extracted from the samples using a PowerSoil DNA Isolation Kit (Mo Bio Laboratories, Carlsbad, CA, USA), according to the manufacturer’s instructions. DNA concentration and purity were measured using a NanoDrop ND-2000 spectrophotometer (Thermo Fisher Scientific, Waltham, MA, USA). PCR was performed using the following primers: 16S V3 (5’-TCG TCG GCA GCG TCA GAT GTG TAT AAG AGA CAG CCT ACG GGN GGC WGC AG-3) and 16S V4 (5´-GTC TCG TGG GCT CGG AGA TGT GTA TAA GAG ACA GGA CTA CHV GGG TAT CTA ATC C-3). The PCR cycle was as follows: initial denaturation for 2 min at 95°C, followed by 30 cycles of denaturation for 20s at 95°C, annealing for 15s at 72°C, extension for 1 min at 72°C, and a final extension step for 5 min.
The total DNA extracted from kimchi samples was subjected to PCR using the 16S V4 primer. Sequencing was conducted on the Mi-SeqTM platform (Illumina, San Diego, CA, USA) by Macrogen (Macrogen Inc., Seoul, Korea). After sequencing errors and ambiguous and chimeric sequences were eliminated, the CD-HIT-OTU analysis program was used to determine the species-level operational taxonomic units (OTUs) to cluster sequences with a similarity of 97%. The representative OTU sequence was used to perform UCLUST (v.1.2.22) in the reference database (SILVA DB) and generate taxonomic assignments based on homology. Microbial communities were analyzed using classifiers from the Ribosomal Database Project in QIIME (v.1.8.0).
Analysis of metabolome analysis
After freeze-drying, 100 μL of O-methoxyamine hydrochloride in a pyridine solution (20 mg/mL) was added to each sample. All samples were incubated at 30°C for 90 min in the dark. The silylation process was performed by adding 50 μL of N-methyl-N-trimethylsilyl-trifluoroacetamide containing 1% trimethylchlorosilane. After vortexing each sample for 30s, it was incubated at 37°C for 30 min. Ten microliters of ribitol (0.5 mg/L) was added as the internal standard (IS). After centrifuging the samples at 13,000 rpm for 10 min, the supernatants were subjected to GC-MS analysis. The derivatized samples were analyzed using GC-MS (QP2020, Shimadzu, Kyoto, Japan). An Rtx-5 MS with a fused silica capillary column (30 m × 0.25 mm ID, J&W Scientific, CA) was used for the separation of metabolites. The front inlet temperature was 230°C. The column temperature was held at 80°C for 2 min isothermally and then raised by 15°C/min to 330°C and held there for 6 min isothermally. The transfer line and ion source temperatures were 250°C and 200°C, respectively. Ionization was achieved with a 70 eV electron beam. The flow rate of helium gas through the column was 1 mL/min. Twenty scans per second were recorded over the mass range of 85–500 m/z. Chromatograms and mass spectra were acquired using Shimadzu GC solution (Shimadzu, Kyoto, Japan). Metabolites were identified by comparing their mass spectra with the AIoutput software, NIST 14.0 library, and the human metabolome database (HMDB, http://www.hmdb.ca).
Sensory evaluation
Sensory evaluation of the four kimchi samples with specific salted shrimps was conducted by 15 trained panelists from the World Institute of Kimchi (Gwangju, Korea). Sensory tests were performed weekly for 6 weeks to compare differences. Four samples were randomly presented to the panelists in clear plastic cups with a random number. Water and crackers were provided to the panel to rinse their palates between samples. Sensory evaluation (sour flavor, off-flavor, sourness, umami, off-taste, and crispy) was scored using a 9-point scale, where 1 = very weak, 3 = weak, 5 = moderate, 7 = strong, and 9 = very strong. Sensory preference (flavor, appearance, taste, texture, and overall likability) was scored using a 9-point scale, where 1 = extremely dislike, 3 = dislike, 5 = neither like nor dislike, 7 = like, and 9 = like extremely.
Statistical analysis
Statistical analyses were performed using GraphPad Prism 9.0 (GraphPad Software, Inc., San Diego, CA, USA). The SPSS software package (Statistical Package for the Social Science, version 19, SPSS Inc., Chicago, IL, USA) was used to perform a one-way ANOVA test for the determination of statistical significance, while Duncan’s multiple range test[Citation29] was performed for post hoc testing to test for significant inter-sample differences (P < 0.05). For correlation analyses between the bacterial community and the metabolites in kimchi, the Pearson’s correlation coefficient, using R statistical system 4.1.3 (R Development Core Team 2022), was performed based on the bacterial abundance in kimchi at the species level and the targeted metabolite concentration. Principal component analysis (PCA) of amino acids and nucleotides with the sensory data as supplementary quantitative variables was performed using the R statistical system 4.1.3 (R Development Core Team 2022) with FactoMineR packages.
Results and discussion
Changes in pH, titratable acidity, and viable bacteria during fermentation
shows the characteristics of salted shrimp fermented for 5 months before being added to kimchi. The pH was approximately 6.74–6.93, and the salinity was approximately 22.37–23.93%. The total nitrogen (TN) was measured in the range of approximately 12.63–14.47 mg/L. Among the nucleic acid-related substances, the amounts of ATP and hypoxanthine in KWR were significantly higher, and the amount of inosine was significantly lower. In general, the amount of hypoxanthine increases and ATP, ADP, AMP, and IMP decrease during the fermentation process of aquatic products.[Citation30,Citation31]
The pH, titratable acidity, and viable bacterial changes in kimchi with specific varieties of salted shrimp were analyzed for 6 weeks (). The initial pH of all the samples was approximately 6.1. There was no difference in pH between the samples, but the pH generally decreased as fermentation progressed. For the first week of the fermentation process, the pH was similar to that of the initial fermentation. However, it decreased sharply in the second week. After the fourth week, the pH of the KWR was slightly higher, but the pH for all samples was in the range of approximately 4.1–4.4. The initial titratable acidity was 0.3% and gradually increased to approximately 1.0%. As with pH, it changed rapidly in the second week, and from the third week, the acidity was slightly lower in the KWR group than in the other groups.
Figure 1. Changes in the pH (a), Acidity (b), AN (c), and microbial evaluation (d-f) of kimchi with different salted shrimp during fermentation.
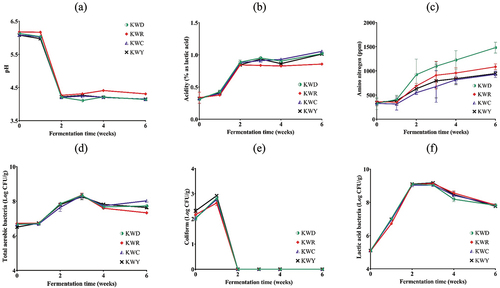
This slightly lower acidity could be related to the growth of LAB at approximately 9.0 log CFU/g. In addition to lactic acid and acetic acid as the main organic acids, minor components such as propionic acid, butanoic acid, 2-methyl propionic acid, fumaric acid, succinic acid, and tartaric acid are produced by LAB during the fermentation of kimchi.[Citation32,Citation33] These organic acids lowered the pH and increased the acidity.[Citation34] As the pH decreased, no coliform bacteria were detected in the second week because, in contrast to LAB, coliform bacteria are difficult to grow below a pH of 4.5.[Citation35] AC and LAB showed maximum growth densities in the range of approximately 8.27–8.35 log CFU/g and 9.04–9.17 log CFU/g, respectively, at week 3, and decreased from week 4. There was no significant difference between each treatment.
Changes in amino nitrogen during fermentation
Amino nitrogen is a chemical form of nitrogen that exists as a free amino group. Its presence means that the protein in the sample is decomposed into amino acids via a small molecule peptide by a microorganism or a self-degrading enzyme.[Citation36] It is used as a food quality index. For example, a fish sauce could be graded by some concentration of α-AN, and the higher the α-AN concentration in the sauce, the better its quality.[Citation37] The changes in AN content during fermentation for the selected kimchi varieties are shown in . In all samples, AN was initially in the range of approximately 326.7–361.7 ppm and gradually increased to approximately 928.3–1481.7 ppm. The tendency for AN to increase in all samples as kimchi fermented was consistent with previous studies.[Citation12,Citation13] There was no significant difference between the samples until the third week. However, from the fourth week, the AN content of the KWD group was significantly higher than that of the other groups. The change in AN in salted fish indicates that the protein is being decomposed into low-molecular-weight peptides and amino acids by microorganisms or proteolytic enzymes.[Citation38] Therefore, it was presumed that kimchi using Detteugijeot was more prone to protein degradation. In a previous study,[Citation39] AN content increased significantly according to the TN content of the raw material. Here, it was further confirmed that when the TN content was similar, the increase in AN could also be affected by the type of salted shrimp.
Microbial community analysis
The taxonomic structures at the species level were investigated to compare the changes in microbial community composition according to the kimchi samples (). The microbial communities of the four kimchi samples showed a similar trend, with Trichocoleus caatingensis being the predominant microorganism at the species level at the beginning of fermentation. However, the relative proportion of Trichocoleus caatingensis in KWD decreased sharply from 58.75% to 25.95% during the first week. At weeks 0 and 1, Bacillus sp. were detected in all kimchi samples, accounting for 8.32, 5.16, 7.10, and 4.60% of the KWD, KWR, KWC, and KWY microbial communities, respectively. B. subtilis and B. velezensis are frequently isolated from a variety of niches, including fermented foods, water, and soil, and produce an antibiotic protein, an alpha-amylase, a protease, and a restriction enzyme.[Citation40] As with this study, Bacillus sp. has been detected in kimchi,[Citation41,Citation42] and it was inferred that it was affected by red pepper powder.[Citation40,Citation43]
Figure 2. Composition of bacterial community at the species level in kimchi fermented with specific varieties of salted shrimp, as found using the SILVA rRNA database. ‘Other’ shows a percentage of reads < 0.5% of the total reads in all kimchi samples in species-level analyses.
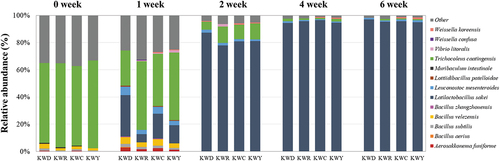
In addition, it was remarkable that Vibrio sp., a marine-derived microorganism, was observed in KWR, KWC, and KWY during the first week and survived until the second week only in KWR. In a previous study,[Citation44] Vibrio parahaemolyticus was detected in 4.3% of salted seafood. Jung et al. (2013) also discovered Vibrio in salted shrimp.[Citation45] Therefore, Vibrio sp. was derived from red shrimp and detected in kimchi in this study. In addition, it could be attributed to the species-specific structure of the red shrimp which is larger and more complex than that of other shrimp. Nevertheless, it was not detected after the second week, because the LAB isolated from salted and fermented shrimp inhibited the growth of Vibrio sp.[Citation46]
The predominance of Latilactobacillus sakei was observed as fermentation progressed. Because Latilactobacillus has acid tolerance, it becomes more dominant as the kimchi fermentation environment changes to more anaerobic and acidic conditions.[Citation47] This was consistent with previous studies[Citation32,Citation41] that studied the microbial community during kimchi fermentation. Latilactobacillus sakei accounted for more than 30% of the total microorganisms within the first week in KWD. After week 4, the proportions of microbial communities remained similar for each sample. Weissella koreensis was not identified in KWR, and Aerosakkonema funiforme was not detected in KWR or KWY over all periods.
The difference in the microbial community was considered due to the difference in the variety of salted shrimp added to kimchi. In previous studies, microbial community of the shrimp ecosystem changes depends on environmental factors such as salinity and temperature.[Citation48] In addition, differences in microbial communities have a significant impact on metabolites during fermentation.[Citation49] Therefore, the changes in the microbial community according to the variety of salted shrimp also affected the metabolites they produced. This suggests that the variety of salted shrimp added to kimchi has the potential to affect the taste and quality of kimchi.
Free amino acids, nucleotides, and their association with the sensory evaluation
In this study, 25 free amino acids and three nucleotides were detected. The free amino acid content of kimchi containing specific varieties of salted shrimp is shown in (except for some amino acids with low content). In all samples, the free amino acid content, such as glutamic acid, alanine, histidine, serine, and GABA, tended to increase but the arginine content decreased. The content of glutamine accounted for approximately 24–33% of the total free amino acids. From the second week, the proline content was significantly higher in KWY and showed a continuous increase. The asparagine content of KWY was the lowest but tended to continue to increase. The main cause of the change in free amino acids during kimchi fermentation is the proteolytic reaction of the protein in jeotgal, which affects the flavor of kimchi.[Citation50]
Table 2. Changes in the free amino acid contents of kimchi with specific salted shrimp during fermentation.
The nucleotide content of kimchi containing saeujeot is shown in . Among the saeujeot, Red shrimp had the highest adenosine triphosphate (ATP, 478.62 ± 14.22 mg/L) and hypoxanthine (HP, 347.07 ± 16.34 mg/L) content and the least amount of inosine (723.44 ± 9.84 mg/L; ). However, when it was added to kimchi, ATP was detected in none of the samples (no data presented), and only HP and inosine were derived from kimchi. Notably, the HP content of KWY increased the most from the lowest 31.16 ± 0.67 mg/L to the highest 99.26 ± 0.32 mg/L compared with other groups. In contrast, no inosine was detected during the second week. ATP and nucleic acid-related substances are degraded by autodigestive enzymes in fish and shellfish muscle,[Citation51] according to the following steps: ADP AMP
IMP
Inosine
Hypoxanthine
Xanthine
Uric acid. Inosine and hypoxanthine are known as bitter-tasting substances.[Citation52] Therefore, the sharp increase of HP content in KWY was due to the high content of autolytic enzymes in yukjeot. This means that KWY had a higher probability of causing a bitter taste compared to other samples.
Table 3. Changes in the nucleic acid contents of kimchi with specific salted shrimp during fermentation.
The sensory evaluation results are shown in . There was a significant difference in sour flavor and sourness in week 0 and the 6 weeks. This result was considered because of the decrease in pH and the increase in content of organic acids such as lactic acid, as fermentation proceeded. It has been studied that sour taste is affected by lactic acid, Late lactobacillus sakei, and Lactobacillus plantarum.[Citation53] The overall preference was significantly higher in KWY at week 4. The KWY was the highest in both flavor and taste, but the difference was not statistically significant. It seems that the high score for acceptability is due to significantly higher glutamine, glutamic acid, alanine, and hypoxanthine in KWY.
Table 4. Sensory evaluation of kimchi with specific salted shrimp during fermentation.
Amino acids such as glutamic acid, glycine, and alanine added a unique flavor to the kimchi, and leucine, isoleucine, and arginine contributed to the bitter taste.[Citation3] As shown in , the relationships among the descriptive analysis-rated attribute intensities in 16 kimchi samples were determined using PCA. Principal components (PCs) 1 and 2 were 67.63% and 14.62%, respectively, which could explain 82.25% of the total fluctuation. Glutamic acid, serine, HP, histidine, and GABA showed strong positive correlations with PC1. These properties are related to kimchi fermentation. In contrast, inosine and arginine, which were associated with the week 0 group where no fermentation occurred, had strong correlations with each other in the negative direction of PC1. Therefore, PC1 can be interpreted as representing the variance between fermentation periods. Arginine, taurine, and glutamine, which were loaded in a positive direction, marked PC2. In addition, as shown in ), umami taste was strongly related to HP and amino acids such as GABA, glutamic acid, and serine. Referring to the supplementary variable, KWY at weeks 4 and 6 was strongly associated with umami taste, which was also related to the increase in HP. Also, this could explain why KWY had the highest overall preference at week 4. In addition, it is considered that strong off-taste and off-flavor were noted in KWR at weeks 4 and 6.
Correlation of microbial community and metabolites
Metabolite profiles are affected by the microbial community composition during kimchi fermentation.[Citation54] shows the Pearson’s correlation coefficient between the top 30 metabolites and the microbial community. The correlations between species-level transitions and changes in the metabolite profile during kimchi fermentation were analyzed using a heatmap. A correlation coefficient analysis was performed using the relative abundance of abundant bacterial taxa (>0.5% of the mean abundance) at the species level and the abundance profiles of kimchi metabolites. The correlation was near 1 in the case of a positive correlation and near in the case of a negative correlation. The metabolites of kimchi with salted shrimp of different varieties were similar (data not shown). In the early stage of fermentation, sugars such as mannose and fructose accounted for a large proportion, and the content of amines, amino acids, and organic acids increased toward the latter stage of fermentation. The early dominant species, Trichocoleus caatingensis, showed a strong positive correlation with sugars such as mannose, fructose, and ribose, and with inosine, fumaric acid, and citric acid. In addition, there was a strong negative correlation with metabolites derived from later fermentation periods, such as hypoxanthine, amino acids, and lactic acid. In contrast, Latilactobacillus sakei, the late dominant species, was positively correlated with the amounts of phosphate, hypoxanthine, amino acids, lactic acid, and propylene glycol in all samples. Notably, Weissella koreensis showed a strong positive correlation with KWC and KWY, but it was weak with KWD and was not found in KWR. W. koreensis showed a positive correlation with HP, lactic acid, or ornithine. Therefore, it was possible that these differences had an effect on enhancing the umami taste and sourness or function of KWC and KWY. Also, in KWY and KWC, which showed overall high preference for taste and flavor, the values of glutamic acid, mannitol, and lactic acid, which can have a significant effect on taste, showed a high correlation with Latilactobacillus sakei, Leuconostoc mesenteroides, and Weissella koreensis. Therefore, it is thought that the microbial community formed by the bacteria derived from Chujeot and Yukueot had a direct effect on the production of metabolites that affect overall preference.
Figure 4. Heat map analyzing the correlation between the species-level microbial community and the top 30 metabolites. The blue and red colors correspond to negative and positive correlations, respectively. * P < .05, ** P < .01, *** P < .001.
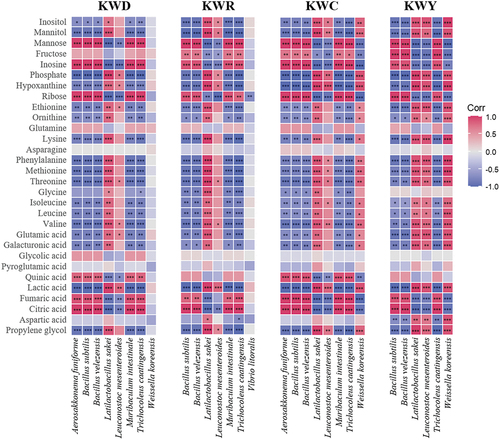
In addition, Leuconostoc mesenteroides and Late lactobacillus sakei showed a high positive correlation with metabolites such as amino acids, lactic acid, and mannitol, which affected the taste of kimchi during late fermentation. This tendency was also observed in previous studies, which reported that different types of preferred free sugars were produced depending on the dominant LAB species, thus producing different metabolites.[Citation55,Citation56] In addition, Jung et al. (2022) reported that changes in the microbial community in kimchi affect metabolites such as ribose, ornithine, and glyceric acid.[Citation57] Therefore, it was thought that the difference in the microbial community between the varieties of salted shrimp caused differences in metabolites during fermentation.
Conclusion
In this study, changes in characteristics were observed depending on the variety of salted shrimp used as kimchi ingredients. The AN content significantly increased in KWD. In the initial stage of fermentation, there was a correlation between Trichocoleus caatingensis and saccharides, inosine, and citric acid. In the late stage of fermentation, there was a correlation between Latilactobacillus sakei and amino acids. In addition, differences in metabolites owing to differences in microbial communities were observed according to the variety of salted shrimp. The highest rate of HP increase was observed in KWY, due to the high autolysis enzyme content. It was also confirmed to have a strong correlation with the umami taste. The quality characteristics and their correlation depending on the type of salted shrimp used for kimchi can help us understand the organic relationship between kimchi fermentation and the manufacture of high-quality kimchi. The results obtained in this study can be applied to fermented foods processed with distinct types of salted shrimp.
ABBREVIATION
Author contributions
Ju-Young Lim: Data curation, Formal analysis, Writing-original draft; Yun-Jeong Choi: Validation, Formal analysis; Seong Youl Lee: Data curation, Validation; Min-Jung Lee: Formal analysis; Hae-Il Yang: Formal analysis, Visualization; Eun-Hae Kim: Formal analysis; Sung Jin Park: Investigation, software; Ji-Hee Yang: Validation, Methodology; Young Bae Chung: Visualization; Sung-Hee Park: Investigation, Methodology; Sung Gi Min: Conceptualization, Project administration; Mi-Ai Lee: Conceptualization, Writing-review and editing, Supervision
Disclosure statement
No potential conflict of interest was reported by the author(s).
Additional information
Funding
References
- Kim, K.-O.; Kim, W.-H. Changes in Properties of Kimchi Prepared with Different Kinds and Levels of Salted and Fermented Seafoods during Fermentation. Korean J. Food Sci. Technol. 1994, 26, 324–330. DOI: 10.3746/jkfn.2005.34.2.267.
- Lee, S.; Lee, H.; Kang, K. Studies on the Origin and Nutrition of Jeotgal (Fermented Fish Products). Anseong Nat Polytech Univ Rep. 1993, 25, 175–195.
- Koo, O. K.; Lee, S. J.; Chung, K. R.; Jang, D. J.; Yang, H. J.; Kwon, D. Y. Korean Traditional Fermented Fish Products: Jeotgal. J. Ethnic Foods. 2016, 3(2), 107–116. DOI: 10.1016/j.jef.2016.06.004.
- Nam, E.-J.; Oh, S.-W.; Jo, J.-H.; Kim, Y.-M.; Yang, C.-B. Purification and Characterization of Alkaline Protease from saewoo-jeot, Salted and Fermented Shrimp (Acetes Japonicus). Korean J. Food Sci. Technol. 1998, 30, 82–89.
- Song, K. The Changes of the Spatial Characteristics in Production of Salted Seafood. J. Korean Geogr. Soc. 2009, 43, 159–172.
- JIangm, S. T.; Moody, M. W.; Chen, H. C. Purification and Characterization of Proteases from Digestive Tract of Grass Shrimp (Penaeus Monodon). J. Food Sci. 1991, 56(2), 322–326. DOI: 10.1111/j.1365-2621.1991.tb05271.x.
- Oh, S.-W.; Kim, Y.-M.; Nam, E.-J.; Jo, J.-H. Proteolytic Properties of Saewoojeot (Salted and Fermented Shrimp) on Meat Proteins. Korean J. Food Sci. Technol. 1997, 29, 1191–1195.
- Yongsawatdigul, J.; Rodtong, S.; Raksakulthai, N. Acceleration of Thai Fish Sauce Fermentation Using Proteinases and Bacterial Starter Cultures. J. Food Sci. 2007, 72(9), M382–M390. DOI: 10.1111/j.1750-3841.2007.00532.x.
- Sila, A.; Nasri, R.; Bougatef, A.; Nasri, M. Digestive Alkaline Proteases from the Goby (Zosterisessor Ophiocephalus): Characterization and Potential Application as Detergent Additive and in the Deproteinization of Shrimp Wastes. J. Aquat Food Prod. Technol. 2012, 212, 118–133. DOI:10.1080/10498850.2011.587149.
- Kim, J.-S.; Kim, K.H. Processing and characterization of salt-fermented fish (jeotgal) using seafood by-products in Korea. In Seafood Processing By-Products: Trends and Applications; Kim, S.-K., Ed.; Springer: New York, NY, USA, 2014; pp. 63–99. DOI: 10.1007/978-1-4614-9590-1_5.
- Jung, M. Y.; Kim, T.-W.; Lee, C.; Kim, J. Y.; Song, H. S.; Kim, Y. B.; Ahn, S. W.; Kim, J. S.; Roh, S. W.; Lee, S. H. Role of Jeotgal, a Korean Traditional Fermented Fish Sauce, in Microbial Dynamics and Metabolite Profiles during Kimchi Fermentation. Food Chem. 2018, 265, 135–143. DOI: 10.1016/j.foodchem.2018.05.093.
- Lee, K.-D.; Choi, C.-R.; Cho, J.-Y.; Kim, H.-L.; Ham, K.-S. Physicochemical and Sensory Properties of salt-fermented Shrimp Prepared with Various Salts. J. Korean Soc. Food Sci. Nutr. 2008, 37(1), 53–59. DOI: 10.3746/jkfn.2008.37.1.53.
- Song, H. S.; Kim, S. H. Effect of Fermentation Temperature and Salt Concentration on Changes in Quality Index of Salted Shrimp during Fermentation. J. Food Hyg. Saf. 2017, 325, 355–362. DOI:10.13103/JFHS.2017.32.5.355.
- Sen, N. P.; Seaman, S. W.; Baddoo, P. A.; Burgess, C.; Weber, D. Formation of N-Nitroso-N-methylurea in Various Samples of Smoked/Dried Fish, Fish Sauce, Seafoods, and Ethnic Fermented/Pickled Vegetables following Incubation with Nitrite under Acidic Conditions. J. Agric. Food Chem. 2001, 49(4), 2096–2103. DOI: 10.1021/jf0011384.
- Park, C.-K.; Kim, W.-J.; Kim, K.-S.; Park, J.-N. Extractive Nitrogenous Constituents in Commercial Saeujeot, a Salted and Fermented Shrimp (Acetes Japonicus). Korean J. Food Sci. Technol. 1996, 28, 1135–1141.
- Guan, L.; Cho, K. H.; Lee, J.-H. Analysis of the Cultivable Bacterial Community in Jeotgal, a Korean Salted and Fermented Seafood, and Identification of Its Dominant Bacteria. Food Microbiol. 2011, 28(1), 101–113. DOI: 10.1016/j.fm.2010.09.001.
- Lee, S. H.; Jung, J. Y.; Jeon, C. O. Effects of Temperature on Microbial Succession and Metabolite Change during saeu-jeot Fermentation. Food Microbiol. 2014, 38, 16–25. DOI: 10.1016/j.fm.2013.08.004.
- Mah, J.-H.; Han, H.-K.; Oh, Y.-J.; Kim, M.-G.; Hwang, H.-J. Biogenic Amines in Jeotkals, Korean Salted and Fermented Fish Products. Food Chem. 2002, 79(2), 239–243. DOI: 10.1016/S0308-8146(02)00150-4.
- Shim, K. B.; Han, H. G.; An, B. K.; Lee, W. J.; In, J. J.; Song, H.-S. Comparison of the Physiochemical Compositions and Biogenic Amine Contents of Salt-fermented Fishery Products Fromy Different Raw Materials. Korean J. Fish. Aquat. Sci. 2021, 54, 835–840. DOI: 10.5657/KFAS.2021.0835.
- Hwang, I. M.; Lee, H. M.; Lee, H.-W.; Jung, J.-H.; Moon, E. W.; Khan, N.; Kim, S. H. Determination of Toxic Elements and Arsenic Species in Salted Foods and Sea Salt by ICP–MS and HPLC–ICP–MS. ACS omega. 2021, 6(30), 19427–19434. DOI: 10.1021/acsomega.1c01273.
- Peralta, E. M.; Hatate, H.; Kawabe, D.; Kuwahara, R.; Wakamatsu, S.; Yuki, T.; Murata, H. Improving Antioxidant Activity and Nutritional Components of Philippine salt-fermented Shrimp Paste through Prolonged Fermentation. Food Chem. 2008, 111(1), 72–77. DOI: 10.1016/j.foodchem.2008.03.042.
- Jang, K.-S.; Kim, M.-J.; Oh, Y.-A.; Kim, I.; No, H.-K.; Kim, S.-D. Effects of Various sub-ingredients on Sensory Quality of Korean Cabbage Kimchi. J. Korean Soc. Food Nutr. 1991, 20, 233–240.
- Park, W.-P.; Kim, Z.-U. The Effect of Seasonings and salted-fermented Fish on Kimchi Fermentation. Appl. Biol. Chem. 1991, 34, 242–248.
- Ku, K.-H.; Sunwoo, J.-Y.; Park, W.-S. Effects of Ingredients on the Its Quality Characteristics during Kimchi Fermentation. J. Korean Soc. Food Sci. Nutr. 2005, 34, 267–276. DOI: 10.3746/jkfn.2005.34.2.267.
- Woo, M.; Choi, J. R.; Kim, M.; Jang, M.-S.; Cho, E. J.; Song, Y. O. Physicochemical Characteristics of seafood-added Kimchi during Fermentation and Its Sensory Properties. J. Korean Soc. Food Sci. Nutr. 2012, 41(12), 1771–1777. DOI: 10.3746/jkfn.2012.41.12.1771.
- Park, D.-C.; Park, J.-H.; Gu, Y.-S.; Han, J.-H.; Byun, D.-S.; Kim, E.-M.; Kim, Y.-M.; Kim, S.-B. Effects of salted-fermented Fish Products and Their Alternatives on Nitrite Scavenging Activity of Kimchi during Fermentation. Korean J. Food Sci. Technol. 2000, 32, 942–948.
- Park, D.-C.; Kim, E.-M.; Kim, E.-J.; Kim, Y.-M.; Kim, S.-B. The Contents of Organic Acids, Nucleotides and Their Related Compounds in Kimchi Prepared with salted-fermented Fish Products and Their Alternatives. Korean J. Food Sci. Technol. 2003, 35, 769–776.
- Northrop, J. H. A Convenient Method for the Formol Titration. J. Gen. physiol. 1926, 96, 767. DOI:10.1085/jgp.9.6.767.
- Duncan, D. B. Multiple Range and Multiple F Tests. Biometrics. 1955, 11(1), 1–42. DOI: 10.2307/3001478.
- Chung, S.-Y.; Sung, N.-J.; Lee, J.-M. The Taste Compounds in Fermented Entrails of Trepang, Stichopus Japonicus. J. Korean Soc. Food Sci. Nutr. 1981, 10, 1–16.
- Chung, S.-Y.; Sung, N.-J.; Lee, Y.-K. Compositions in Amino Acids and Nucleotides of Fermented Entrails of Yellow Corvina. J. Korean Soc. Food Sci. Nutr. 1984, 13, 285–290.
- Jung, J. Y.; Lee, S. H.; Jeon, C. O. Kimchi Microflora: History, Current Status, and Perspectives for Industrial Kimchi Production. Appl. Microbiol. Biotechnol. 2014, 98(6), 2385–2393. DOI: 10.1007/s00253-014-5513-1.
- Shim, S.-M.; Kim, J. Y.; Lee, S. M.; Park, J.-B.; Oh, S.-K.; Kim, Y.-S. Profiling of Fermentative Metabolites in Kimchi: Volatile and non-volatile Organic Acids. J. Korean Soc. Appl. Biol. Chem. 2012, 554, 463–469. DOI:10.1007/s13765-012-2014-8.
- Doores, S. Organic Acids. In Antimicrobials in Foods; Davidson, P. M., Branen, A. L., Eds.; Marcel Dekker. Inc.: New York, NY, 2005; Vol. 145. 91. DOI:10.1201/9781420028737.
- Knarreborg, A.; Miquel, N.; Granli, T.; Jensen, B. Establishment and Application of an in Vitro Methodology to Study the Effects of Organic Acids on Coliform and Lactic Acid Bacteria in the Proximal Part of the Gastrointestinal Tract of Piglets. Anim. Feed Sci. Technol. 2002, 99(1–4), 131–140. DOI: 10.1016/S0377-8401(02)00069-X.
- Cho, Y.-J.; Jung, M.-H.; Kim, B.-K.; Jung, W.-Y.; Gye, H.-J.; Jung, H.-J. Effect of Raw Material Freshness on Quality and Safety of Anchovy Fish Sauce. J. Fisher. Marine Sci. Edu. 2015, 274, 1194–1201. DOI:10.13000/JFMSE.2015.27.4.1194.
- Wang, H.; Zhang, F.; Cao, J.; Zhang, Q.; Chen, Z. Comparison of Chromatographic and Titrimetric Methods for the Determination of the a-amino Nitrogen in Standard Solution and Fish Protein Hydrolysates. J. Food Res. 2012, 1(4), 174. DOI: 10.5539/jfr.v1n4p174.
- Lee, K.-G.; Kim, S.-M. Quality Changes in low-salted Squid Jeot-gal during Fermentation and Determination of shelf-life. J. Korean Soc. Food Sci. Nutr. 2012, 41(5), 687–694. DOI: 10.3746/jkfn.2012.41.5.687.
- Choi, Y.-J.; Lee, H.-W.; Yang, J.-H.; Hong, S.-W.; Park, S.-H.; Lee, M.-A. Changes in Quality Properties of Kimchi Based on the Nitrogen Content of Fermented Anchovy Sauce, Myeolchi Aekjeot, during Fermentation. Food Sci. Biotechnol. 2018, 27(4), 1145–1155. DOI: 10.1007/s10068-018-0349-6.
- Cho, M. S.; Jin, Y. J.; Kang, B. K.; Park, Y. K.; Kim, C.; Park, D. S. Understanding the Ontogeny and Succession of Bacillus Velezensis and B. Subtilis Subsp. Subtilis by Focusing on Kimchi Fermentation. Sci. Rep. 2018, 8(1), 1–10. DOI: 10.1038/s41598-018-25514-5.
- Jeong, S. H.; Lee, S. H.; Jung, J. Y.; Choi, E. J.; Jeon, C. O. Microbial Succession and Metabolite Changes during Long‐term Storage of Kimchi. J. Food Sci. 2013, 78(5), M763–M769. DOI: 10.1111/1750-3841.12095.
- Jung, J. Y.; Lee, S. H.; Jin, H. M.; Hahn, Y.; Madsen, E. L.; Jeon, C. O. Metatranscriptomic Analysis of Lactic Acid Bacterial Gene Expression during Kimchi Fermentation. Int. J. Food Microbiol. 2013, 163(2–3), 171–179. DOI: 10.1016/j.ijfoodmicro.2013.02.022.
- Kang, B. K.; Cho, M. S.; Park, D. S.; Landi, S.; Tonazzini, I.; Cecchini, M.; Piazza, V.; Gemmi, M. Red Pepper Powder Is a Crucial Factor that Influences the Ontogeny of Weissella Cibaria during Kimchi Fermentation. Sci. Rep. 2016, 6(1), 1–8. DOI: 10.1038/srep28232.
- Yang, Z.-Q.; Jiao, X.-A.; Zhou, X.-H.; Cao, G.-X.; Fang, W.-M.; Gu, R.-X. Isolation and Molecular Characterization of Vibrio Parahaemolyticus from Fresh, low-temperature Preserved, Dried, and Salted Seafood Products in Two Coastal Areas of Eastern China. Int. J. Food Microbiol. 2008, 125(3), 279–285. DOI: 10.1016/j.ijfoodmicro.2008.04.007.
- Jung, J. Y.; Lee, S. H.; Lee, H. J.; Jeon, C. O. Microbial Succession and Metabolite Changes during Fermentation of saeu-jeot: Traditional Korean Salted Seafood. Food Microbiol. 2013, 34(2), 360–368. DOI: 10.1016/j.fm.2013.01.009.
- Le, B.; Yang, S. H. Probiotic Potential of Novel Lactobacillus Strains Isolated from salted-fermented Shrimp as Antagonists for Vibrio Parahaemolyticus. J. Microbiol. 2018, 56(2), 138–144. DOI: 10.1007/s12275-018-7407-x.
- Chang, J. Y.; Chang, H. C. Improvements in the Quality and Shelf Life of Kimchi by Fermentation with the Induced Bacteriocin-Producing Strain, Leuconostoc Citreum GJ7 as a Starter. J. Food Sci. 2010, 75(2), M103–M110. DOI: 10.1111/j.1750-3841.2009.01486.x.
- Hou, D.; Huang, Z.; Zeng, S.; Liu, J.; Wei, D.; Deng, X.; Weng, S.; He, Z.; He, J. Environmental Factors Shape Water Microbial Community Structure and Function in Shrimp Cultural Enclosure Ecosystems. Front. Microbiol. 2017, 8, 2359. DOI: 10.3389/fmicb.2017.02359.
- Park, S. E.; Seo, S. H.; Kim, E. J.; Byun, S.; Na, C. S.; Son, H. S. Changes of Microbial Community and Metabolite in Kimchi Inoculated with Different Microbial Community Starters. Food Chem. 2019, 274, 558–565. DOI: 10.1016/j.foodchem.2018.09.032.
- S Hawer, W.-D.; Ha, J.-H.; Seog, H.-M.; Nam, Y.-J.; Shin, D.-W. Changes in the Taste and Flavour Compounds of Kimchi during Fermentation. Korean J. Food Sci. Technol. 1988, 20, 511–517.
- Fox, I. H. Metabolic Basis for Disorders of Purine Nucleotide Degradation. Metabolism. 1981, 30(6), 616–634. DOI: 10.1016/0026-0495(81)90142-6.
- Ryu, K.-Y.; Shim, S.-L.; Kim, W.; Jung, M.-S.; Hwang, I.-M.; Kim, J.-H.; Hong, C.-H.; Jung, C.-H.; Kim, K.-S. Analysis of the Seasonal Change of the Proximate Composition and Taste Components in the Conger Eels (Conger Myriaster). J. Korean Soc. Food Sci. Nutr. 2009, 38(8), 1069–1075. DOI: 10.3746/jkfn.2009.38.8.1069.
- Choi, Y. J.; Yong, S.; Lee, M. J.; Park, S. J.; Yun, Y. R.; Park, S. H.; Lee, M. A. Changes in Volatile and non-volatile Compounds of Model Kimchi through Fermentation by Lactic Acid Bacteria. Lwt. 2019, 105, 118–126. DOI: 10.1016/j.lwt.2019.02.001.
- Lee, M.-A.; Choi, Y.-J.; Lee, H.; Hwang, S.; Lee, H. J.; Park, S. J.; Chung, Y. B.; Yun, Y.-R.; Park, S.-H.; Min, S. Influence of Salinity on the Microbial Community Composition and Metabolite Profile in Kimchi. Fermentation. 2021, 7(4), 308. DOI: 10.3390/fermentation7040308.
- Lee, S. H.; Whon, T. W.; Roh, S. W.; Jeon, C. O. Unraveling Microbial Fermentation Features in Kimchi: From Classical to meta-omics Approaches. Appl. Microbiol. Biotechnol. 2020, 104(18), 7731–7744. DOI: 10.1007/s00253-020-10804-8.
- Song, H. S.; Lee, S. H.; Ahn, S. W.; Kim, J. Y.; Rhee, J. K.; Roh, S. W. Effects of the Main Ingredients of the Fermented Food, Kimchi, on Bacterial Composition and Metabolite Profile. Food Res. Int. 2021, 149, 110668. DOI: 10.1016/j.foodres.2021.110668.
- Jung, M. J.; Kim, J.; Lee, S. H.; Whon, T. W.; Sung, H.; Bae, J. W.; Choi, Y.-E.; Roh, S. W. Role of Combinated Lactic Acid Bacteria in Bacterial, Viral, and Metabolite Dynamics during Fermentation of Vegetable Food, Kimchi. Food Res. Int. 2022, 157, 111261. DOI: 10.1016/j.foodres.2022.111261.