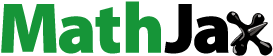
ABSTRACT
Melicope pteleifolia is a traditional Chinese medicinal plant that is thought to be developed as a biological insecticide. In this work, essential oils (EOs) of M. pteleifolia (collected in 2021 and 2022) over different extraction time (1 h, 2 h, 5 h) were investigated for their chemical composition and insecticidal activities. The EOs extracted from fresh leaves in 2021 were named A1h, A2h and A5h, and extracted from fresh leaves in 2022 were named B1h, B2h and B5h. The chemical components of EOs were analyzed by GC-MS. α-Thujene is the common primary compound of the EOs A1h, A2h and A5h, which contained 83.27%, 63.86% and 25.17%, respectively. Principal component analysis (PCA) revealed that sesquiterpenes were the primary representative components in EOs. The EOs that were extracted for 5 h were significantly different from other EOs. Moreover, the EOs and α-thujene showed obvious contact and repellent activities against Lasioderma serricorne and Liposcelis bostrychophila. The gray correlation degree explained that EOs A2h and A5h had the strongest repellency against L. serricorne, while EOs A1h and B1h had the most significant repellent effect on L. bostrychophila, with coefficients above 0.50. The comprehensive score of weighting coefficient revealed that the scores of EOs A1h and A5h were the highest and both reached 0.80. In general, M. pteleifolia have the potential to be exploited as biological insecticide.
Introduction
Booklice are members of the genus Liposcelis and are in the family Liposcelididae. L. bostrychophila is one of the most common species in the booklouse population and is particularly prone to destroying food stored at low oxygen and temperatures.[Citation1] L. bostrychophila has caused serious damage to crops in Canada, Australia, the United States and many other regions.[Citation2] There is already evidence that wheat (Triticum aestivum) seeds damaged by L. bostrychophila have difficulty germinating, which substantially affects crop yields. Moreover, L. bostrychophila can also pose a threat to human health by spreading fungal pathogens and causing allergic reactions.[Citation3]
The cigarette beetle (L. serricorne) is a widely eaten pest that destroys a variety of crops, such as tobacco (Nicotiana tabacum), tea (Camellia sinensis), grains, and beans.[Citation4] Studies showed that L. serricorne caused losses to the seeds of fennel (Foeniculum vulgare) and dill (Anethum graveolens) under different storage conditions, with losses of 58.02% and 39.0%, respectively.[Citation5] Yams (Dioscorea alata) lose 25% to 60% from the field to the market owing to the destruction by L. serricorne in India.[Citation6]Furthermore, the date (Phoenix dactylifera) is one of the most valuable fruits, and its market value is reduced owing to infestation by L. serricorne.[Citation7] . Hence, there is an urgent need to control these storage pests.
Currently, chemical insecticides are commonly used for pest control. However, the improper use of chemical pesticides can cause serious results. Chemical pesticides have been demonstrated to produce pesticide residues, which can degrade the quality of crops.[Citation8] In addition, only 0.1% of the chemical pesticides used were effective in controlling the target pests, while the rest of the pesticides contaminated the soil, water, and air.[Citation9] Moreover, human exposure to chemical pesticides can increase the risk of asthma, cancer and other diseases.[Citation10]
Plant-based biological insecticides are an intensive area of research. A comparison of biological and chemical insecticides indicated that the protein content of crops treated with chemical insecticides decreased, whereas the biological insecticides had no significant effect on the crops.[Citation11] Since the raw materials of biological insecticides are non-toxic natural plants, they are not harmful to human health.[Citation12] Besides, biological insecticides are species-selective and only have toxic effects on their target pests, which is highly significant for the protection of species in nature.[Citation13,Citation14]
Melicope pteleifolia (Champion ex Bentham) T. G. Hartley is a medicinal plant with substantial potential, which is characterized by bitter in flavor and cold in nature, attributed to lung and liver meridians, and has analgesic, anti-inflammatory, antipyretic, and other effects.[Citation15] M. pteleifolia is rich in chemical components, such as EO, alkaloids, and flavonoids.[Citation16] To fully utilize its resources as a medicinal plant, the EO of M. pteleifolia was tested for their activity against storage pests.
In this experiment, we collected fresh leaves of M. pteleifolia in two years (2021, 2022), and extracted the EOs at 1 h, 2 h, and 5 h, respectively. The six EOs were tested for their effectiveness at repelling infestations and insecticidal ability against L. serricorne and L. bostrychophila. This enabled us to conclude that the fresh leaves of M. pteleifolia have the potential to be exploited as biological insecticides.
Materials and methods
Plant materials and extraction of EOs
Fresh leaves of M. pteleifolia were collected in Pu’er, Yunnan, China (altitude 1,305 m, 22°46’ N, 100°58’ E) in December 2021 and August 2022. After identification, the samples were flown to the laboratory. The fresh leaf samples were maintained at the College of Resources Science and Technology, Faculty of Geographical Science, Beijing Normal University (Beijing, China), and the voucher specimen numbers were BNU-DSS-202112 and BNU-DSS-202208, respectively.
A Clevenger-type device was used to extract the EOs from fresh leaves of M. pteleifolia by hydrodistillation. The fresh leaves were dried naturally and crushed, and 1,250 g was weighed and placed in a round-bottom flask. Distilled water was added at a ratio of 1:10 (w/v). The experiment was initiated once the distilled water had started to boil, and the EOs were collected at 1 h, 2 h, and 5 h. The EOs were stored at 4°C after they had been dehydrated by anhydrous sodium sulfate. Each EO was extracted in triplicate, and the average yield was estimated.
Chemicals
n-Hexane (97%) was purchased from Beijing Chemical Works (Beijing, China). Fluon was purchased from Beijing Sino-Rich Material Science (Beijing, China). α-Thujene (98%) was purchased from Beijing InnoChem Science & Technology Co., Ltd. (Beijing, China).
Insect rearing
L. serricorne and L. bostrychophila were selected as target pests in this experiment. A total of 45 to 50 healthy and active adults of L. serricorne were transferred to glass jars (200 mL) that contained flour and yeast at ratio of 10:1 (w/w). In addition, four pieces of filter paper with a diameter of 1 cm were placed into a triangular flask that contained a large number of L. bostrychophila adults. After 30–35 adults had climbed onto each piece of filter paper, they were transferred to Erlenmeyer flasks (100 mL) that contained flour, yeast, and milk powder at a ratio of 10:1:1 (w/w/w). Both target pests were kept in the dark at 28–30°C and 70–80% relative humidity. One-week-old adults were tested for their insecticidal activity.
GC-MS analysis
The chemical compositions of EOs were analyzed using an Agilent 6890 N gas chromatograph coupled with an Agilent 5973 N mass selective detector to conduct gas chromatography-mass spectrometry (GC-MS) (Agilent Technologies, Santa Clara, CA, USA). A DB-5 MS quartz capillary column was used. The chromatographic conditions were as follows: the inlet temperature was 250°C; the split ratio was 1:20, and 1 μL of 1% sample solution (solvent: n-hexane) was injected. The carrier gas was helium at a constant flow rate of 1.0 mL/min by autoinjection. The column was maintained at 50°C for 3 min and then increased to 290°C at 10°C/min for 5 min. The mass spectrometry conditions were as follows: in the full scanning mode, electron-impact source analysis (EI) was used, with an electron energy of 70 eV, a temperature of 200°C for the electron source and 250°C for the transmission line, and a scanning range of 45–650 m/z. Homologous n-alkanes (C5-C25) were injected under the same conditions, and the retention index (RI) was calculated. The RI values were compared with those of NIST 17 (Standard Reference Data, Gaithersburg, MD, USA) and chemical standards. Representative compounds were quantified using external standards and by a calibration curve that was generated from the GC analysis.
Fumigant toxicity
The fumigation test steps of L. serricorne were as follows: 10 unsexed adults were randomly selected and transferred to glass vials (diameter 2.5 cm, height 5.5 cm) that were coated with Teflon. A circular filter paper with a diameter of 2 cm was attached to the inside of the bottle cap and 10 μL of the prepared sample solution was injected. The bottle cap was tightened and then sealed with Parafilm.[Citation17] The positive and negative controls were phosphine and n-hexane, respectively.[Citation18] L. bostrychophila was done according to the method described by Zhao et al. .[Citation19] Briefly, 10 adults were randomly selected and transferred to small glass bottles (8 mL), which were transferred to large glass jars (250 mL). A 5 cm long and 1 cm wide strip of filter paper strip was attached to the inside of large glass jar cap, and 10 μL of the prepared sample solution was injected into the front end. The bottle cap was capped with a matching glass jar and then sealed with Parafilm. The positive and negative controls were dichlorvos and n-hexane, respectively.[Citation20]
The concentrations of samples in these experiments were evaluated by preliminary results that tested L. serricorne at concentrations of 2%, 10% and 50%. L. bostrychophila was tested at concentrations of 0.2%, 1%, and 5%. The formal test concentrations are shown in . There were five replicates for each concentration. All the treated pests were kept in the dark at 32°C with a relative humidity of 70% to 80%, and the mortality was calculated after 24 h.
Table 1. Five specific concentrations of different samples for formal experiments against L. serricorne and L. bostrychophila.
Contact toxicity
L. serricorne contact methods were followed by the methods described by Liu and Ho.[Citation17] 10 one-week-old adults were randomly selected and placed in petri dishes. A volume of 0.5 μL of the sample solution was injected on their dorsal area, and the treated pests were transferred to glass bottles (diameter 2.5 cm, height 5.5 cm). For L. bostrychophila, a circular piece of filter paper with a diameter of 5.5 cm was filled with 300 μL of sample solution until it became saturated. After drying, the treated filter paper was attached to the inside of the Petri dish. Ten adults were randomly selected and released in the center of the Petri dish.[Citation19]
The concentrations of L. serricorne and L. bostrychophila in the preliminary experiments were 2%, 10%, and 50%, 0.2%, 1%, and 5%, respectively. The range of concentrations was determined based on the results of preliminary experiment and repeated five times. The formal test concentrations are shown in . The positive and negative controls for both pests were pyrethrins and n-hexane, respectively.[Citation18,Citation21] All the treated pests were kept at 32°C in a dark incubator with relative humidity of 70–80%, and mortality was calculated after 24 h.
Repellent effect
The test concentrations for L. serricorne were 78.63, 15.83, 3.15, 0.63, and 0.13 nL/cm,[Citation2] respectively. A circular filter paper with a diameter of 9 cm was cut into two symmetrical parts, and 500 μL of n-hexane and sample solution were injected until the paper was saturated. After natural drying, the corresponding two parts were pasted on the inside of the same petri dish (diameter 9 cm). A total of 20 adults were then released into the center of the petri dish.[Citation17] The difference was that a circular filter paper with diameter of 5.5 cm was used in the repellent experiment of L. bostrychophila, and 150 μL n-hexane and sample solution (63.17, 12.63, 2.53, 0.51, and 0.10 nL/cm[Citation2]) were injected into the corresponding filter paper.[Citation19] The repellent rates (PR) were estimated at 2 and 4 h. The positive control was DEET.
Statistical analysis
The components that were present at levels greater than 2% in the EO from six segments of M. pteleifolia fresh leaves were screened for statistical analysis. The FactoMineR and ExtrafActor packages in R (4.1.3) were used for principal component analysis (PCA) to analyze and visualize the chemical characteristics of EOs.[Citation22] The LC50/LC90 and LD50/LD90 of the fumigation and contact of EOs against two pests were evaluated using Probit (SPSS 22.0, IBM, Inc., Armonk, NY, USA). Confidence intervals (CI) of 95% and chi-square values were calculated.[Citation23] The repellent effect is displayed by calculating the repellent rate (PR), which was calculated using the following formula:
where Nc is the number of insects in the negative control, and Nt is the number of insects in the treatment. The PR values were converted to arcsine square root values, and a one-way analysis of variance and Tukey test were performed to compare the differences between means.[Citation24] P < .05 was used to indicate significant differences between the repellent means. The repellent activities of EOs against the target pests were calculated using the grey correlation analysis method in R (4.1.3).[Citation25] The weighted coefficient comprehensive scoring method was introduced.[Citation26] Taking the EOs yield, contact activity and repellent activity (relative correlation degree) of EOs as indices, the weight of oils yield was 0.2, and the total weight of insect resistance activity was 0.8. The two activities of the two target pests were respectively assigned weights of 0.2, and the quality of each EO was reflected by the comprehensive score.
Results
Chemical compositions of the EOs
shows the composition of EOs extracted from the fresh leaves of M. pteleifolia at different periods in 2021 and 2022. The average yields of EOs extracted for 1 h, 2 h and 5 h were 0.22%, 0.08%, and 0.09% (2021); 0.06%, 0.03% and 0.07% (2022), respectively.
Table 2. Constituents identified in the different periods EOs from leaves of M. pteleifolia.
Our chromatographic analysis revealed that EOs A1h, A2h and B1h were mainly composed of monoterpenes, which contented 92.74%, 72.01% and 55.26%, respectively. The primary components of the EOs A5h, B2h and B5h were 57.62%, 52.80% and 83.76% of sesquiterpene hydrocarbons, respectively. α-Thujene was the primary compound in the EOs A1h, A2h and A5h, and they contained 83.27%, 63.86% and 25.17%, respectively. The primary compound of EO B1h was 38.95% of ψ-limonene. Moreover, the primary compounds of EOs B2h and B5h were β-sesquisabinene (19.24%) and β-acoradiene (33.19%), respectively.
illustrates the results of PCA for EOs. PC1 and PC2 represented 28.97% and 27.53% of the EOs, respectively. Sesquiterpenes are the primary representative components of most EOs. The EOs A1h, A2h, B1h and B2h responded positively to PC1, while EOs A1h and B1h responded negatively to PC2. The EOs A5h and B5h responded negatively to PC1, while EO B5h responded negatively to PC2. The components of EOs A5h and B5h were significantly different from those of the other EOs. The variations in chemical composition of EOs may be attributed to the long duration of heating during the extraction.
Figure 1. The plot of PCA of different periods EOs of leaves from M. pteleifolia (the serial numbers of compounds are the same as ).

Fumigant toxicity
demonstrates the fumigation effects of six EOs extracted from M. pteleifolia against L. serricorne and L. bostrychophila. Only the primary compound α-thujene and EO A1h, A2h displayed fumigant remarkable toxicity against L. serricorne. The fumigation effect of α-thujene was the most obvious, and the value of LC50 was 50.25 mg/L air. The LC50 value was 52.18 mg/L air at EO A1h, and the LC50 value of EO A2h was 158.59 mg/L air. However, fumigation with 50% of the samples did not affect L. bostrychophila.
Table 3. Fumigant toxicity of EOs and major compound against L. serricorne and L. bostrychophila.
Contact toxicity
displays the contact toxicity of EOs extracted from the fresh leaves of M. pteleifolia over six different periods in 2021 and 2022 against the two target pests. EO A5h had the strongest contact effect against L. serricorne (LD50 = 20.75 μg/adult). The next most effective EO was EO B1h (LD50 = 21.66 μg/adult). The contact effect of EO B2h was the weakest compared with that of the other EOs (LD50 = 39.80 μg/adult). The contact effect of EO B2h against L. bostrychophila was the most apparent, and the LD50 value was 90.69 μg/adult. The LD50 values of the EOs B5h and B1h were 97.36 μg/adult and 101.05 μg/adult, respectively. EO A1h had the weakest contact effect on L. bostrychophila (LD50 = 207.73 μg/adult).
Table 4. Contact toxicity of EOs and major compound against L. serricorne and L. bostrychophila.
Repellent effect
The repellent effects of the six EOs extracted from M. pteleifolia against the two target pests are shown in . The EOs varied in their ability to repel the pests. In general, the repellent effect of samples against L. bostrychophila was more apparent, and all the samples were repelled by more than 80% at the highest concentration (78.63 nL/cm[Citation2]) after 2 h. However, the repellent effect of α-thujene decreased significantly after 4 h. Moreover, the repellent effect of EOs B1h and B2h exceeded that of the positive control. The EOs A2h, A5h and B2h were obvious effective at repelling L. serricorne at the highest concentration (78.63 nL/cm[Citation2]), and the repellent effect of EOs A2h and A5h was more apparent than that of the positive control after 2 h. However, the repellent effect was reduced to varying degrees after exposure of the target pest to the samples for 4 h. The repellent effect of the samples against both target pests decreased in parallel with the concentration.
Figure 2. (a) and (b) PR of EOs and α-Thujene against L. serricorne at 2 and 4 h post-exposure. (c) and (d) PR of EOs and α-Thujene against L. bostrychophila at 2 and 4 h post-exposure.

A grey correlation analysis was used to calculate the relative degree of correlation of the repellent activities of EOs and DEET against the two target pests, and the results are shown in . The relative correlation degree of EOs of A2h and A5h against L. serricorne was higher than 0.51, and the effect of EO A2h was the strongest with a relative correlation degree of 0.53. The repellent effect of EO B2h on L. serricorne was the weakest, and the relative correlation degree was only 0.43. The repellent activity of EO B1h was the strongest, with a relative correlation degree of 0.51. However, the repellent effect of EO A2h was the weakest, with a relative correlation degree of only 0.40. The relative correlation degree of the repellent effect of all the EOs was not higher than that of DEET, which indicated that the repellent activity of EOs was weaker than that of DEET.
Table 5. Grey correlation of repellent activities of EOs against L. serricorne and L. bostrychophila.
Comprehensive weighted assessment of EOs
shows the comprehensive score based on EOs yield, contact activity and repellent activity against the two target pests. The yield score of EO A5h was the highest, which reached 0.08. In the contact activity score, except for the EO A1h, all the EOs reached above 0.30, among which the EO B1h had the highest score, reaching 0.37. The repellent activity scores of EOs against the target pests were all above 0.30, and the best repellent effect was A1h with a score of 0.39. According to the comprehensive score, the highest score was EO of A1h, which reached 0.81, indicating that this EO was superior to the other EOs in terms of yield, contact activity and repellent activity.
Table 6. Weighted composite score for EOs yield, contact and repellent activities against L. serricorne and L. bostrychophila.
Discussion
This experiment studied the chemical composition of EOs extracted from the fresh leaves of M. pteleifolia collected at different periods (1 h, 2 h, and 5 h) in 2021 and 2022 and evaluated the insecticidal activity against two target pests. The EOs were primarily composed of monoterpenes and sesquiterpenes. The solution extracted from the leaves of M. pteleifolia with chloroform and methanol contained coumarins, flavonoids, alkaloids, and sesquiterpenes.[Citation27] The difference between the results of the two studies could be owing to the different polarity of solvents selected for extraction. A mixture of chloroform and methanol as solvents is more polar, which facilitates the dissolution of compounds. Simultaneously, the difference in the extraction time of EOs affects the chemical composition. The content of major compound α-thujene in EOs of A1h, A2h and A5h decreased with the increase of extraction time and the major components of B1h, B2h and B5h were different. Thus, the biological activity of EOs were changed. The location and season of plant collection are also the reasons for the difference of EOs composition. 46 compounds were found in the EO extracted from leaves of M. pteleifolia collected in Guangzhou, China, and the primary components included palmitic acid (30.74%), dibutyl phthalate (15.87%) and phytol (13.46%).[Citation28] Moreover, the EO of leaves from M. pteleifolia collected from Hainan, China was mainly composed of oxygen-containing organic compounds and hydrocarbons (52% and 29%, respectively), which α-humulene was the major compound.[Citation29] These findings revealed significant differences in the chemical composition of EOs from plant collected from different regions. In this study, the primary components of the leaves of M. pteleifolia collected in December 2021 and August 2022 differed. According to the weighted comprehensive score, the EOs extracted from the fresh leaves collected in 2021 were better than those extracted from the leaves collected in 2022. Owing to the changes of temperature, precipitation, and air pressure at different collection times, the types and contents of the secondary metabolites of plants were correspondingly different, resulting in the difference of insecticidal activities among EOs.[Citation30] The chemical composition of Mesosphaerum suaveolens harvested in three different seasons also differed. Although the primary component was 1,8-cineole, the composition also clearly differed (30.15%, 64.44% and 46.31%).[Citation24] In addition, it was also confirmed that collecting the EOs of Tanacetum perthenium and Pycnocycla spinosa at different seasons affected the composition of their EOs.[Citation31,Citation32]
α-Thujene was the primary component of leaf EOs from M. pteleifolia collected in 2021, which is the focus of this study. α-Thujene was also found in Tagetes lacera, Baccharis tridentate, and Campomanesia adamantium at concentrations of 20.5%, 22.93% and 8.86%, respectively.[Citation33–35] However, as a common compound, its application has not been fully developed, and to our knowledge, this work is the first to investigate its insecticidal activity against storage pests. In the fumigation test, α-thujene was more effective against L. serricorne than the EOs, and the fumigation effect of EOs improved with the increase in content of α-thujene. In contrast, there was no such pattern in the contact test. The repellent activity of EOs in 2021 was stronger than that in 2022, and increased with the decrease of α-thujene content. This may be attributed to the fact that the primary compounds in the EOs in 2022 are different from those in 2021, leading to changes in repellent activity. Also, there may be synergetic or antagonistic interactions between the components in EOs and causes changes in biological activity. The primary compounds in the five plant EOs were tested for their insecticidal activities, and it was proven that the mixture of limonene with diallyldisulfide would produce synergistic effects, while the combination of limonene with α-pinene or eucalyptol would produce antagonistic effects.[Citation36] A study of the ability of primary EOs of Carum copticum to control the tomato leaf miner (Tuta absoluta) clarified that combining thymol and p-cymene increased their toxicity, whereas mixing ɤ-terpinene and p-cymene produced antagonistic effects.[Citation37] The interaction between compounds in the EOs of the fresh leaves of M. pteleifolia merits further study.
In the fumigation test of this experiment, only the EOs A1h, A2h, and the primary compound α-thujene were toxic to L. serricorne, and the insecticidal activity of contact was more apparent than that of fumigation. Similarly, the EOs of southern cone marigold (Tagetes minuta), silver mint (Mentha longifolia), rosemary (Rosmarinus officinalis), Helichrysum odoratissimum and rose-scented Geranium (Pelargonium graveolens) tested for pest control were more insecticidal by contact than fumigation; the activities of EOs were dose-dependent, and the insect mortality increased in parallel with the concentration of EOs.[Citation38] Thus, different modes of action will lead to substantial differences in insecticidal activity. However, the mechanism by which different modes of action produce different pest control effects merits further study.
In addition to the insecticidal effects against L. serricorne and L. bostrychophila, M. pteleifolia also inhibited the oviposition activity of Trichophysetis cretacea by 93.40%.[Citation39] The toxic effect of insecticides and their compounds on storage pests is due to the inhibition of carboxylesterase (CarE) and acetylcholinesterase (AChE) activities. The primary compound R-(+) pulegone in lemon grass (Cymbopogon citratus) EO inhibited the activity of AChE in L. serricorne, and the degree of inhibition changed with concentration.[Citation40] The mechanism of action of insecticide against L. bostrychophila was confirmed to be the inhibition of CarE and AChE.[Citation41,Citation42]
The EOs from M. pteleifolia were proven to have insecticidal effects on storage pests. In this study, the plant samples were inexpensive (1.68 USD per kilogram), and the price is appropriate after the products have been developed into commodities. The composition of EOs is unstable, volatile, and metamorphic, which could affect the insecticidal effects. However, this problem can be solved by creating nanoemulsions based on the EOs. The insecticidal effect of the EO from citronella grass (Cymbopogon nardus) was compared with that of a nanoemulsion, and the nanoemulsion was found to be more toxic, which could be related to its enhanced stability.[Citation43] Cassava (Manihot esculenta) starch was mixed with the EO from Siparuna guianensis for microencapsulation, which provided further evidence that the microencapsulated EO was clearly more insecticidal and solved the problem of the instability of the EO components.[Citation44] Overall, the EOs from M. pteleifolia have the potential to be developed as commercial insecticides.
Conclusion
Fragmented EOs from the fresh leaves of M. pteleifolia were investigated for their chemical composition and insecticidal activity. There were significant differences in the major components of EOs between 2021 and 2022. α-Thujene is the major component of EOs A1h, A2h and A5h, containing 83.27%, 63.86% and 25.17%, respectively. The biological activity of EOs were changed due to the difference of primary components in EOs. Moreover, The EOs and primary compound α-thujene had significant contact and repellent effects against L. serricorne and L. bostrychophila. The overall effect of EOs extracted from the leaves collected in December 2021 was highly toxic than that of fresh leaves collected in August 2022. M. pteleifolia has prospects for development as a biological insecticide product owing to its strong insecticidal properties, low price, and simple preparation process.
Author contributions
Conceptualisation, B.-Y.L.; Data curation, Q.-H.X.; Formal analysis, B.-Y.L. and S.X.; Investigation, J.-W.Z.; Methodology, Y.Z.; Resources, S.-S.D.; Supervision, D.W. and S.-S.D.; Validation, B.-Y.L.; Visualisation, Q.-H.X.; Writing—original draft, B.-Y.L.; Writing—review and editing, J.-W.Z. All authors have read and agreed to the published version of the manuscript.
Disclosure statement
No potential conflict of interest was reported by the author(s).
Additional information
Funding
References
- Cheng, W.-X. Research Progress in the Resistance and Control Method to Liposcelis bostrychophila and Liposcelis entomophila. J. AGR. 2008, 36, 6376–6379.
- Diaz-Montano, J.; Campbell, J. F.; Phillips, T. W.; Throne, J. E. Evaluation of Potential Attractants for Liposcelis bostrychophila (Psocoptera: Liposcelididae). J. Econ. Entomol. 2014, 107(2), 867–874. DOI: 10.1603/ec13427.
- Gautam, S. G.; Opit, G. P.; Giles, K. L.; Weight Loss, A. B. Germination Failure Caused by Psocids in Different Wheat Varieties. J. Econ. Entomol. 2013, 106(1), 491–498. DOI: 10.1603/ec12253.
- Miyatake, T.; Yokoi, T.; Fuchikawa, T.; Korehisa, N.; Kamura, T.; Nanba, K.; Ryouji, S.; Kamioka, N.; Hironaka, M.; Osada, M., et al. Monitoring and Detecting the Cigarette Beetle (Coleoptera: Anobiidae) Using Ultraviolet (LED) Direct and Reflected Lights and/or Pheromone Traps in A Laboratory and A Storehouse. J. Econ. Entomol. 2016, 109(6), 2551–2560. DOI: 10.1093/jee/tow225.
- Kant, K.; Ranjan, J. K.; Mishra, B. K.; Meena, S. R.; Lal, G.; Vishal, M. K. Post Harvest Storage Losses by Cigarette Beetle (Lasioderma serricome Fab.) in Seed Spice Crops. Indian J. Hortic. 2013, Vol. 70, 392–396.
- Vernier, P.; Goergen, G.; Dossou, R.; Letourmy, P.; Chaume, J. Utilization of Biological Insecticides for the Protection of Stored Yam Chips. Outlook Agric. 2005, 34(3), 173–179. DOI: 10.5367/000000005774378810.
- Mailafiya, D. M.; Bamaiyi, L. J.; Magaji, B. T.; Musa, I. P.; Kwanashie, A. J.; Banwo, O. O.; Bawa, L. Y. Pest Activity and Natural Enemy Diversity in Stored Dry Date (Phoenix dactylifera L. (Arecales: Arecaceae)) in the Northern Guinea Savannah Agroecological Zone. Int. J. Trop. Insect Sci. 2022, 42(1), 457–470. DOI: 10.1007/s42690-021-00562-z.
- Zhang, Y.-X.; Liu, Z.-W. Mechanisms of Insecticide Resistance and Selectivity. Bull. National Nat. Sci. Foundation of Chin. 2020, 34, 511–518.
- Devrnja, N.; Milutinovic, M.; Savic, J. When Scent Becomes a Weapon-plant Essential Oils as Potent Bioinsecticides. Sustainability. 2022, 14(11), 6847. DOI: 10.3390/su14116847.
- Alif, A. H. A.; Lokman, M. I. M.; Afzan, M. Y.; Siew, P. L.; Fakhuruddin, N. H. N.; Hussin, M. The Alarming Community Concern on Household Insecticide Exposure and Usage. Int. Medical J. Malaysia. 2019, 18, 133–138.
- Wang, H.-M.; Li, Y.-F.; Zhang, X.-H.; Li, H.; Zhao, J.-H. Effects of Pesticides on Antioxidant Enzyme Activities and Related Physiological Indices on Cucumber Seedlings. Chin. J. Eco-Agric. 2015, 23, 1185–1190.
- Aydin, C.; Mammadov, R. Plant Secondary Metabolites Demonstrating Insecticidal Activity and Effect Mechanism. Marmara Pharm. J. 2017, 21, 30–37. DOI: 10.12991/marupj.259878.
- Benelli, G.; Pavela, R.; Drenaggi, E.; Desneux, N.; Maggi, F. Phytol, (E)-Nerolidol and Spathulenol from Stevia rebaudiana Leaf Essential Oil as Effective and Eco-friendly Botanical Insecticides Against Metopolophium dirhodum. Ind. Crop Prod. 2020, 155, 112844. DOI: 10.1016/j.indcrop.2020.112844.
- Canto-Tejero, M.; Pascual-Villalobos, M. J.; Guirao, P. Aniseed Essential Oil Botanical Insecticides for the Management of the Currant-Lettuce Aphid. Ind. Crops Prod. 2022, 181, 114804. DOI: 10.1016/j.indcrop.2022.114804.
- Chen, C.-Y.; Huang, Y.-Q.; Wang, X.-P.; Han, -Z.-Z.; Xin, J.-Y.; Zhan, R.-T. Herbal Textual Research on Origin and Development of Sanchaku Melicope pteleifolia (Champ. ex Benth.)T. G. Hartley. Traditional Chinese Drug Research and Clinical Plarmacology. 2017, 28, 543–548.
- Liu, T.; Wang, S.; Wang, Y.; Tan, Y.; Chen, S. Research Progress in Melicope pteleifolia. Chin. Herb. Med. 2016, 47, 4103–4110.
- Liu, Z.-L.; Ho, S.-H. Bioactivity of the Essential Oil Extracted from Evodia rutaecarpa Hook f. et Thomas Against the Grain Storage Insects, Sitophilus zeamais Motsch and Tribolium castaneum (Herbst). J. Stored Prod. Res. 1999, 35(4), 317–328. DOI: 10.1016/S0022-474X(99)00015-6
- Yang, K.; Wang, C.-F.; You, C.-X.; Geng, Z.-F.; Sun, R.-Q.; Guo, -S.-S.; Du, -S.-S.; Liu, Z.-L.; Deng, Z.-W. Bioactivity of Essential Oil of Litsea cubeba from China and Its Main Compounds Against Two Stored Product Insects. J. Asia Pac. Entomol. 2014, 17(3), 459–466. DOI: 10.1016/j.aspen.2014.03.011.
- Zhao, -N.-N.; Zhou, L.-G.; Liu, Z.-L.; Du, -S.-S.; Deng, Z.-W. Evaluation of the Toxicity of the Essential Oils of Some Common Chinese Spices Against Liposcelis bostrychophila. Food Control. 2012, 26(2), 486–490. DOI: 10.1016/j.foodcont.2012.02.021.
- Liu, X.-C.; Li, Y.-P.; Li, H.-Q.; Deng, Z.-W.; Zhou, L.-G.; Liu, Z.-L.; Du, -S.-S. Identification of Repellent and Insecticidal Constituents of the Essential Oil of Artemisia rupestris L. Aerial Parts against Liposcelis bostrychophila Badonnel. Molecules. 2013, 18(9), 10733–10746. DOI: 10.3390/molecules180910733.
- You, C.-X.; Yang, K.; Wu, Y.; Zhang, W.-J.; Wang, Y.; Geng, Z.-F.; Chen, H.-P.; Jiang, H.-Y.; Du, -S.-S.; Deng, Z.-W., et al. Chemical Composition and Insecticidal Activities of the Essential Oil of Perilla frutescens (L.) Britt. Aerial Parts Against Two Stored Product Insects. Eur. Food Res. Technol. 2014, 239(3), 481–490. DOI: 10.1007/s00217-014-2242-8.
- Le, S.; Josse, J.; Husson, F. FactoMineR: An R Package for Multivariate Analysis. J. Stat. Softw. 2008, 25(1), 1–18. DOI: 10.18637/jss.v025.i01
- Probit, S. M. Analysis of Preference Data. Appl. Entomol. Zool. 1998, 33(3), 339–347. DOI: 10.1303/aez.33.339.
- Luz, T.; Leite, J. A. C.; de Mesquita, L. S. S.; Bezerra, S. A.; Silveira, D. P. B.; de Mesquita, J. W. C.; Ribeiro, E. C. G.; Vilanova, C. M.; Ribeiro, M. N. D.; Do Amaral, F. M. M., et al. Seasonal Variation in the Chemical Composition and Biological Activity of the Essential Oil of Mesosphaerum suaveolens (L.) Kuntze. Ind. Crop Prod. 2020, 153, 112600. DOI: 10.1016/j.indcrop.2020.112600.
- Wu, Z.; Su, -W.-W.; He, -X.-X. Study on Quality Evaluation of Forsythia suspensa with Grey Pattern Recognition. Chinese herbal midicine. 2000, 9, 536–538. DOI: 10.13863/j.1001-4454.2000.09.012.
- You, C.-X.; Zhang, Z.-Q.; Li, F.; Hou, X.-Z.; Determination, Y. C. Fingerprint Analysis of Eight Constituents in Puerarae Radix by HPLC Wavelength Switching. Chinese herbal midicine. 2013, 44, 616–621.
- Nakashima, K.; Abe, N.; Chang, F. R.; Inoue, M.; Pteleifolols A-E, O. M. Pteleifolols A–E, acetophenone di-C-glycosides and a benzopyran dimer from the leaves of Melicope pteleifolia. J. Nat. Med. 2017, 71(1), 299–304. DOI: 10.1007/s11418-016-1035-8.
- Diao, Y.-M.; Gao, Y.-H. 广东产三叉苦叶挥发性成分的气相色谱-质谱联用分析. Shi Zhen Chinese medicine. 2008, 151(3), 708.
- Bi, H.-P.; Han, C.-R.; Han, J.-P. 三叉苦叶挥发油的化学成分分析. Chin. Herb. Med. 2005, (5), 663–664.
- Prinsloo, G.; Nogemane, N. The Effects of Season and Water Availability on Chemical Composition, Secondary Metabolites and Biological Activity in Plants. Phytochem. Rev. 2018, 17(4), 889–902. DOI: 10.1007/s11101-018-9567-z.
- Bozovic, M.; Navarra, A.; Garzoli, S.; Pepi, F.; Ragno, R. Essential Oils Extraction: A 24-Hour Steam Distillation Systematic Methodology. Nat. Prod. Res. 2017, 31(20), 2387–2396. DOI: 10.1080/14786419.2017.1309534.
- Leon-Fernandez, M.; Sanchez-Govin, E.; Quijano-Celis, C. E.; Pino, J. A. Effect of Planting Practice and Harvest Time in Oil Content and its Composition in Melissa officinalis L. Cultivated in Cuba. J. Essent. Oil Bear. Pl. 2008, 11(1), 62–68. DOI: 10.1080/0972060x.2008.10643599.
- Diaz-Cedillo, F.; Serrato-Cruz, M. A.; Arce-Montoya, M.; Leon-de La Luz, J. L. Composition of Essential Oil of Tagetes lacera, Endemic Plant from Baja California Sur, Mexico. Rev. Mex. Biodivers. 2012, 83, 543–547.
- Sa, S.; Chaul, L. T.; Alves, V. F.; Fiuza, T. S.; Tresvenzol, L. M. F.; Vaz, B. G.; Ferri, P. H.; Borgese, L. L.; Paula, J. R. Phytochemistry and Antimicrobial Activity of Campomanesia adamantium. Rev. Bras. Farmacogn. 2018, 28(3), 303–311. DOI: 10.1016/j.bjp.2018.02.008.
- Souza, S. P.; Cardoso, M. G.; Souza, P. E.; Guimarães, L. G. L.; Andrade, J.; Mallet, A. C. T.; Nelson, D. L. Óleo essencial de Baccharis tridentata Vahl: composição química, atividade antioxidante e fungitóxica, e caracterização morfológica das estruturas secretoras por microscopia eletrônica de varredura. Revista Brasileira de Plantas Medicinais. 2011, 13(4), 456–466. DOI: 10.1590/s1516-05722011000400011.
- Sarma, R.; Adhikari, K.; Mahanta, S.; Khanikor, B. Combinations of Plant Essential Oil Based Terpene Compounds as Larvicidal and Adulticidal Agent Against Aedes aegypti (Diptera: Culicidae). Sci. Rep. 2019, 9(1), 9471. DOI: 10.1038/s41598-019-45908-3.
- Piri, A.; Sahebzadeh, N.; Zibaee, A.; Sendi, J. J.; Shamakhi, L.; Shahriari, M. Toxicity and Physiological Effects of Ajwain (Carum copticum, Apiaceae) Essential Oil and Its Major Constituents Against Tuta absoluta (Meyrick) (Lepidoptera: Gelechiidae). Chemosphere. 2020, 256, 127103. DOI: 10.1016/j.chemosphere.2020.127103.
- Odeyemi, O. O.; Masika, P.; Afolayan, A. J. Evaluation of the Activities of Five Essential Oils Against the Stored Maize Weevil. Nat. Prod. Commun. 2008, 3, 1097–1102.
- Wei, D.-W.; Zeng, T.; Li, L.-F.; Wang, Z.-Y.; Chen, H.-S.; Liu, Y. Studies on the Ovipositional Deterrent Activity of 24 Plant Extracts Against Trichophysetis cretacea. Plant Prot. 2006, 32, 38–40.
- Ramadan, G. R. M.; Abdelgaleil, S. A. M.; Shawir, M. S.; El-bakary, A. S.; Zhu, K. Y.; Phillips, T. W. Terpenoids, DEET and Short Chain Fatty Acids as Toxicants and Repellents for Rhyzopertha Dominica (coleoptera: Bostrichidae) and Lasioderma serricorne (Coleoptera: Ptinidae). J. Stored Prod. Res. 2020, 87(87), 101610. DOI: 10.1016/j.jspr.2020.101610.
- Cheng, W.-X.; Wang, -J.-J.; Ding, W.; Zhao, Z.-M. Inhibition Kinetics on Carboxylesterase and Acetylcholinesterase of Liposcelis bostrychophila and Liposcelis entomophila (Psocop., Liposcelididae) of Two Insecticides. J. Appl. Entomol. 2004, 128(4), 292–297. DOI: 10.1111/j.1439-0418.2004.00846.x.
- Ren, Y.; Wei, X.-Q.; Wu, S.; Dou, W.; Wang, -J.-J. Comparison of Acetylcholinesterase from Three Field Populations of Liposcelis paeta Pearman (Psocoptera: Liposcelididae): Implications of Insecticide Resistance. Pestic. Biochem. Physiol. 2008, 90(3), 196–202. DOI: 10.1016/j.pestbp.2007.11.008.
- Gharsan, F. N.; Kamel, W. M.; Alghamdi, T. S.; Alghamdi, A. A.; Althagafi, A. O.; Aljassim, F. J.; Al-Ghamdi, S. N. Toxicity of Citronella Essential Oil and Its Nanoemulsion Against the Sawtoothed Grain Beetle Oryzaephilus surinamensis (Coleoptera: Silvanidae). Ind. Crop Prod. 2022, 184, 115024. DOI: 10.1016/j.indcrop.2022.115024.
- Moura, W. S.; Oliveira, E. E.; Haddi, K.; Correa, R. F. T.; Piau, T. B.; Moura, D. S.; Santos, S. F.; Grisolia, C. K.; Ribeiro, B. M.; Aguiar, R. W. S. Cassava Starch-based Essential Oil Microparticles Preparations: Functionalities in Mosquito Control and Selectivity Against Non-target Organisms. Ind. Crops Prod. 2021, 162, 113289. DOI: 10.1016/j.indcrop.2021.113289.