ABSTRACT
The effects of gellan gum addition and the ohmic cooking method on the color, starch retrogradation, textural properties, total phenolic contents, and antioxidant activities of refrigerated ready-to-eat cooked brown rice were investigated and compared to those from the conventional cooking method. Brown rice samples of four rice cultivars were used including RD 43, Riceberry, KDML 105, and RD 6. The key objective was to determine the benefits of applying the gellan gum addition and the ohmic cooking method on different quality parameters of the ready-to-eat cooked brown rice products kept in refrigerator. The results indicated that both gellan gum addition and ohmic cooking method led to less color intensity, slower starch retrogradation, a reduction in rice hardness escalation during storage, and greater antioxidant capabilities of the ready-to-eat brown rice products compared to their counterparts. The starch retrogradation was not detected for the samples of 1 night storage; however, it appeared in every sample after 7 days. The retrogradation enthalpy values were different between rice varieties. The effect of gellan gum addition on the starch retrogradation delay was minimal for the glutinous rice. The samples cooked by ohmic method had greater antioxidant activity levels than the conventionally-cooked samples in almost all cases.
Introduction
During recent decades, the socio-economic transformation of cities has led to the alteration of eating behavior from traditional home-cooked meals to ready-to-eat food. The reasons behind this trend may involve the increasing population of working women, hectic work schedules, more disposable income, and on-the-go consumption habits. In addition, the increasing demand of health-conscious consumers has stimulated the ready-to-eat food manufacturers to improve their products especially regarding nutritional quality, while maintaining superior physical and sensorial attributes.
To date, the consumption of ready-to-eat cooked brown rice products is not yet widespread owing to limited published information on how to improve its quality parameters, especially textural characteristics. Starch retrogradation is a key reason for the textural change of cooked rice during storage. Mariotti et al.[Citation1] considered that the starch granules typically swell and develop a sturdy, dense gel network after cooking, with the strength of its structure increasing on cooling because of starch retrogradation. A number of studies have demonstrated that the addition of hydrocolloid could lessen the starch retrogradation and improve texture. For example; Song et al.[Citation2] investigated the effect of three kinds of gums including konjac glucomannan, carrageenan, and gellan gum on the retrogradation of Indica rice starch and found that the addition of carrageenan at 0.2–0.3% w/w could hinder the rice starch retrogradation more than that of gellan gum and glucomannan respectively. Eom et al.[Citation3] applied an edible coating treatment using waxy corn starch with edible gums, such as gellan gum and sodium alginate, on steamed rice cakes to retard starch retrogradation during storage. Although there has been some researches reporting the effects of gum addition on the delay of quality alteration of cooked white rice during refrigeration storage,[Citation4] the exact solution for impeding the quality changes of the cooked brown rice is still lacking.
Cooking brown rice is commonly conducted in electric rice cookers that are generally sold in the market. The heating principle of these rice cookers relies on conventional mechanisms. Firstly, heat is generated by the electrical heater and transferred to the pot by conduction. After that the heat is conveyed to water and rice inside the pot by convection and conduction, respectively which require long cooking times, subsequently leading to nutrient loss. Consequently, an innovative cooking technique that has potential to reduce the cooking period and provide better quality cooked brown rice has been explored. In ohmic heating, the heat is generated inside food by passing an electrical current through the food, with the heat quantity depending on the applied electrical field strength and the electrical conductivity of the food. In other words, the heating rate can be raised by increasing either the electrical field strength or the electrical conductivity of the food. Sarang et al.[Citation5] reported that the key parameters influencing the electrical conductivity of food are its temperature and composition. The addition of ionic substances such as salt can raise the electrical conductivity of the rice-water mixture to make it suitable for ohmic cooking. Jittanit et al.[Citation6] and Kanjanapongkul[Citation7] applied the ohmic heating technique to cooking rice and reported that the properties of rice cooked using the ohmic method were significantly different from those cooked by the conventional method as a result of more uniformity of heat distribution inside the rice-solution mixture and the formation of pores in cell membranes of rice because of the presence of an electric field during ohmic heating.
The current study investigated the effects of gellan gum addition and using the ohmic cooking method on the properties of refrigerated ready-to-eat cooked brown rice compared with those from using the conventional cooking method. The gellan gum was chosen because it can be dissolved by heating to over 90°C and then forming an elastic and brittle gel once cooled; as a result, it was expected to induce the low adhesiveness of cooked rice, retard starch retrogradation, and act as a barrier to the movement of some bioactive substances. The findings from this work would be beneficial for food producers who intend to improve the quality of cooked brown rice and are interested in applying the ohmic heating technique.
Materials And Methods
Raw materials
Paddy samples of four varieties that have exceptional functional characteristics including RD 43 (amylose content 18.82% w/w), Riceberry (amylose content 15.60% w/w), KDML 105 (amylose content 11.39% w/w), and RD 6 (amylose content 5.48% w/w) were applied in this study. RD 43 has a moderate glycemic index which is considered low compared to other rice varieties[Citation8] whereas after cooking it has a soft texture and satisfactory odor.[Citation9] Riceberry, a purple-black color rice, is renowned for its high antioxidants, particularly anthocyanins, together with free fatty acids and iron.[Citation10] KDML 105 (so-called jasmine rice) is the most famous aromatic rice of Thailand owing to its exceptional aroma with a soft and a little sticky texture when cooked. RD 6 is a glutinous rice cultivar that has fascinating characteristics because of its softness, cooking quality, and aroma[Citation11] together with its high contents of vitamin E, iron, folic acid, and various phenolic compounds.[Citation12] The sample of the RD 43 variety was supplied by Tung Wat Sing Agricultural Cooperative Co., Ltd., Chai Nat, Thailand while the sample of Riceberry was purchased from Nang Sa-Ngob Community Enterprise, Phra Nakhon Sri Ayutthaya, Thailand. The Ubon Ratchathani Rice Research Center, Ubon Ratchathani, Thailand supplied the paddy samples of the KDML 105 and RD 6 varieties. All the paddy of each variety were collected from the same growing season and applied in the experiments within 4 months after having been harvested. The paddy samples had initial moisture contents between 12–15% on a dry basis (d.b.). The brown rice samples were prepared by dehusking the paddy using a rice milling machine (model NW 1000 turbo, Natrawee Technology Co., Ltd., Chachoengsao, Thailand). The brown rice samples were packed in the polyethylene plastic packages and kept at approximately 0 °C in a coldroom.
Cooking
Conventional method: An electric rice cooker (model CR-110 T, OTTO Kingglass Co., Ltd., Bangkok, Thailand) was used for cooking all the brown rice samples. All the cooking conditions are presented in . The proportions of deionized water and gellan gum used in this study were selected from the previous study[Citation13] by considering the cooked brown rice qualities obtained from the different deionized water and gellan gum ratios. Before cooking, the brown rice samples of RD 43, Riceberry, and KDML 105 varieties were soaked for 30 min in the deionized water while the soaking period was 2 h for the RD 6 variety as it is a glutinous rice. The soaking water was then used in the cooking step. All samples sizes were 600 g in every cooking experiment. For cooking conditions that applied gellan gum, the soaking water was poured out of the brown rice and added by the specified ratio of gellan gum prior to stirring using a magnetic stirrer to entirely dissolve the gum and then putting the solution back onto the brown rice. In the cooking step, the temperatures were taken applying a type-T thermocouple probe placed in the water at the midpoint of mixture sample and connected to a data logger. The sample was heated from around 30 to 105 °C during the cooking mode using the electric rice cooker before turning to the warm mode to hold the temperature at 105.0 ± 0.5 °C for 10 min. The cooking mode spent times for 43, 35, 41, and 26 min for the RD 43, Riceberry, KDML 105, and RD 6 samples respectively. After cooking, the brown rice samples were instantly filled in microwavable plastic trays (EKA PAK Co., Ltd., Chachoengsao, Thailand) that had the width, length, height, and thickness of 118, 179, 40.5 mm and 800 ± 50 μm, respectively. The plastic trays containing the cooked brown rice were shielded with a white-color lidding film (thickness of 84 ± 10% μm) (Bangkok Foil Co., Ltd., Samut Sakhon, Thailand). The samples were stored at 4–10°C in a refrigerator prior to the quality measurement.
Table 1. Conditions for cooking experiments.
Ohmic heating method: The brown rice samples were prepared applying the identical process to the conventional method; except that for this method, the soaking water was separated from the brown rice and added with a calculated amount of iodized table salt before cooking. An amount of salt (0.005 M concentration) was added for escalating the electrical conductivity of the brown rice-water mixture because the mixture was not electrical-conductive. The schematic diagram showing the ohmic heating system applied in the present work is depicted in . The 200 mm cubical-shaped ohmic cell was constructed from an acrylic plate material (5 mm thickness). The electrodes (width and length of 198 mm) were made of titanium grade Gr-2 and placed on both left-right walls of the ohmic cell. The gap between the left and right electrodes was 192 mm. An agitator was mounted on the cover of the ohmic cell to mix the sample during cooking. The agitator was directly driven by a gear motor with an electrical voltage of 12 V DC and a maximum current of 3 A. The material of the agitator shaft was Teflon, an electrical insulator (diameter of 8 mm). The agitator speed could be adjusted up to 200 rpm by a voltage-regulated controller. The temperatures of samples were measured and recorded throughout the cooking process using a type-T thermocouple connected to a data logger. The temperature profiles of samples during ohmic cooking were regulated to be similar to those of the conventional cooking method by manually adjusting the applied electrical voltage using a variable-voltage transformer. It must be noted that there were two voltage transformers applied in the cooking experiments as shown in , consisting of a variable transformer with input/output voltages of 220 V AC/0–250 V AC, a power capacity of 5 KVA and a maximum electric current of 20 A (model TSGC2-5 K, Stable Transformer Co., Ltd., Pathum Thani, Thailand) and an invariable transformer with its input/output voltages of 220 V AC/350 V AC, a power capacity of 7 KVA and a maximum electric current of 20 A (model STB 301, Stable Transformer Co., Ltd., Pathum Thani, Thailand). All samples were instantly packed after cooking and the same method was used as for the conventional cooking.
Quality determination
The color values, starch retrogradation levels and textural properties of all cooked brown rice samples were determined after refrigerated storage for 1 night (approximately 16 h) and 7 days. In addition, the total phenolic content and antioxidant activity values of the cooked brown rice samples that were kept in refrigeration storage for 1 night were examined to compare between both cooking methods and to investigate the effect of the gellan gum addition. Prior to all measurements, the refrigerated samples were warmed using a microwave oven (model R380I, Sharp Appliances (Thailand) Co., Ltd, Bangkok, Thailand) at 1200 W for 90 seconds in order to simulate the common practice of most consumer that warm the refrigerated ready-to-eat product in microwave oven before eating and be along with the instructions on the labels of some refrigerated ready-to-eat rice meals.
Color: The color values of the ready-to-eat cooked brown rice samples were determined in triplicate using a spectrophotometer (UltraScan PRO, HunterLab Corporation, VA, USA) based on the L*, a*, b* scales, where L* denotes lightness (0 < L < 100), whereas a* (+), a* (-), b* (+), and b* (-) denote redness, greenness, yellowness, and blueness, respectively. The instrument was calibrated applying a standard white plate with L*, a*, and b* values of 94.2, 0.3157, and 0.3320, respectively.
Starch retrogradation: The levels of starch retrogradation of the ready-to-eat cooked brown rice samples were examined in duplicate applying a differential scanning calorimeter (model DSC 1, Mettler-Toledo International Inc., OH, USA). The measurement method was adapted from Yu et al.[Citation14] After warming in the microwave oven, the ready-to-eat cooked brown rice samples were mixed with 95% ethanol (1:4, v/v) for dehydration before hot-air drying at 37 °C in oven (model FED 53, Binder GmbH, Tuttlingen, Germany) for 24 h. Afterward, the samples were pulverized in blender (model HR2115, Philips Electronics (Thailand) Ltd., Bangkok, Thailand) and screened on a laboratory 100-mesh sieve (model D‐42759, Retsch GmbH, Hann, Germany). Approximately 4 mg (dry matter) of brown rice flour was put in a stainless-steel pan prior to adding deionized water at 1:2 w/w flour-to-water ratio. Then, the pan was firmly sealed and placed for 24 h at room temperature to equilibrate. The measurements were carried out in the differential scanning calorimeter by heating the pan from 20 to 140 °C at a rate of 10 °C/min. The data acquired from each measurement were plotted as a thermogram containing the onset (To), peak (Tp), and conclusion (Tc) temperatures with the calculation of melting enthalpy (∆H).
Instrumental textural properties: The textural properties of the ready-to-eat cooked brown rice samples were tested using a texture analyzer (model TA.XT. Plus, Stable Micro Systems Ltd., Godalming, UK). Four rice kernels were selected and placed in a row on a metal plate. The rice kernels were compressed using a 36 mm diameter cylindrical until 60% deformation. The specified pretest and posttest speeds were 1 and 0.5 mm/s, correspondingly. The measurement was carried out in three replications. The force-deformation data were analyzed whereas the mean hardness and adhesiveness (stickiness) values were calculated.
Total phenolic content: The ready-to-eat cooked brown rice samples were determined for their total phenolic contents by applying Folin-Ciocalteu reagent. The method was adapted from Wolfe et al.[Citation15] In the measurement, gallic acid was applied for calibrating the standard graph using the concentration range between 0 and 50 μM. The 0.2 ml of methanolic extract sample was added into 1 ml of 1:10 diluted Folin-Ciocalteu reagent. After 6 min, the 2 ml of saturated sodium carbonate was also added. After that, the sample was left for 90 min in the dark at room temperature. The absorbance value was examined at 750 nm applying a Genesys 10S UV-visible spectrophotometer (Thermo Fisher Scientific, Waltham, MA, USA). The total phenolic content values of the extracts were evaluated and then indicated as milligram gallic acid equivalent per gram of sample weight (mg GAE/g). The measurement was carried out in three replications for every sample.
Antioxidant activity: The antioxidant activity for each ready-to-eat cooked brown rice sample was measured based on the scavenging activity of the stable 1, 1-diphenyl-2-picrylhydrazyl (DPPH) free radical, applying the method adapted from Tachibana et al.[Citation16] A calibration graph was generated applying Trolox as an external standard with the range of concentration between 10 and 50 μM. One ml of 200 μM DPPH was added into 1 ml of methanolic extract sample prior to incubation for 30 min in the dark at room temperature. After that, the absorbance was examined applying the spectrophotometer at 515 nm. The antioxidant activity values were recorded as milligrams Trolox equivalent per gram of sample weight (mg TE/g). The measurement was carried out in three replications.
Statistical analysis
Analysis of variance and Duncan’s multiple range test was carried out using the SPSS software package (version16.0; SPSS Inc.; Chicago, IL, USA).
Results And Discussion
Color of ready-to-eat cooked brown rice
The results of the color measurement of the ready-to-eat cooked brown rice samples are presented in . The 7 day period is a common shelf-life under refrigerated condition of the ready-to-eat cooked brown rice product in the market. It appeared that the color values were related to the rice variety. The lowest lightness and yellowness values were in the ready-to-eat cooked brown rice of the Riceberry variety whereas the redness of this variety was the highest because its rice kernel has a unique purple-black color, mainly caused by anthocyanins (cyanidin-3-O-glucoside, and peonidin-3-O-glucoside) and phytosterols (γ-oryzanol, β-sitosterol, and triterpene alcohol).[Citation17,Citation18] The appearances of cooked brown rice samples of RD 43 variety that has regular brown rice color and those of Riceberry variety with purple-black color are illustrated in . Comparing among the three varieties of brown rice that had a rather common color (RD 43, KDML 105, and RD 6), it appeared that in overall RD 43 had the lowest lightness and yellowness followed by KDML 105 and RD 6, correspondingly, whereas the redness values were rather similar among these three varieties. The variation in their bran layer thicknesses and in the components such as amylose, protein, minerals, and pigments should be the key reasons for these results. Moongngarm et al.[Citation19] de-hulled and polished the paddy rice of four varieties including non-waxy rice (KDML 105 and Red rice) and waxy rice (RD 6 and Black rice) using rice de-husker and rice milling machine with the same degree of milling (8%). They reported that the amounts of rice bran and bran layer getting from the milling and polishing steps are apparently different among four varieties. Furthermore, they demonstrated that the proximate compositions of rice bran and bran layer of each rice variety are diverse.
Table 2. Color values of ready-to-eat cooked brown rice samples after 1 night and 7 days of storage.
Figure 2. The images of the ready-to-eat cooked brown rice products after 7 nights of refrigeration storage: (a) RD 43 added with gellan gum 0.3% and conventional cooking; (b) RD 43 added with gellan gum 0.3% and ohmic cooking; (c) Riceberry added with gellan gum 0.3% and conventional cooking; (d) Riceberry added with gellan gum 0.3% and ohmic cooking.
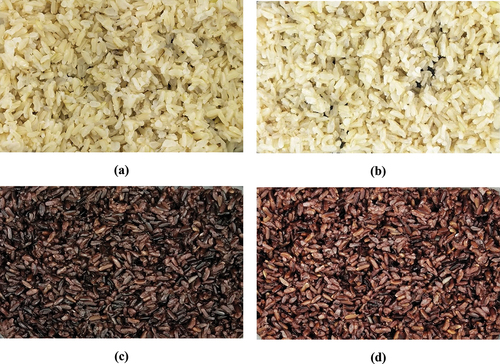
The addition of gellan gum at the amount specified in resulted in a slight increase of lightness values in most samples whereas in overall the redness and yellowness values decreased slightly because the added gellan gum would cover the rice surface and subsequently diminish the impact of components such as amino acids and reducing sugars contained in the rice bran when exposed to heat. Consequently, the browning reaction would decrease. Azarakhsh et al.[Citation20] reported that a gellan gum-based coating provided a selective barrier to oxygen, carbon dioxide, and moisture; thus, it can delay the discoloration and browning of a food product. It appeared that lengthening the storage period from 1 night to 7 days did not result in any noticeable color change in the ready-to-eat cooked brown rice samples. The low storage temperature at 4–10°C might have been the reason for the color stability of samples during storage for a week. Furthermore, the color measurement results indicated that the ohmic cooking method likely produced a lower color intensity of products compared to the conventional cooking method. The possible reason for this was that the ohmic method directly generated heat inside the brown rice samples whereas the conventional method, based on using an electric rice cooker, produced heat from an electric heater at a higher temperature and then transferred heat to the sample via heat conduction and convection mechanisms. Therefore, the conventionally cooked samples were exposed to a more severe source of heat than their counterparts leading to the greater occurrence of browning reaction. The addition of iodized table salt before ohmic cooking might have been another cause of the less intense color of products because NaCl can help to inhibit the browning reaction of some food items. Loypimai et al.[Citation21] demonstrated that soaking glutinous rice in NaCl solution during the soaking step of parboiled rice production could improve the visual color of the parboiled rice product, especially when using NaCl solution at a higher concentration.
Starch retrogradation
The values of the transition temperatures and retrogradation enthalpies of the ready-to-eat cooked brown rice samples after refrigerated storage for periods of 1 night and 7 days are shown in . There was no peak of retrogradation enthalpy in the thermograms obtained from the measurement of all samples that were kept in the refrigerator for 1 night. In contrast, a peak of retrogradation enthalpy was found for every sample that was stored for 7 days. Referring to Yu et al.,[Citation14] the maximum value of the retrogradation enthalpies of cooked milled rice samples were almost achieved after 7 days of storage at 4 °C.
Table 3. Transition temperature, retrogradation enthalpy, and textual property of ready-to-eat brown rice products after 1 night and 7 days of storage.
The results in indicated that the values of transition temperatures and the retrogradation enthalpy were not much different among all rice varieties. The ranges of transition temperatures were 52.57–64.66, 51.94–64.95, 51.17–64.08, and 50.86–62.91 °C for the RD 43 (18.82% w/w amylose content), Riceberry (15.60% w/w amylose content), KDML 105 (11.39% w/w amylose content), and RD 6 varieties (5.48% w/w amylose content), correspondingly. It appeared that the transition temperatures of samples were correlated with their amylose contents. Yu et al.[Citation14] stated that the cooked milled rice with a greater amylose content would have a higher transition temperature than rice containing a lower amylose content. The ranges of retrogradation enthalpy values were 0.39–1.94, 0.75–2.18, 0.81–2.14, and 0.60–2.33 J/g for the RD 43, Riceberry, KDML 105, and RD 6 varieties, respectively. The explanation is that commonly, amylopectin has a highly branched nature and the double helices that form are all connected to the same backbone of the polymer and are in closer proximity. Consequently, they would aggregate more easily to form crystals than amylose leading to a greater value of retrogradation enthalpy. Li et al.[Citation22] claimed that the distribution of the starch chain length apparently affected the retrogradation process. The amylose molecules with shorter-to-medium length chains tended to have faster and stronger interactions along the storage duration at 4 °C.
It was interesting that the gellan gum addition could significantly lessen the retrogradation of starch during storage. The effect of gellan gum addition on the retardation of starch retrogradation was slight for the RD 6 variety which is a glutinous rice. After dissolving in water, gellan gum forms a strong gel and covers the brown rice surface generating the barrier for the amylose movement and consequently delaying the starch retrogradation.
As previously described, the starch retrogradation was undetectable in every sample that was stored in the refrigerator overnight. The study of Yu et al.[Citation14] demonstrated that retrogradation enthalpies were found in cooked milled rice samples of all 11 varieties that were kept at 4 °C for 0 and 1 day. Therefore, it is expected that the existing bran layer could hurdle the starch retrogradation in the cooked brown rice because the rice bran layer is composed of protein, fiber, and lipid that can resist the water removal from the cooked rice. According to Mariotti et al.,[Citation1] the constituents in the surface layer of brown rice help in reducing the starch retrogradation level to lower than that of cooked white rice along the duration of refrigerated storage at 4 °C.
The ohmically-cooked brown rice samples had significantly lower retrogradation enthalpy values than the conventionally-cooked samples implying that ohmic heating had some effect on the starch structure. Yang et al.[Citation23] revealed that the ohmic method caused a more porous structure within the cooked rice compared to the conventional cooking method due to electroporation. The electric field created the formation of pores in the cell membrane, leading to higher membrane permeability and diffusivity. The porous microstructure of brown rice cooked by ohmic method helps to simplify the diffusion of some constituents from the rice bran into the rice kernel, leading to the obstruction of starch retrogradation. In addition, Kanjanapongkul[Citation7] reported that the intermolecular links of starch molecules break and then develop hydrogen bonding with the surrounding water during starch gelatinization, whereas the application of an electric field in ohmic heating could boost the diffusion of moisture into the rice kernel and subsequently influence the gelatinization process. In addition to the moisture diffusion improvement, the diffusion of some constituents; for instance, lipid, proteins, and soluble dietary fiber from the bran layer into the rice kernel might escalate when using ohmic heating technique. The diffusion of these components resulted in better retardation of starch retrogradation compared to the conventional cooking method. Yu et al.[Citation14] claimed that constituents such as protein and lipid can influence the starch retrogradation of cooked rice; nevertheless, the relationships between the protein, lipid, and starch retrogradation are complex. Likewise, Wu et al.[Citation24] pointed out that some carbohydrates, polyphenols, proteins, and lipids could obstruct the occurrence of starch retrogradation to some extent. In addition, with the ohmic cooking technique, 0.005 M of NaCl was added into the samples to raise their electrical conductivity. This salt addition can lead to a stronger gel structure of the gellan gum within the cooked brown rice samples and consequently, starch retrogradation was retarded by the gellan gum more efficiently than without salt addition. Moritaka et al.[Citation25] stated that the addition of NaCl affected the binding of the gellan gum helices, resulting in greater gel strength.
There are some studies reporting that the NaCl addition could delay the retrogradation of the starch gels such as Wang et al.[Citation26] and Huang et al.[Citation27] Wang et al.[Citation26] demonstrated that the increasing NaCl concentrations diminish the amount of free water in the starch, the zeta potential of the starch gels, and consequently re-association of starch molecules. Huang et al.[Citation27] pointed out that NaCl protected the granular morphology of starch and increased the aggregation of starch granules. However, the concentration of NaCl applied in the present study was very low compared to the aforementioned studies; therefore, the retardation of starch retrogradation should be mainly caused by the ohmic cooking method rather than the NaCl addition.
Textural properties
The hardness and adhesiveness values of the ready-to-eat cooked brown rice after the periods of 1 night and 7 days of refrigerated storage are shown in . The highest hardness and lowest adhesiveness values were in the samples of the RD 43 variety which had the highest amylose content among all samples. As expected, the glutinous brown rice samples (RD 6 variety), which had the minimum amylose content compared to their counterparts, had the lowest hardness and highest adhesiveness values. The Riceberry samples, which had a slightly higher level of amylose content than the KDML105 samples, had lower hardness and adhesiveness values than KDML105. The greater amylose content should account for these lower adhesiveness results whereas their bran layer thickness and brown rice composition would be the causes of their lesser hardness. Isnaini et al.[Citation28] reported that the total dietary fiber values of brown rice of the Riceberry variety were more than those of the KDML105 variety, while Kamjijam et al.[Citation29] mentioned that Riceberry had a higher protein content (9.19% w/w) than KDML 105 (7.12% w/w). Clearly, lengthening the storage period from 1 night to 7 days resulted in a significant increase in the hardness values whereas there was a reduction in adhesiveness only in some cases. This result was consistent with Yu et al.[Citation30] who found that the hardness of cooked rice increased while the adhesiveness declined during the first 7 days of refrigerated storage. Starch retrogradation was considered the key reason for the hardness escalation during storage. Yu et al.[Citation14] pointed out that the hardness and adhesiveness of cooked rice were associated with its amylose content and starch retrogradation. Starch retrogradation causes the hardness to increase and the adhesiveness to decrease in cooked rice, while rice with a higher amylose content is likely to have a harder texture. Nonetheless, they noted that other components such as protein and lipid and the starch structure also affect the texture of cooked rice.
The addition of gellan gum significantly increased the hardness values of the samples stored for 1 night but it did not significantly affect the adhesiveness values, probably because the hardness escalation was caused by the gel strength of the hydrocolloid layer covering the rice surface. However, after 7 days of refrigerated storage, the samples with the gellan gum added had lower hardness than without gellan gum addition. In other words, although the gellan gum caused a significant increase in the hardness of the ready-to-eat cooked brown rice samples due to the gellan gum layer covering the rice surface, the gellan gum could significantly delay the escalation of rice hardness during long term storage. This result was consistent with the starch retrogradation level that was clearly hurdled by the gellan gum as described in the previous section. Starch retrogradation was deemed as the main reason for the hardness increase because of the development of the stronger bonding and the ejection of moisture from the starch gel structure.
Another result of interest in the current study was the effect of the ohmic cooking method on the texture of the ready-to-eat cooked brown rice. After 7 days of refrigerated storage, the ohmically-cooked samples had significantly lower hardness values than from the conventional cooking method. Again, this result was consistent with the starch retrogradation levels of samples. The explanation for the reduction in starch retrogradation of the ohmically-cooked brown rice samples compared to the conventionally-cooked samples was described in the prior section.
Total phenolic content and antioxidant activity
The total phenolic content and antioxidant activity values of the ready-to-eat brown rice samples that were kept in refrigerated storage for 1 night are presented in . The samples after storing in the refrigerated condition for 7 days were not determined for their total phenolic content and antioxidant activity values since these values are usually affected by heat much more than that by the low temperature storage in refrigerator for a week. The samples of the Riceberry variety had the greatest total phenolic contents and antioxidant activity values among all samples with there being little difference among the other varieties. This was not surprising because Riceberry is a Thai black-pigmented rice whose bran contains significant levels of antioxidants and other important constituents, such as anthocyanins.[Citation10] Waewkum and Singthong[Citation31] reported that Thai pigmented brown rice contained apparent amount of total phenolic compounds, flavonoids, and antioxidant activities, particularly in Riceberry. They demonstrated that the values of total phenolic compounds and antioxidant activities of the flour of Riceberry brown rice are 8.514 mg GAE/g sample and 1.227 mg TE/g sample respectively. These values are different from the results of cooked brown rice in the present study undoubtedly due to the effects of soaking and cooking process. González-Aguilar et al.[Citation32] indicated that the total phenolic compounds and antioxidant activities of the apple flesh which is well-known for its high in total phenolic compounds and antioxidant activities are in the ranges of 164–561 mg GAE/g sample and 890–2090 mg TE/g sample correspondingly depending on its variety. Although the values of the cooked brown rice are much lower than those of the apple, the rice is a staple food that is daily consumed in the larger amount than apple especially for the Asian people.
Figure 3. Total phenolic content of ready-to-eat brown rice products after 1 night of refrigeration storage:



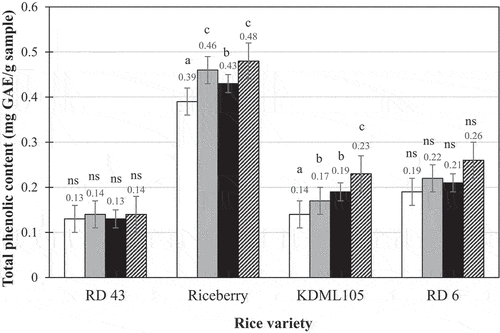
Figure 4. Antioxidant activities of ready-to-eat brown rice products after 1 night of refrigeration storage:



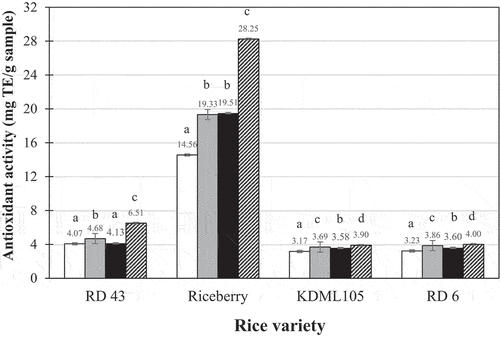
The addition of gellan gum led to the higher antioxidant activity values for the ready-to-eat brown rice samples than for the samples without gellan gum addition whereas it resulted in significantly greater total phenolic contents only in the samples of the Riceberry and KDML105 varieties. Rukmanikrishnan et al.[Citation33] prepared biopolymers of gellan gum and 2-hydroxyethyl cellulose, and lignin-based binary and ternary sustainable composites for use in food packaging and biomedical applications and found that the gellan gum film alone (without any 2-hydroxyethyl cellulose or lignin) had low antioxidant activity even at a high concentration. This implied that the gellan gum itself has low antioxidant activity. Moreira et al.[Citation34] pointed out that gellan gum has exceptional colloidal and gelling properties; hence, it has the potential to form a good, edible coating. The results of their study demonstrated that the application of either gellan gum-based edible coatings or gellan gum-based edible coatings with apple fiber addition in the formulation could preserve the antioxidant activity potential of the fresh-cut apples. Similarly, Robles-Sanchez et al.[Citation35] indicated that the gellan gum-based coating helped in preserving the antioxidant capacity of fresh-cut mangoes. The explanation for the positive effect of gellan gum addition on the preservation of antioxidant activity and phenolic compounds in the ready-to-eat brown rice samples was that the gellan gum layer could entrap some antioxidants and phenolic compounds moving out of the rice kernels during cooking.
It was interesting that the samples cooked using the ohmic method had greater antioxidant activity levels than the conventionally-cooked samples in almost all cases. The first explanation for this is that the electroporation caused by the ohmic method led to the formation of pores in the cell membranes of the brown rice kernels. Consequently, the antioxidants existing in the rice bran could diffuse more easily into the rice kernel and be protected from heat damage compared to the conventional cooking method. In the conventional cooking method, the antioxidants could not easily diffuse into the rice kernel; therefore, many of them were likely to leach out to the surrounding water and be exposed to heat. Several studies revealed that the high heating temperatures and the longer times used during cooking influenced the degradation of phenolic compounds and the antioxidant capacity in pigmented rice.[Citation36,Citation37] Salt addition in the ohmic cooking technique would be another reason for the greater antioxidant activity levels in the ohmically-cooked samples. As mentioned in the previous section, Moritaka et al.[Citation25] and Hui and Sherkat[Citation38] reported that the addition of NaCl impacted on the binding of the gellan gum helices resulting in an escalation in the gel strength. The stronger gel structure could protect against antioxidant loss more efficiently.
Conclusion
The current study proved that the addition of gellan gum in the cooking of brown rice resulted in less color intensity, delayed starch retrogradation, a reduced increase in rice hardness during storage, and higher antioxidant capability levels in the ready-to-eat brown rice products compared to those without the addition of the gum. Similar to the effects of gellan gum addition, the ohmic cooking method provided some advantages over the conventional method including slightly less dark color, slower starch retrogradation, a reduced rate of hardness escalation, and greater antioxidant activity levels in the products. The retrogradation of starch was not detected in all samples that were kept in the refrigerator overnight. Nevertheless, it appeared in every sample that was stored for 7 days. The effect of gellan gum addition on the retardation of starch retrogradation was least for the RD 6 variety that is a glutinous rice. Although the ohmic cooking method had some benefits, the addition of salt to increase the electrical conductivity of the brown rice-water mixture could adversely influence the product taste and slightly increase the sodium intake of the consumer. The addition of other ionic constituents apart from salt should be explored in the future.
Abbreviation
L*, Lightness; a* (+), Redness; a* (-), Greenness; b* (+), Yellowness; b* (-), Blueness; To, Onset temperature; Tp, Peak temperature; Tc, Conclusion temperature; ∆H, melting enthalpy; DPPH, 2,2-diphenyl-1-picrylhydrazyl; di(phenyl)-(2,4,6-trinitrophenyl) iminoazanium)
Acknowledgments
This work was partially supported by the Center for Advanced Studies for Agriculture and Food, Institute for Advanced Studies, Kasetsart University, Bangkok, Thailand under the Higher Education Research Promotion and National Research University Project of Thailand, Office of the Higher Education Commission, Ministry of Education, Thailand.
Disclosure statement
No potential conflict of interest was reported by the author(s).
Additional information
Funding
References
- Mariotti, M; Sinelli, N; Catenacci, F; Pagani, M. A; Lucisano, M. Retrogradation Behaviour of Milled and Brown Rice Pastes during Ageing. J. Cereal Sci. 2009, 49, 171–177. DOI: 10.1016/j.jcs.2008.09.005.
- Song, R.; Min Huang, M.; Li, B.; Zhou, B. The Effect of Three Gums on the Retrogradation of Indica Rice Starch. Nutrients. 2012, 4(6), 425–435. DOI: 10.3390/nu4060425.
- Eom, H.; Chang, Y.; Lee, E. S.; Choi, H. D.; Han, J. Development of a starch/gum-based Edible Coating for Rice Cakes to Retard Retrogradation during Storage. LWT-Food Sci. Technol. 2018, 97, 516–522. DOI: 10.1016/j.lwt.2018.07.044.
- Chung, H. J.; Liu, Q.; Lim, S. T. Texture and in Vitro Digestibility of White Rice Cooked with Hydrocolloids. Cereal Chem. 2007, 84(3), 246–249. DOI: 10.1094/CCHEM-84-3-0246.
- Sarang, S.; Sastry, S. K.; Knipe, L. Electrical Conductivity of Fruits and Meats during Ohmic Heating. J. Food Eng. 2008, 87, 351–356. DOI: 10.1016/j.jfoodeng.2007.12.012.
- Jittanit, W.; Khuenpet, K.; Kaewsri, P.; Dumrongpongpaiboon, N.; Hayamin, P.; Jantarangsri, K. (Ohmic Heating for Cooking Rice: Electrical Conductivity Measurements, Textural Quality Determination and Energy Analysis. Innov. Food Sci. Emerg. Technol. 2017, 42, 16–24. DOI: 10.1016/j.ifset.2017.05.008.
- Kanjanapongkul, K. Rice Cooking Using Ohmic Heating: Determination of Electrical Conductivity, Water Diffusion and Cooking Energy. J. Food Eng. 2017, 192, 1–10. DOI: 10.1016/j.jfoodeng.2016.07.014.
- Nilkamheang, T. Evaluation of the Glycemic Index of RD43-rice, and Its Products by Means of in Vitro Glucose Digestion Mimic Techniques. J. Food Technol. 2021, 16(1), 59–77.
- Suklaew, P.; Chusak, C.; Adisakwattana, S. Physicochemical and Functional Characteristics of RD43 Rice Flour and Its Food Application. Foods. 2020, 9, 1912. DOI: 10.3390/foods9121912.
- Leardkamolkarn, V.; Thongthep, W.; Suttiarporn, P.; Kongkachuichai, R.; Wongpornchai, S.; Wanavijitr, A. Chemopreventive Properties of the Bran Extracted from a newly-developed Thai Rice: The Riceberry. Food Chem. 2011, 125, 978–985. DOI: 10.1016/j.foodchem.2010.09.093.
- Thanasilungura, K.; Kranto, S.; Monkham, T.; Chankaew, S.; Sanitchon, J. Improvement of a RD6 Rice Variety for Blast Resistance and Salt Tolerance through marker-assisted Backcrossing. Agronomy. 2020, 10, 1118. doi: 10.3390/agronomy10081118
- Tamprasit, K.; Weerapreeyakul, N.; Sutthanut, K.; Thukhammee, W.; Wattanathorn, J. Harvest Age Effect on Phytochemical Content of White and Black Glutinous Rice Cultivars. Molecules. 2019, 24, 4432. DOI: 10.3390/molecules24244432.
- Tumpanuvatr, T.; Jittanit, W. Quality Improvement of Refrigerated ready-to-eat Cooked Brown Rice by Adding Gellan Gum and Trehalose with Ohmic Heating Compared to Conventional Cooking Method. J. Food Process. Preserv. 2022, 46(4), e16443. DOI: 10.1111/jfpp.16443.
- Yu, S.; Ma, Y.; Sun, D. Impact of Amylose Content on Starch Retrogradation and Texture of Cooked Milled Rice during Storage. J. Cereal Sci. 2009, 50(2), 139–144. DOI: 10.1016/j.jcs.2009.04.003.
- Wolfe, K.; Wu, X.; Liu, R. H. Antioxidant Activity of Apple Peels. J. Agr. Food Chem. 2003, 51(3), 609–614. DOI: 10.1021/jf020782a.
- Tachibana, Y.; Kikuzaki, H.; Lajis, N. H.; Nakatani, N. Antioxidative Activity of Carbazoles from Murraya Koenigii Leaves. J. Agric. Food Chem. 2001, 49, 5589–5594. DOI: 10.1021/jf010621r.
- Suttiarporn, P.; Sookwong, P.; Mahatheeranont, S. Fractionation and Identification of Antioxidant Compounds from Bran of Thai Black Rice Cv. Riceberry. Int. J. Chem. Eng. Appl. 2016, 7, 109–114. DOI: 10.7763/IJCEA.2016.V7.552.
- Sivamaruthi, B. S.; Kesika, P.; Chaiyasut, C. Anthocyanins in Thai Rice Varieties: Distribution and Pharmacological Significance. Int. Food Res. J. 2018, 255, 2024–2032. DOI:10.5772/intechopen.86962.
- Moongngarm, A.; Daomukda, N.; Khumpika, S. Chemical Compositions, Phytochemicals, and Antioxidant Capacity of Rice Bran, Rice Bran Layer, and Rice Germ. APCBEE procedia. 2012, 2, 73–79. DOI: 10.1016/j.apcbee.2012.06.014.
- Azarakhsh, N.; Osman, A.; Ghazali, H. M.; Tan, C. P.; Mohd, A. N. Optimization of Alginate and gellan-based Edible Coating Formulations for fresh-cut Pineapples. Int. Food Res. J. 2012, 19(1), 279–285.
- Loypimai, P.; Sittisuanjik, K.; Jundum, C. Impact of Soaking in Sodium Chloride Solution on Physicochemical Properties and Cooking Quality of Parboiled Glutinous Rice. Khon Kaen Agr. J. 2017, 45(4), 573–582.
- Li, C.; Luo, J. X.; Zhang, C. Q.; Yu, W. W. Causal Relations among Starch chain-length Distributions, short-term Retrogradation and Cooked Rice Texture. Food Hydrocoll. 2020, 108, 106064. DOI: 10.1016/j.foodhyd.2020.106064.
- Yang, M. D.; Chen, X.; Sun, Z. Y.; Li, G.; Liu, Z. D. Study on the Characteristics of Ohmic Heating of Rice. Food Sci. Technol. 2006, 8, 031.
- Wu, N. N.; Qiao, C. C.; Tian, X. H.; Tan, B.; Fang, Y. Retrogradation Inhibition of Rice Starch with Dietary Fiber from Extruded and Unextruded Rice Bran. Food Hydrocoll. 2021, 113, 106488. DOI: 10.1016/j.foodhyd.2020.106488.
- Moritaka, H.; Nishinari, K.; Nakahama, N.; Fukuba, H. Effects of Potassium Chloride and Sodium Chloride on the Thermal Properties of Gellan Gum Gels. Biosc.i Biotech. Biochem. 1992, 564, 595–599. DOI:10.1271/bbb.56.595.
- Wang, G.; Hong, Y.; Gu, Z. Effect of NaCl Addition on the freeze-thaw Stability of Tapioca Starch Gels. Starch. 2015, 67, 604–611. DOI: 10.1002/star.201500048.
- Huang, Y.; Bao, X.; Li, P.; Zhan, L.; Wu, H.; Chen, P. Effect of NaCl Addition on alcohol-alkali-treated Waxy Rice Starch: Structural and Physicochemical Functionality. Food Chem. 2022, 389, 133021. DOI: 10.1016/j.foodchem.2022.133021.
- Isnaini, C.; Pathomrungsiyounggul, P.; Konsue, N. Effect of Preparation Method on Chemical Property of Different Thai Rice Variety [Sic]. J. Food Nutr. Res. 2019, 7(3), 231–236. DOI: 10.12691/jfnr-7-3-8.
- Kamjijam, B.; Bednarz, H.; Suwannaporn, P.; Na-Jom, K.; Niehaus, K. Localization of Amino Acids in Germinated Rice Grain: Gamma-aminobutyric Acid and Essential Amino Acids Production Approach. J. Cereal Sci. 2020, 93, 102958. DOI. DOI: 10.1016/j.jcs.2020.102958.
- Yu, S.; Ma, Y.; Liu, T.; Menager, L.; Sun, D. Impact of Cooling Rates on the Staling Behavior of Cooked Rice during Storage. J. Food Eng. 2010, 96, 416–420. DOI: 10.1016/j.jfoodeng.2009.08.019.
- Waewkum, P.; Singthong, J. Functional Properties and Bioactive Compounds of Pigmented Brown Rice Flour. Bioact. Carbohydr. Diet. Fibre. 2021, 26, 100289. DOI: 10.1016/j.bcdf.2021.100289.
- González-Aguilar, G.; Robles-Sánchez, R. M.; Martínez-Téllez, M. A.; Olivas, G. I.; Alvarez-Parrilla, E.; de la Rosa, L. A. Bioactive Compounds in Fruits: Health Benefits and Effect of Storage Conditions. Stewart Postharvest Rev. 2008, 3, 8. DOI: 10.2212/spr.2008.3.8.
- Rukmanikrishnan, B.; Ramalingam, S.; Rajasekharan, S. K.; Lee, J. Binary and Ternary Sustainable Composites of Gellan Gum, Hydroxyethyl Cellulose and Lignin for Food Packaging Applications: Biocompatibility, Antioxidant Activity, UV and Water Barrier Properties. Int. J. Biol. Macromol. 2020, 153, 55–62. DOI: 10.1016/j.ijbiomac.2020.03.016.
- Moreira, M. R; Tomadoni, B.;.; Martín-Belloso, O.;.; Soliva-Fortuny, R. Preservation of fresh-cut Apple Quality Attributes by Pulsed Light in Combination with Gellan gum-based Prebiotic Edible Coatings. LWT-Food Sci. Technol. 2015, 64, 1130–1137. DOI: 10.1016/j.lwt.2015.07.002.
- Robles-Sanchez, R.; Rojas-Graü, A.; Odriozola-Serrano, I.; Gonzalez-Aguilar, G.; Martín-Belloso, O. Influence of alginate-based Edible Coating as Carrier of Antibrowning Agents on Bioactive Compounds and Antioxidant Activity in fresh-cut Kent Mangoes LWT-Food. Sci.Technol. 2013, 50, 240–246. DOI: 10.1016/j.lwt.2012.05.021.
- Bhawamai, S.; Lin, S. H.; Hou, Y. Y.; Chen, Y. H. Thermal Cooking Changes the Profile of Phenolic Compounds, but Does Not Attenuate the Anti‐inflammatory Activities of Black Rice. Food Nutr. Res. 2016, 60, 32941. DOI: 10.3402/fnr.v60.32941.
- Surh, J; Koh, E. Effects of Four Different Cooking Methods on Anthocyanins, Total Phenolics and Antioxidant Activity of Black Rice. J. Sci. Food Agric. 2014, 94(15), 3296–3304. DOI: 10.1002/jsfa.6690.
- Hui, Y. H.; Sherkat, F. Functional Properties of Carbohydrates: Polysaccharide Gums; Boca Raton: Handbook of Food Science, Technology and Engineering. CRC Press, 2005; pp 13.