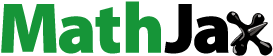
ABSTRACT
The medicinal use of Salsola tetragona Delile (Amaranthaceae) aerial parts is a longstanding tradition. This study delved into the plant’s potential as an antioxidant, anti-diabetic, and anti-Alzheimer agent. The aerial portion extracted and evaluated four fractions (n-hexane, dichloromethane, ethyl acetate, n-butanol) for their antioxidant activity using DPPH, FRAP, and anti-hemolysis tests, as well as the inhibitory activity of cholinesterase and carbohydrate digesting enzymes. The results showed that the dichloromethane fraction (St.DCM) of S. tetragona had a significant ability to scavenge DPPH• radicals. The ethyl acetate fraction (St.EtOAc) had the best reduction power test activity compared to other solvent fractions. The n-hexane fraction (St.Hex) was most effective in the anti-hemolysis test. The ethyl acetate fraction also had inhibitory activities (p < .05) with IC50 values of 70 ± 1.80 µg/ml for α-glycosidase, equivalent to the n-butanol fraction (St.n-BuOH), which had very significant activity (p < .05) in the α-amylase inhibition test with an IC50 of 64 ± 1.80 µg/ml. The ethyl acetate fraction also had a considerable concentration of total phenolic compounds and flavonoids and exhibited significant (p < .05) inhibitory effects on acetylcholinesterase with an IC50 of 30 ± 0.30 µg/ml. Therefore, the aerial parts of S. tetragona contained high levels of biologically active compounds, making it a potential source for developing new plant-based pharmaceuticals and nutraceuticals to enhance human health.
Introduction
Plants, particularly herbs, have been utilized in medicine and therapy for an extended period and in various forms as they serve as the primary source of medication. With the advancement of science, the therapeutic properties of plants have gained significant attention and importance worldwide. These properties include anti-inflammatory, anti-cancer, anti-malarial, anti-ulcer, anti-microbial, antioxidant effects, and various nutraceutical properties.[Citation1] Studies have focused on the beneficial roles of phytochemicals present in plants and their ability to promote overall health of the many secondary metabolites in plants, phenolics and flavonoids are particularly notable for their ability to provide these health benefits.[Citation2] New drugs have been developed using plant-based materials or plant precursors, making a comprehensive evaluation of plants crucial. As societies become increasingly focused on wellness and diet, natural plant products are considered viable alternatives to synthetic drugs. New plant-based products or Phytomedicine are deemed valuable resources that may replace synthetic medicines in light of these developments.
One such therapeutic plant includes Salsola tetragona Delile. This plant belongs to the genus Salsola (comprises over 120), family Amaranthaceae, which species found in arid and semi-arid regions of Asia, Europe, and Africa.[Citation3] This medicinal plant’s leaves and aerial parts have been traditionally used to treat various ailments, including indigestion, constipation, abdominal and gastric pain, hypertension, kidney disease, and diabetes.[Citation4] The powder or decoction of S. tetragona leaves can alleviate gastrointestinal pains, gastric pains, intestinal worms, microbial infections, cancer, and arrhythmia and can be used as a purgative.[Citation5] S. tetragona is commonly used as a treatment for diabetes, with the leaves, roots, bark, and fruit being used for this purpose, typically in powder form or as a decoction.[Citation6] These findings suggest that S. tetragona may have potential therapeutic effects against diabetes and other related diseases.
This investigation aimed to determine the characteristics of S. tetragona as anti-diabetic and anti-Alzheimer properties by assessing its inhibitory effects on enzymes (α-Amylase, α-Glucosidase, and Acetylcholinesterase) associated with these diseases. Additionally, this study aims to evaluate the antioxidant activity as well as the polyphenol and flavonoid content in four fractions of the aerial portion of S. tetragona: n-hexane (St.Hex), dichloromethane (St.DCM), ethyl acetate (St.DCM), and n-butanol (St.DCM). This study represents the initial examination of this plant, with the ultimate aim of contributing to the utilization of plants in the creation of novel medications and functional food ingredients to benefit human health.
Materials and methods
Chemicals and reagents
The chemicals and reagents used in this research are of analytical grade. n-hexane (Fisher Scientific), dichloromethane (Fischer Scientific), ethyl acetate (Fischer Scientific), and n-butanol (Fisher Scientific) were purchased from the local vendor Rouiba, Algeria. Folin – Ciocalteu phenol reagent (FCR; Sigma Aldrich), α-glucosidase, α-amylase, and acetylcholinesterase, Acarbose, and Galantamine (Sigma-Aldrich, St. Louis) were also purchased from Algeria, Algeria. Chemicals and reagents such as gallic acid, quercetin, ascorbic acid, 1,1-diphenyl-2-picrylhydrazyl (DPPH), Butylated hydroxyanisole (BHA), α-Tocopherol and Na2CO3, AlCl3, C2H3O, Al (NO3)3, FeCl3, and K3[Fe(CN)6 were of analytical grade (Sigma Aldrich, St. Louis) and purchased from Algeria, Algeria.
Plant material collection
S. tetragona aerial portion was collected on May 15th, 2020, from the El-Magrane region (El-Oued, southeast Algeria, 33°48‘39“N 6°55’19.5” E) under the supervision of Dr Youcef Halis, Director of the Technical Research Center on Arid Regions, Algeria. A voucher specimen (LOST.St07/09) was deposited at the herbarium of the Faculty of Life and Natural Sciences, El-Oued University. The specimen was meticulously cleaned and underwent a process of desiccation at ambient temperature for 20 days, under conditions that ensured protection from moisture, light exposure, dust, and other contaminants. Adequate ventilation was maintained throughout the process. Upon completion of the drying process, the specimen was pulverized and stored in an airtight glass container to preserve its integrity.
Preparation of the extract and fractionation of plant material
The dried powder of S. tetragona (200 g) was subjected to maceration at ambient temperature utilizing a solvent mixture of MeOH: H2O (70:30, V/V) at a volume of 600 mL. This process was repeated thrice, with solvent regeneration at 24-hour intervals. Following filtration, the extract solutions were concentrated via rotary evaporation at a temperature not exceeding 40°C, and distilled water (800 mL) was added. The resulting solution was subsequently subjected to liquid-liquid extraction utilizing organic solvents of varying polarities, including n-hexane, dichloromethane, ethyl acetate, and n-butanol (3×600 mL for each solvent).
TPC (total phenolic contents)
The TPC of the samples was detected using the Folin-Ciocalteu reagent (FCR) and represented as µg of gallic acid equivalents.[Citation7] The principle of this assay is to produce the Molybdenum-tungsten blue by reducing the (FCR) in the presence of phenolics compounds. The phenolic amount was determined using the standard gallic acid graph (Abs = 0.006 µg gallic acid + 0.0007, r2 = 0.999).
TFC (total flavonoid contents)
The TFC of the samples was determined using a colorimetric method[Citation8] and represented as µg of quercetin equivalents. This method involved the reaction between aluminum nitrate and potassium acetate, which resulted in the formation of a yellow complex. The flavonoid amount was determined using the standard quercetin graph (Abs = 0.005 µg quercetin + 0.0402, r2 = 0.999).
In vitro antioxidant activity
DPPH Scavenging assay: The DPPH• radical scavenging activity of fractions was determined utilizing a modified protocol.[Citation9] 1 ml at varying concentrations of S. tetragona fractions was added to 1 ml of 0.1 × 10−3 M DPPH (methanol solution). At 517 nm, the absorbance was measured after the mixture was incubated for 15 min at ambient temperature. The following equation determined the percentage of inhibition (EquationEquation 1(1)
(1) ):
In the DPPH test, α-Tocopherol and BHA were employed as reference standards for comparison. The IC50 (µg/ml), or the lowest concentration capable of decreasing DPPH• radicals by 50%, was calculated using the calibration curve’s regression equation and used to express and compare the results.
Ferric reducing capacity: The reducing potential of S. tetragona fractions (St.Hex, St.DCM, St.EtOAc, St.n-BuOH) was evaluated by determining their ability to reduce FeCl3 solution.[Citation10] The same volume (2.5 mL) of each fraction and 200 mM PBS (phosphate buffer solution pH 6.6) and 1% K3[Fe(CN)6] were mixed and then incubated for 20 min at 50°C. After adding 2.5 mL of 10% TCA, the solution was centrifuged for 10 min at 650 rpm, and 5 mL of the filtrate was mixed with an equal volume of distilled water and 1 mL of 0.1% FeCl3. At 700 nm, the optical density was measured, and ascorbic acid was used as a standard. The results were compared to its EC50 value (effective concentration that gives an absorption of 0.5 at 700 nm).
Anti-hemolysis activity: The anti-hemolytic properties of the fractions were assessed in vitro using the protocol outlined by Afsar and others[Citation11] with certain modifications. The extract (2 ml) was incubated for 5 minutes at 37°C with 40 μl of a 10% human red blood cell suspension. Following this incubation time, the mixture was treated with 40 μL of 30 μM H2O2, 40 μL of 80 mM FeCl3, and 40 μL of 50 mM ascorbic acid and incubated for an additional hour at 37°C. The mixture was then centrifuged at 700 revolutions per minute for 5 minutes. The absorbance at 540 nm was measured, and the percentage of hemolysis was estimated using the following equation (EquationEquation 2(2)
(2) ):
In this experiment, ascorbic acid was used as the standard. The Hly50 value, which shows the concentration at which 50% of red blood cells were lysed, was used to express the results.
α-amylase inhibitory activity
α-amylase inhibitory activity was determined using the technique described by the Marmouzi study.[Citation12] This involved incubating various concentrations of S. tetragona extracts (25 μL) with the α-amylase enzyme in a phosphate buffer (50 μL) and starch solution (50 μL) at 37°C. After 10 minutes, the reaction was stopped by adding 25 μL of 1 M hydrochloric acid and 100 μL of IPI (iodine-potassium iodide) solution. Following that, the absorbance was measured at 630 nm. As a positive control, acarbose was used. The inhibitory % activity was calculated in the following way (EquationEquation 3(3)
(3) ):
The results were represented as IC50 values, which show the fraction concentration necessary to block 50% of enzyme activity.
α-glucosidase inhibitory activity
To determine the inhibitory capacity of α-glucosidase using S. tetragona extracts as the test sample. Methodologies established by Marmouzi[Citation12] were utilized, with p-nitrophenyl-α-D-glucopyranoside as the substrate and a 96-well microplate format. A mixture of 50 μL of the extract, 50 μL of glutathione, and 50 μL of α-glucosidase solution in phosphate buffer (pH 6.8), and PNPG (50 μL) was prepared and incubated for 15 minutes. The process was then stopped by adding 50 μL of 0.2 M Na2CO3, and the absorbance at 400 nm was measured. Acarbose was employed as a positive control. The inhibitory percentage activity was estimated using the equation below (EquationEquation 4(4)
(4) ):
Acetylcholinesterase inhibition activity
The capacity of S. tetragona fractions to inhibit acetylcholinesterase was analyzed through the method of Ellman et al.[Citation13] The procedure involved mixing 300 μL of 50 mM Tris-HCl buffer (pH 8), 30 μL of AChE solutions, and 100 μL of the sample. Following a 15-minute incubation, 130 μL of acetylthiocholine iodide and 440 μL of DTNB (3 mM) were added to end the reaction, and the absorbance was determined at 412 nm. Galantamine was used as the standard, and IC50 values were utilized to report the results. The inhibition potency was calculated using the equation below (EquationEquation 5(5)
(5) ):
A is AChE capacity in the absence of an inhibitor, B is AChE capacity in the presence of an inhibitor.
Statistical analysis
Quantitative estimates, enzyme inhibitory activity assays, and antioxidant ability were carried out in triplicates. The results obtained have been included as mean ± SD (standard deviation). One-way ANOVA with post hoc Tukey’s (HSD) test was used to assess statistical differences. And p-values <.05 were as significant.
Results and discussion
TPC and TFC in plant fractions
The results of the TPC (total phenolic content) of the S. tetragona fractions were quantified and expressed as gallic acid (GA) equivalents (presented in ). The data indicated that the ethyl acetate fraction (St.EtOA) exhibited the highest quantitative content (p < .05) of polyphenols at 52.76 ± 0.25 μg GAE/mg, followed by the dichloromethane fraction (St.DCM) with 45.65 ± 0.22 μg GAE/mg, the n-hexane fraction (St.Hex) with 36.41 ± 0.18 μg GAE/mg and finally, the n-butanol fraction (St.n-BuOH) with 35.62 ± 0.1 μg GAE/mg.
Table 1. Values of total polyphenol, flavonoid, and In vitro antioxidant capacity of the various fractions of S. tetragona.
Regarding the quantitative total flavonoid content (TFC), the results were quantified and expressed as quercetin (Q) equivalents and presented in . The values were relatively similar, with slight variations between the fractions. St.EtOA fraction recorded the highest value (p < .05) at 22.77 ± 0.09 µg QE/mg ED, followed by St.n-BuOH fraction with 17.24 ± 0.05 µg QE/mg ED,
St.DCM fraction with 15.57 ± 0.41 µg QE/mg ED and finally, St.Hex fraction with 15.17 ± 0.23 µg QE/mg ED.
The quantitative analysis of phenols and flavonoids in S. tetragona can be explained by various factors due to the absence of any previous study of this plant. These include internal factors such as genetic makeup, developmental stage, hormonal signals, nutrient availability, and abiotic stress, and external factors such as light intensity and quality, temperature, water availability, air pollution, soil quality, pathogens, and pollinators.[Citation14]
The slight variations observed in the quantified phenol and flavonoid content in the fractions of S. tetragona extracts can be attributed to the solvents’ polarity used during the extraction process. Different solvents remove different substances from the plant matrix in various ways due to the varying degree of polarity of phenols, flavonoids, and other secondary metabolites.[Citation15,Citation16]
The quantitative assessment of flavonoids revealed that the n-hexane fraction, despite its low polarity, had similar flavonoid content to the other fractions with relatively high polar solvents. This is because solvents with low polarity can extract some types of flavonoids, particularly isoflavonoids, which have lower polarity compared to other flavonoids. Therefore, although flavonoids are generally considered to have high polarity, the presence of flavonoids in less polar solvents can be attributed to the extraction of isoflavonoids known for their low polarity.[Citation17–19]
In vitro antioxidant activity
Three commonly used assay methods -DPPH, anti-Hemolysis, and FRAP- were utilized to assess the antioxidant activity of four S. tetragona plant fractions. According to the study’s findings (), the plant extract fractions showed significant antioxidant activity. The DPPH test is excellent for determining how healthy antioxidants are at scavenging free radicals. This assay is based on the ability of the antioxidants to reverse and stabilize the DPPH• radical, causing the solution to turn purple to yellow.[Citation20] With an IC50 value of 62 ± 4.10 µg/ml, the dichloromethane fraction (St.DCM) of the plant extract had the most significate (p < .05) activity in the DPPH test. The anti-Hemolysis assay measures the antioxidant activity by evaluating how well the antioxidants defend against hemolysis of red blood cells (rupture). This test is based on antioxidants’ capacity to guard red blood cells against oxidative damage from hydrogen peroxide (H2O2), including phenols, alkaloids, terpenes, and tannins.[Citation21] The hexane fraction (St.Hex) of the plant demonstrated superior performance in the anti-Hemolysis test (p < .05) (IC50 = 200 ± 2.10 µg/ml). The FRAP test assesses antioxidant activity by determining the antioxidant’s capacity to decrease the (Fe3+) to (Fe2+)[Citation22]; in this test, the ethyl acetate fraction (St.EtOA) of the S. tetragona exhibited the most significant antioxidant activity with an (IC50 = 910 ± 30 µg/ml) (p < .05). The current study demonstrates that the fractions of S. tetragona plant extract possess significant antioxidant activity as evaluated by DPPH, FRAP, and anti-Hemolysis assays and suggests that the antioxidant capacity of the fractions may be due to the kind and amount of phenolic and flavonoid bio compounds present ().
Inhibitory activities of α-amylase and α-glucosidase
The antidiabetic potential of the fractions from S. tetragona was evaluated by inhibiting Carbohydrate Metabolism enzymes (α-amylase and α-glucosidase). The results were quantified using IC50 values. Overall, the data suggest S. tetragona possesses significant antidiabetic activity, which aligns with traditional medicinal uses.[Citation6] The inhibitory impacts of various fractions on the alpha-amylase enzyme were evaluated. Results indicated that the n-butanol fraction (St.n-BuOH) and the ethyl acetate fraction (St.EtOA) displayed the highest degree of inhibition (p < .05), with an estimated IC50 value of 70 ± 1.80 µg/ml, 70 ± 1.30 µg/ml respectively. This inhibitory capacity was comparable to that of the acarbose control, which had an activity level of 62 ± 2.80 µg/ml. At the same time, dichloromethane (St.DCM) and hexane (St.Hex) fractions displayed lower inhibitory activity (p < .05), with IC50 values of 124 ± 5.10 µg/ml and 130 ± 0.40 µg/ml, respectively. In regards to the inhibitory properties of the α-glucosidase enzyme, the ethyl acetate and dichloromethane fractions exhibited comparable inhibitory activity (p < .05), with an IC50 value of 70 ± 1.80 µg/ml. This value is significant to the acarbose control, which had an IC50 value of 60 ± 1 µg/ml. The n-hexane and dichloromethane fractions’ inhibitory activity was similar (p < .05), with IC50 values of 130 ± 1.20 µg/ml and 132 ± 3.20 µg/ml, respectively.
The inhibitory effect of S. tetragona fractions is significantly attributed to active natural compounds in both quantitative and qualitative amounts. Several studies have shown that polyphenols and flavonoids inhibit α-amylase and α-glucosidase enzymes, essential in carbohydrate digestion. The inhibition of these enzymes by flavonoids and polyphenols is thought to occur through binding these compounds to the enzyme’s active site. This binding prevents substrate binding and enzymatic activity. Studies have identified several types of flavonoids and polyphenols that have been shown to inhibit these enzymes, such as catechins, procyanidins, quercetin, kaempferol, myricetin, curcumin, gallic acid, ellagic acid, naringenin, apigenin, and luteolin.[Citation23]
One researcher[Citation24] investigated the relationships between flavonoids and human α-amylase to comprehend the molecular needs for enzyme inhibition better. The study discovered that the quantity of hydroxyl radical on the B ring of the flavonoid structure correlates with inhibitory ability. The interaction occurs through hydrogen bonds between the hydroxyl radical in position R6 or R7 of ring A and position R4 or R5 of the ring B of the polyphenol ligands and the catalytic residues of the binding site. A conjugated π-system is also produced, stabilizing the contact with the active site. These findings are consistent with the postulated mode of action for acarbose.[Citation25]
Evidence suggests that the type of solvent used to extract compounds from plants can affect the effectiveness of inhibition digest carbohydrates enzymes (α-amylase, α-glucosidase). Different solvents can selectively extract different types of compounds, and some solvents may be more effective at extracting compounds that inhibit the enzymes in question.[Citation26] This explains the differential inhibitory capacity between the various fractions of S. tetragona hydromethanolic extract.
Acetylcholinesterase inhibition activity
The acetylcholinesterase (AChE) enzyme inhibition assay results, presented in , indicate that the fractions derived from S. tetragona exhibit a potent inhibitory capacity compared to the reference compound, galantamine. The ethyl acetate fraction (St.EtOA) showed the most significate levels of inhibition (p < .05), with an IC50 value of 30 ± 0.30 µg/ml, which is comparable to and slightly less than the inhibitory potency of galantamine, 28 ± 0.30 µg/ml. The n-butanol (St.n-BuOH) fraction exhibited a somewhat lower inhibitory capacity (p < .05), with an IC50 value of 32 ± 0.60 µg/ml. The dichloromethane (St.DCM) and hexane (St.Hex) fractions also displayed inhibitory capacity, with IC50 values of 60 ± 0.10 µg/ml and 63 ± 0.60 µg/ml (p < .05), respectively.
Table 2. Inhibitory Effects of S. tetragona fractions on α-glucosidase, α-amylase, and acetylcholinesterase (AChE).
It is believed that the inhibiting properties of S. tetragona plant fractions are attributed to their active compound’s contents, particularly polyphenols, and flavonoids. These compounds have been shown to exert an inhibitory influence on acetylcholinesterase (AChE) through various mechanisms, which differ from the known inhibitor, galantamine. Specifically, polyphenols such as gallic acid and quercetin have been found to exert a mixed-type inhibition on acetylcholinesterase, which is characterized by binding to both the active site and non-active site of the enzyme, resulting in uncompetitive and noncompetitive inhibition.[Citation27] Flavonoids, including kaempferol and luteolin, have been found to exert a noncompetitive inhibition on acetylcholinesterase, characterized by binding to a location other than the enzyme’s active.[Citation28] In contrast, galantamine, on the other hand, is a reversible competitive inhibitor of acetylcholinesterase that binds to the enzyme’s active site, obstructing substrate binding and decreasing enzyme activity.[Citation29]
Table 3. Pearson’s correlation (R) and significance levels (p-values <.05) between total phenolic, and flavonoid content of various fractions of S.tetragona and their biological activity.
Conclusion
This study aimed to discover the underlying phytochemical content, antioxidant activity, and inhibitory enzyme properties of S. tetragona ariel part fractions. In vitro experiments revealed that S. tetragona fractions have inhibitory effects on enzymes connected to diabetes and Alzheimer’s disease. Furthermore, the study discovered a significant presence of total phenolic and flavonoids in S. tetragona, which enhances its antioxidant and enzyme-inhibitory activities. These findings support the traditional usage of S. tetragona in the treatment of diabetes and open the door to the development of pharmaceuticals for diabetes and associated disorders. Nonetheless, it is necessary to conduct additional in vivo research to fully comprehend the mechanisms of antioxidant and enzyme inhibition, the degree to which the substances are absorbed and utilized by the body, and their metabolic pathways.
Ethical approval and consent to participate
The study does not involve human or animal trials.
Acknowledgments
The authors would like to thank Dr. Youcef Halis, Director of the Technical Research Center on Arid Regions, Algeria, for taxonomic identification of the plant material. Also, the Authors are thankful to Kampala International University, Kampala, for providing the facilities used for this study.
Disclosure statement
No potential conflict of interest was reported by the author(s).
References
- Krishnaiah, D.; Sarbatly, R.; Nithyanandam, R. A Review of the Antioxidant Potential of Medicinal Plant Species. Food and Bioprod. Process 2011, 89(3), 217–233. DOI: 10.1016/j.fbp.2010.04.008.
- Fereidon, S.; Ambigaipalan, P. Phenolics and Polypenolics in Foods, Beverages and Spices: Antioxidant Activity and Health Effects. J. Funct. Foods. 2015, 18, 820–897. DOI: 10.1016/j.jff.2015.06.018.
- Singh, G. Plant Systematics: An Integrated Approach; CRC Press, 2019.
- Lakhdari, W.; Dehliz, A.; Acheuk, F.; Mlik, R.; Hammi, H.; DOUMANDJI-MITICHE, B.; Gheriani, S.; Berrekbia, M.; Guermit, K.; Chergui, S. Ethnobotanical Study of Some Plants Used in Traditional Medicine in the Region of Oued Righ (Algerian Sahara). J. Med. Plants Stud. 2016, 4(2), 204–211.
- Daoud, S.; Elbrik, K.; Tachbibi, N.; Bouqbis, L.; Brakez, M.; Harrouni, M. C. The Potential Use of Halophytes for the Development of Marginal Dry Areas in Morocco. 2016; pp. 141–156. DOI: 10.1016/B978-0-12-801854-5.00009-1.
- Ghourri, M.; Zidane, L.; Douira, A. Usage des plantes médicinales dans le traitement du Diabète Au Sahara marocain (Tan-Tan). J. Anim. Plant Sci. 2013, 17(1), 2388–2411.
- Li, H.; Cheng, K.; Wong, C.; Fan, K.; Chen, F.; Jiang, Y. Evaluation of Antioxidant Capacity and Total Phenolic Content of Different Fractions of Selected Microalgae. Food. Chem. 2007, 102(3), 771–776. DOI: 10.1016/j.foodchem.2006.06.022.
- Chekroun-Bechlaghem, N.; Belyagoubi-Benhammou, N.; Belyagoubi, L.; Gismondi, A.; Nanni, V.; Di Marco, G.; Canuti, L.; Canini, A.; El Haci, I.; Atik Bekkara, F. Phytochemical Analysis and Antioxidant Activity of Tamarix Africana, Arthrocnemum Macrostachyum and Suaeda Fruticosa, Three Halophyte Species from Algeria. Plant. Biosyst-An Int. J. Deal Aspect Plant Bio. 2019, 153(6), 843–852. DOI: 10.1080/11263504.2018.1555191.
- Brand-Williams, W.; Cuvelier, M.-E.; Berset, C. Use of a Free Radical Method to Evaluate Antioxidant Activity. LWT Food Sci. Technol. 1995, 28(1), 25–30. DOI: 10.1016/S0023-6438(95)80008-5.
- Saague, P. W. K.; Moukette Moukette, B.; Njimou, J. R.; Biapa, P. C. N.; Nzufo Tankeu, F.; Moor, V. J. A.; Pieme, C. A.; Ngogang, J. Y. Phenolic Compounds from Water-Ethanol Extracts of Tetrapleura Tetraptera Produced in Cameroon, as Potential Protectors Against in vivo CC L 4 -Induced Liver Injuries. Sci. World J. 2019, 2019, 1–10. DOI: 10.1155/2019/5236851.
- Afsar, T.; Razak, S.; Khan, M. R.; Mawash, S.; Almajwal, A.; Shabir, M.; Haq, I. U. Evaluation of Antioxidant, Anti-Hemolytic and Anticancer Activity of Various Solvent Extracts of Acacia Hydaspica R. Parker Aerial Parts. BMC Complement Altern. Med. 2016, 16(1), 1–16. DOI: 10.1186/s12906-016-1240-8.
- Marmouzi, I.; Tamsouri, N.; El Hamdani, M.; Attar, A.; Kharbach, M.; Alami, R.; El Jemli, M.; Cherrah, Y.; Ebada, S. S.; Faouzi, M. E. A. Pharmacological and Chemical Properties of Some Marine Echinoderms. Rev. Bras. Farmacogn. 2018, 28, 575–581. DOI: 10.1016/j.bjp.2018.05.015.
- Ellman, G. L.; Courtney, K. D.; Andres, V., Jr; Featherstone, R. M. A New and Rapid Colorimetric Determination of Acetylcholinesterase Activity. Biochem. Pharmacol. 1961, 7(2), 88–95. DOI: 10.1016/0006-2952(61)90145-9.
- Šamec, D.; Karalija, E.; Šola, I.; Vujčić Bok, V.; Salopek-Sondi, B. The Role of Polyphenols in Abiotic Stress Response: The Influence of Molecular Structure. Plants. 2021, 10(1), 118. DOI: 10.3390/plants10010118.
- Kim, D. O.; Lee, C. Y. Extraction and Isolation of Polyphenolics. Curr. Protocols In Food Analytical Chemistry. 2002, 6(1), I1. 2.1–I1. 2.12. DOI: 10.1002/0471142913.fai0102s06.
- Chaves, J. O.; De Souza, M. C.; Da Silva, L. C.; Lachos-Perez, D.; Torres-Mayanga, P. C.; Machado, A. P. D. F.; Forster-Carneiro, T.; Vázquez-Espinosa, M.; González-de-Peredo, A. V.; Barbero, G. F. Extraction of Flavonoids from Natural Sources Using Modern Techniques. Front. Chem. 2020, 8, 507887. DOI: 10.3389/fchem.2020.507887.
- Murshid, S. S.; Atoum, D.; Abou-Hussein, D. R.; Abdallah, H. M.; Hareeri, R. H.; Almukadi, H.; Edrada-Ebel, R. Genus Salsola: Chemistry, Biological Activities and Future Prospective—A Review. Plants. 2022, 11(6), 714. DOI: 10.3390/plants11060714.
- ElNaggar, M. H.; Eldehna, W. M.; Abourehab, M. A.; Abdel Bar, F. M. The Old World Salsola as a Source of Valuable Secondary Metabolites Endowed with Diverse Pharmacological Activities: A Review. J. Enzyme Inhib. Med. Chem. 2022, 37(1), 2036–2062. DOI: 10.1080/14756366.2022.2102005.
- Selim, D. A.; Shawky, E.; Ghareeb, D. A.; Abdulmalek, S. A.; El-Khair, R. M. A. Comparative Metabolomics of the Different Fractions of Two Saltwort (Salsola L.) Species in Relation to Their Anti-Inflammatory Activity. Food. Biosci. 2023, 51, 102306. DOI: 10.1016/j.fbio.2022.102306.
- Gülçin, İ. Antioxidant Activity of Caffeic Acid (3, 4-Dihydroxycinnamic Acid). Toxicol. 2006, 217(2–3), 213–220. DOI: 10.1016/j.tox.2005.09.011.
- Shabbir, M.; Khan, M. R.; Saeed, N. Assessment of Phytochemicals, Antioxidant, Anti-Lipid Peroxidation and Anti-Hemolytic Activity of Extract and Various Fractions of Maytenus Royleanus Leaves. BMC Complementary Altern. Med. 2013, 13, 1–13. DOI: 10.1186/1472-6882-13-143.
- Gülçin, İ.; Elmastaş, M.; Aboul-Enein, H. Y. Antioxidant Activity of Clove Oil–A Powerful Antioxidant Source. Arabian J. Chem. 2012, 5(4), 489–499. DOI: 10.1016/j.arabjc.2010.09.016.
- Meng, Y.; Su, A.; Yuan, S.; Zhao, H.; Tan, S.; Hu, C.; Deng, H.; Guo, Y. Evaluation of Total Flavonoids, Myricetin, and Quercetin from Hovenia Dulcis Thunb. as Inhibitors of α-Amylase and α-Glucosidase. Plant. Foods Human. Nutr. 2016, 71(4), 444–449. DOI: 10.1007/s11130-016-0581-2.
- Lo Piparo, E.; Scheib, H.; Frei, N.; Williamson, G.; Grigorov, M.; Chou, C. J. Flavonoids for Controlling Starch Digestion: Structural Requirements for Inhibiting Human α-Amylase. J. Med. Chem. 2008, 51(12), 3555–3561. DOI: 10.1021/jm800115x.
- Svensson, B.; Fukuda, K.; Nielsen, P. K.; Bønsager, B. C. Proteinaceous α-Amylase Inhibitors. Biochim. Biophys. Acta - Proteins Proteom. 2004, 1696(2), 145–156. DOI: 10.1016/j.bbapap.2003.07.004.
- Wang, L.; Clardy, A.; Hui, D.; Gao, A.; Wu, Y. Antioxidant and Antidiabetic Properties of Chinese and Indian Bitter Melons (Momordica Charantia L.). Food. Biosci. 2019, 29, 73–80. DOI: 10.1016/j.fbio.2019.03.010.
- Liao, Y.; Mai, X.; Wu, X.; Hu, X.; Luo, X.; Zhang, G. Exploring the Inhibition of Quercetin on Acetylcholinesterase by Multispectroscopic and in silico Approaches and Evaluation of Its Neuroprotective Effects on PC12 Cells. Molecules. 2022, 27(22), 7971. DOI: 10.3390/molecules27227971.
- Dhananjayan, K.; Sumathy, A.; Palanisamy, S. Molecular Docking Studies and in-Vitro Acetylcholinesterase Inhibition by Terpenoids and Flavonoids. Asian J. Res. Chem. 2013, 6(11), 1011–1017.
- Greenwood, D.; Galantamine. A Novel Treatment for Alzheimer’s Disease. J. Pharm. Pharmacol. 1998, 50(Supplement_9), 20–20. DOI: 10.1111/j.2042-7158.1998.tb02220.x.