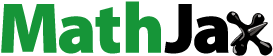
ABSTRACT
This study aimed to explore the chemical composition and biological properties of Dialium guineense essential oils derived from its fruit and leaf. These oils have significant potential as natural alternatives in industries such as food, pharmaceuticals, and cosmetics. The research involved extraction, characterization, antioxidant, and antimicrobial evaluations. The fruit essential oil contained 30 identified compounds, with hexadecanoic acid as the major component. The leaf essential oil had 44 identified compounds, with falcarinol as the predominant compound. Antioxidant capacity was assessed, resulting in a total antioxidant capacity of 70.4 μg/g AAE for the fruit essential oil and 40 μg/g AAE for the leaf essential oil. In the hydrogen peroxide scavenging assay, the fruit essential oil demonstrated an IC50 value of 347.7 ± 0.5 μg/mL, while the leaf essential oil exhibited an IC50 value of 931.7 ± 0.6 μg/mL. The antimicrobial activity of the essential oils was evaluated against various microorganisms. The fruit essential oil showed significant efficacy against S. aureus, S. pneumoniae, K. pneumoniae, P. aeruginosa, E. coli, and C. albicans. The leaf essential oil displayed effectiveness against S. pneumoniae, E. coli, and E. faecalis. Both essential oils inhibited biofilm formation in P. aeruginosa. Molecular docking analysis revealed that compounds in the leaf essential oil had higher binding affinity for LasR, which correlated with superior anti-biofilm activity. These findings suggest that the essential oils from D. guineense have therapeutic potential in combating various diseases.
Introduction
Essential oils (EO) are usually colorless or light yellow, volatile, and strongly scented. They are derived from plant parts such as roots, stems, leaves, fruits, bark, and flowers. They occasionally freeze, easily crystallize, and are liquid at room temperature.[Citation1] These plant oils account for >1% of plant secondary metabolites and usually, are composed of phenylpropanoids, amino acid derivatives, fatty acid derivatives, benzenoids and terpenoids.[Citation1] As a result of the complex chemicals present in them, as well as the diversity of their modes of action, EOs have a broad spectrum of activity.[Citation2] EOs possess lipophilic properties and a low molecular weight, which enables them to penetrate tissues much more quickly and easily.[Citation3] Plant EOs have been used for years due to their anti-inflammatory, antispasmodic, antimicrobial, carminative, antioxidant, and simulative properties, and have thus gained widespread attention.[Citation4]
It has been demonstrated that EOs derived from Plumeria alba and the West African Plum, Vitex doniana, exhibit a broad spectrum of antimicrobial activity against various types of bacteria, including both Gram-positive and Gram-negative strains, as well as fungi.[Citation5,Citation6] Some of these EOs have also displayed preservative properties. Recent studies have revealed that EOs extracted from the halophyte, Lobularia maritima, have natural food preservation effects on meat.[Citation7,Citation8] Other works have also indicated that EOs can inhibit the formation of biofilms by certain bacteria. EOs derived from Averrhoa carambola[Citation9], Chrysophyllum albidum[Citation10], Backhousia citriodora,[Citation11] and Myrtus communis[Citation12] have all demonstrated promising activities in inhibiting biofilm formation. Indications are that that some of these oils function by disrupting the lipid cell membranes of their target organisms or by interfering with essential regulatory systems such as quorum sensing and modulating host immune responses.[Citation13–15] EOs with antioxidant activities are widespread in the literature.[Citation11,Citation16,Citation17] Given their superior biological actions, EOs possess a high potential for development as novel therapeutics for combating a wide range of diseases, as well as natural additives to improve shelf-life and general food safety.
Dialium guineense, also known as the velvet tamarind, is tall, tropical and fruit-bearing. It belongs to trees in the Fabaceae family. The fruits are small with grape-sized edible portions and brown shells which are inedible. D. guineense plant parts have been routinely used in folkloric medicine for treating various diseases. The bark is used to treat stomach aches, headaches, and pains.[Citation18] The leaves are used to treat fever, prenatal pains, and edema.[Citation19] The pulp of the fruits contains proteins and minerals,[Citation20] and some women chew the fruits to facilitate lactation and prevent genital infection.[Citation21] D. guineense leaves and stem bark are also used to treat conditions such as diarrhea, wound, severe cough, fever, bronchitis, malaria, stomachache, and jaundice.[Citation22] Using the agar disc diffusion assay, a previous study reported a broad spectrum of antimicrobial activity of D. guineense leaf extract, with the greatest activity against Streptococcus mutans.[Citation23]
Given the intriguing biological properties of D. guineense, the EOs of the fruits and leaves have also been investigated. Pelissier and coworkers reported that the EOs from the fruit pulp of D. guineense contained acids, alkanes, alkynes, and alcohols. Some predominant compounds identified were nonanal (12.5%), nonanoic acid (11.4%), dodecanoic acid (10.5%) and heptadecane (7%).[Citation24] In Nigeria, EOs highly rich in terpenes and terpenoids were isolated from various parts of D. guineense and found to possess varying biological activities. Precocene was the most abundant compound, with a percentage composition of over 75%. β-caryophyllene (5.3%), valencene (1.4%) and cadalene (1.0%) were other molecules identified in the Nigerian cultivar of D. guineense.[Citation25] EO composition from the same plant might vary, qualitatively and quantitatively, based on such factors as geographical sources, harvesting season, degree of plant maturity, and plant organ used.[Citation26] Due to these factors, and the ethnomedicinal importance of the plant, the EOs present in the leaf and fruit pulp of D. guineense harvested in Ghana were explored. The purpose of this research was to investigate various aspects related to the essential oils derived from the fruit and leaves of D. guineense. The study aimed to determine the chemical composition of these essential oils, assess their ability to scavenge free radicals, evaluate their inhibitory effects against some common pathogens, and examine their potential in eradicating or preventing biofilm formation by bacteria, specifically P. aeruginosa. The antioxidant, antimicrobial, and antibiofilm activities of D. guineense fruits and leaf EOs were evaluated using various in vitro assays. Molecular docking of the oil constituents against the model biofilm-associated protein, LasR from P. aeruginosa, was used to probe the anti-biofilm mechanism of the oil constituents.
Methods
Extraction
Fruits and leaves of D. guineense were collected from Mankessim in the Central Region of Ghana. The samples were identified and verified at the Herbarium of KNUST. A voucher specimen was produced for the plant’s fruit (KNUST/HMI/2021/FR001) and the leaves (KNUST/HMI/2021/FR012). The fruits of D. guineense were peeled off from their shells. To get rid of debris, the fruits were washed under running water. Fruit pulps were de-seeded, placed in plastic bags, sealed, and kept at 4°C until extraction. The leaves were cleaned and allowed to air dry for roughly five days at room temperature. The Clevenger apparatus was used to steam distill the fruit pulp and leaves for 3 hours. The oils were separated from the hydrosol, and the oil dried with anhydrous sodium sulfate. Oil yields were obtained from EquationEquation (1)(1)
(1) :
Essential oil characterization
Gas chromatography-mass spectrometry
The chemical components of the EOs were identified using a gas chromatograph coupled to a mass spectrometer (Perkin Elmer GC-MS). The mass analyzer was a quadrupole. A DB-5 capillary column (ZB-5HTMS; 5% diphenyl/95% dimethylpolysiloxane) whose dimensions were 30 × 0.25 mm ID × 0.25 μm DF was employed as the stationary phase. The GC-MS programs were the same as that used in previous works.[Citation27,Citation28] The GC temperature program consisted of an initial isothermal run at 40°C for 2 min, followed by a temperature increase at a rate of 10°C/min up to 250°C. Subsequently, the temperature was increased at a rate of 20°C/min to reach 280°C, where it was held for 10 min. GC-MS detection utilized an electron ionization system operating in electron impact mode with an ionization energy of 70 eV. Helium (99.9999%) served as the carrier gas at a constant flow rate of 1 mL/min, and a sample injection volume of 1 μL was used. The injector temperature was maintained at 250°C, while the ion-source temperature was set to 220°C. Mass spectra were collected at 70 eV, with a scan interval of 1 s, encompassing fragments ranging from 50 to 4500 Da. A total GC-MS run time of 34.5 minutes was obtained from the method.
Compound identification
The GC-MS studies generated mass spectra, which were then compared to spectra of known compounds in the NIST and Wiley databases to identify the compounds present in the EOs. The names of the compounds were determined based on similarity indices and literature references. The relative proportions of the different constituents were calculated by normalizing the peak area.
Antioxidant assays
A number of assays were utilized in evaluating the antioxidant potential of the oils.
Total antioxidant capacity (TAC)
To assess the EOs’ TAC, the phosphomolybdenum assay was performed. A volume of five milliliters of the PM reagent, comprising 0.6 M sulfuric acid, 28 mM sodium phosphate, and 4 mM ammonium molybdate, was introduced into individual test tubes containing 0.5 mL of each test sample. Subsequently, the test tubes were tightly sealed, vigorously agitated, and subjected to an incubation period of 90 minutes at a temperature of 95°C. Once the samples had cooled down to room temperature, their absorbance was measured at 695 nm with reference to a blank solution. This blank solution was prepared by substituting the test sample with water and incubating it under similar conditions. Ascorbic acid served as the reference standard in this experiment.[Citation29–31] Ascorbic acid was employed as the standard reference. TAC was computed from (2):
where M is the EO mass (g) used in the reaction mix, V represents the total volume in mL of the entire reaction mix, and C is the mass concentration of the standard ascorbic acid used (μg/mL).
DPPH free radical scavenging activity
Various concentrations of essential oils were formulated using a 5% DMSO (dimethyl sulfoxide) solution. A separate solution of DPPH (2,2-diphenyl-1-picrylhydrazyl) at a concentration of 0.1 mM was prepared in methanol. Following this, 50 μL of essential oil was combined with 150 μL of the DPPH solution. The resulting reaction mixture was thoroughly homogenized and placed in a dark environment at room temperature for a duration of 30 minutes. Subsequently, the absorbance of the mixture was measured at a wavelength of 517 nm. Methanol was used in the reaction blank. Ascorbic acid served as a positive control for the experiment.[Citation6,Citation32] The percentage scavenging ability of essential oils was determined using EquationEquation (3)(3)
(3) :
where Acontrol represents the blank solution’s absorbance and Asample is absorbance of test solution. The IC50 values were derived from the curve of the percentage inhibition versus concentration.
Hydrogen peroxide scavenging assay
Similarly, the method employed by Asante Ampadu and coworkers[Citation33] was used to assess the hydrogen peroxide scavenging ability of the EOs. The peroxide scavenging assay was performed by mixing ferrous ammonium sulfate (1 mM), H2O2 (5 mM) and varying concentrations of EO. The reaction mixture was left away from light for 5 minutes at ambient temperature before adding 1,10-phenanthroline (1 mM). The absorbances were read at 510 nm. Gallic acid was utilized as the standard reference and water as the blank solution. IC50 values were determined from a plot of percent hydrogen peroxide scavenged against concentration. Calculation of the percentage of hydrogen peroxide scavenged was by (4).
where Atest is the test sample’s absorbance and Acontrol is the blank’s absorbance.
Inhibition of lipid peroxidation (TBARS assay)
Nartey and colleagues’ method was used to evaluate the ability of EOs to inhibit lipid peroxidation.[Citation10] The oils were added to an egg yolk homogenate solution, then 7 mM FeSO4 was added. The mixture was allowed to stand for 30 minutes at 37°C, followed by the addition of 20% acetic acid (pH 3.5) and a stock reagent (0.8% thiobarbituric acid, 20% trichloroacetic acid in 1.1% sodium dodecyl sulfate). The solution was mixed for 1 minute and heated at 95°C for one hour. After cooling, 5 mL of n-butanol was added, and the solution was then centrifuged (3000 rpm, 10 minutes). The supernatant was used for absorbance reading at 535 nm. Methanol was used as a blank control, BHT and ascorbic acid as positive controls. The percentage inhibition of lipid peroxidation was computed from (1) and IC50 values were determined from a graph of percentage inhibition of lipid peroxidation versus extract concentration.
Data analysis
All experiments were executed in triplicates, and the mean and standard deviations were calculated. The Windows version of the GraphPad Prism program 6.0 was used for all statistical computations (GraphPad Software, San Diego, CA, USA). For all data sets, statistical significance was assumed at p < .05.
Antimicrobial activity
Microbial strains
The antimicrobial activity of the EOs was evaluated against a range of microorganisms, including Gram-negative bacteria (P. aeruginosa ATCC 27,853, E. coli ATCC 25,922, K. pneumoniae ATCC 700,603), Gram-positive bacteria (S. pneumoniae ATCC 49,619, E. faecalis, B. subtilis and S. aureus ATCC 29,213) and a fungus (C. albicans). These microorganisms were donations from the Pharmaceutics Department, KNUST. All bacterial strains were grown overnight at 37°C in nutrient broth, while C. albicans was grown on sabouraud dextrose agar. All bacterial strains were sub-cultured and allowed to grow at 37°C for 18 to 24 hours in an incubator. To achieve a concentration of roughly 2 × 105 CFU/mL, the microbes were inoculated in sterile saline and the turbidity adjusted to the turbidity of a 0.5 McFarland standard solution. The resulting solution was diluted further with sterile double-strength nutrient broth.
Minimum inhibitory concentrations (MIC)
The minimum inhibitory concentrations (MICs) were assessed with the broth dilution method. Ciprofloxacin was used as a standard antibiotic. EOs and ciprofloxacin were prepared by two-fold serial dilution. A 100 μL volume of the drugs were aliquoted into sterile microtiter plates, followed by the addition of 0.1 mL of the appropriate microorganism in nutrient broth. After the plates had been incubated at 37°C for 24 hours, 3-(4, 5-dimethylthiazol-2-yl)-2,5- diphenyltetrazolium bromide solution (MTT, 20 μL, 1.25 mg/mL) was pipetted into each well. After the addition, titer plates were left to stand in an incubator at 37°C for 30 minutes. The MIC was determined to be the lowest possible concentration of the test EO or standard antibiotic that repressed growth. This was shown by the nonappearance of a purple color after MTT addition.[Citation34,Citation35] Each test was run in triplicate.
Zones of inhibition
The agar disc diffusion was used to determine the zones of inhibition of the EOs. On an agar plate, a suspension of the microorganism (∼2.0 × 105 CFU/mL) was introduced. A filter disc was soaked in 30 μL of either 10 mg/mL of standard drug or 30 mg/mL of essential oil. Following the inoculation of the agar plates with the test microorganisms, the discs were impregnated into the agar. The negative control was performed using discs that contained solvents as opposed to EOs. The standard drug was ciprofloxacin. The plates were then put in an incubator (24 hours, 37°C). The antibacterial activity against each test organism was assessed by measuring the zones of inhibition around each filter disc.
Biofilm inhibition
The crystal violet staining method[Citation33] was used. Microtiter wells were filled with 0.1 mL of MIC and sub-MICs of EOs and standard drug (gentamicin). Solvent was used as a negative control in place of the EO. This was followed by the addition of 0.2 mL of the bacterial suspension (at 0.5 McFarland standard) to each well. Microtiter plates were incubated for 24 hours at 37°C. Thereafter, the contents of each well were discarded, and each well washed with deionized water. Crystal violet (0.1%) was used in staining the wells and well contents were eluted with 30% acetic acid into a clean microtiter plate. Absorbances were recorded at 595 nm. EquationEquation 3(3)
(3) was used to estimate the extent of biofilm inhibition.
Molecular docking studies
To perform a molecular docking study, the structure of Las R in complex with its native substrate, N-3-oxo-dodecanoyl-l-homoserine lactone and a quorum sensing anti-activator protein AQS1 was obtained from Protein Data Bank (PDB ID: 6V7X).[Citation6] Both protein and ligands were prepared by removing water molecules. Gasteiger charges, and then polar hydrogens were then added to the protein.[Citation36] Generated output files were all saved in the pdbqt format. In the molecular docking investigation, the most prevalent compounds present in fruit and leaf EOs as well as compounds known to have antimicrobial properties in the literature were considered. The structures of the compounds were drawn and then optimized in Spartan ’14 with appropriate quantum mechanical methods. In validating the docking technique used for precision docking, the AHL was re-docked into the ligand-binding domain of LasR. The docking protocol was validated based on low RMSD (<2 Å) of the re-docked ligand from the conformation of the co-crystallized ligand, and the replication of observed interactions from the pdb structure.[Citation37,Citation38] The preferential binding site of the compounds under investigation were identified by an initial blind docking approach using the AutoDock Vina extension in PyRx. The grid box was set to cover the entire protein in this blind docking. All the compounds which bound in the ligand-binding domain following the blind/whole protein docking experiment were then subjected to a precision docking protocol to explore interactions in the protein-ligand complex. The dimensions of the grid were center – X = 20.0068, Y = −3.27362, Z = −4.70686, and size – X, Y, Z = 20. Each docking score was noted as the binding free energy (kcal/mol). All protein-ligand interactions were examined with the Discovery Studio 2017 R2 client (Dassault Systemes BIOVIA (Discovery Studio Visualizer) (2017 R2 Client), San Diego: Dassault Systemes (2017)).
Results
After extraction, the oils were pale yellow oils, with yields of 0.09% and 0.04% respectively for the fruit and leaf EOs. (fruit) and (leaf) are the GC-MS total ion chromatograms of the EOs from D. guineense. Compound identification was via comparison of the mass spectra obtained to available NIST and Wiley spectra libraries. For the fruit EO, the most abundant compound was hexadecenoic acid (53.13%). Other compounds present in significant amounts were trans-13-octadecanoic acid (22.31%), octadecanoic acid (6.70%) and palmitoleic acid (3.62%). Acids (84.29%), esters (4.12%), alkanes and alkenes (3.90%), alcohols (2.94%), ketones (0.31%), terpenes (0.21%) and phenols (0.11%) were the most common compound classes found in the fruit EO (). For the leaf EO, falcarinol was the most abundant compound (11.13%). Other compounds in high abundance were methyl salicylate (4.60%), cetene (3.40%), nonacosane (3.28%), 2-methoxy-4-vinylphenol (3.20%) and p-xylene (3.04%) – . As shown in , the compounds in the leaf EO could be classified as alkanes and alkenes (24.93%), alcohols (11.59%), ketones (8.03%), esters (7.18%), aldehydes (4.72%), phenols (3.62%), terpenes (3.56%), and acids (1.33%).
Figure 1. Total ion chromatogram (TIC) obtained from the GC-MS run of the essential oil from the fruits of Dialium guineense. Compounds were identified by comparison of MS spectra data with NIST and Wiley libraries as well as published literature. (Chemical structures of some of the peaks are shown and were drawn with ChemDraw.
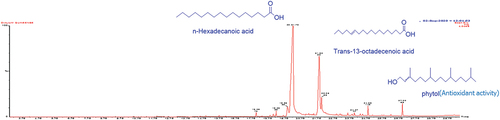
Figure 2. Total ion chromatogram (TIC) obtained from the GC-MS run of the essential oil from the leaves of Dialium guineense. Compounds were identified by comparison of MS spectra data with NIST and Wiley libraries as well as published literature. (Chemical structures of some of the peaks are shown and were drawn with ChemDraw.
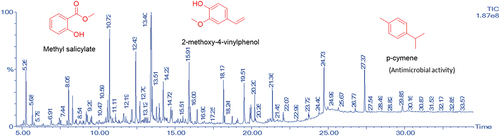
Table 1. Chemical composition of fruit essential oils of Dialium guineense.
Table 2. Chemical composition of leaves essential oils of Dialium guineense.
Table 3. Classification of compounds in the essential oils.
The total antioxidant capacity (TAC) of the fruit and leaf EOs were 70.4 μg/g AAE and 40 μg/g AAE respectively. In the hydrogen peroxide scavenging assay, the IC50 value of the fruit EO was 347.7 ± 0.5 μg/mL while that of the leaf essential oil was 931.7 ± 0.6 μg/mL. The standard, ascorbic acid had an IC50 of 9.1 ± 1.3 μg/mL. Between the fruit and leaf EOs, the fruit EO was a more potent hydrogen peroxide scavenger. In the DPPH assay, the fruit and leaf EOs had IC50 values of 383.5 ± 1.1 μg/mL and 931.7 ± 0.6 μg/mL, respectively. Ascorbic acid, the standard, had an IC50 of 14.30 ± 4.3 μg/mL. For the TBARS assay, the fruit EO exhibited a higher potency to inhibit lipid peroxidation (IC50 value of 1107 ± 0.8 μg/mL) when compared to the leaf EO (IC50 value of 1924 ± 0.9 μg/mL). The IC50 value of the standard, butylated hydroxytoluene (BHT), was 9.2 ± 1.7 μg/mL. A summary of the antioxidant activities of the fruit and leaf EOs has been provided in .
Table 4. Antioxidant activities of leaf and fruit essential oils of D. guineense.
The susceptibility of a panel of microorganisms toward the EOs was tested in the broth dilution and agar well diffusion assays. The fruit EO inhibited the tested microorganisms with zones of inhibition ranging in diameter from 20 mm to 27 mm. Among the test organisms, P. aeruginosa and S. pneumoniae were most susceptible to the fruit EO, with inhibition zones of 27 mm and 26 mm, respectively. A Gram-negative bacterium, K. pneumoniae, had the lowest zone of inhibition of 20 mm. The leaf EO’s inhibition zones for S. pneumoniae and K. pneumoniae were 17 mm and 12 mm, respectively. The leaf EO showed no zones of inhibition for P. aeruginosa, S. aureus, or C. albicans (). For the leaf EO, only C. albicans, the fungus, showed no inhibition zone. Both P. aeruginosa and S. pneumoniae exhibited the highest zones of inhibition (). The antimicrobial activity of the fruit and leaf EOs were also assessed using the broth dilution assay. The EOs’ MICs were between 20 and 40 mg/mL (). The fruit EO was most effective toward S. aureus, S. pneumoniae, P. aeruginosa, K. pneumoniae, and E. coli and C. albicans, with an MIC of 38 mg/mL in all cases. B. subtilis and E. faecalis were the least susceptible microorganisms to the fruit EO. An MIC of 20 mg/mL was obtained for the leaf EO against E. faecalis, S. pneumoniae and E. coli. The leaf EO also repressed the growth of the other tested microbes at an MIC of 40 mg/mL. The crystal violet staining assay was employed in evaluating the EOs’ biofilm inhibitory activity using P. aeruginosa as the test organism. For all concentrations of leaf and fruit EOs examined, biofilm formation was inhibited by more than 45%. The maximum inhibition of biofilm formation was recorded at the MIC for both fruit and leaf EOs at 59% and 64%, respectively ().
Table 5. Zones of Inhibition of Dialium guineense fruit and leaf essential oils from the agar disc diffusion assay.
Table 6. Minimum Inhibitory Concentrations of Dialium guineense fruit and leaf essential oils.
Table 7. Inhibition of biofilm formation in P. aeruginosa by the leaf and fruit essential oils of D. guineense.
Molecular docking was performed on compounds present in both fruit and leaf EOs using the LasR protein of P. aeruginosa. This was done to evaluate the contribution of the compounds in biofilm inhibition. Prior to the precision docking of selected compounds from blind docking, the docking protocol was authenticated by re-docking the co-crystallized ligand, AHL in the ligand binding site of the LasR protein. For the crystal structure, the binding of AHL was stabilized by hydrogen bonds to Trp 60, Ser 129 and Asp 73. Hydrophobic interactions were observed with Ile 52, Ala 50, Leu 40, Val 76, Ala 127, Cys 79, and Leu 125. For the redocked AHL, a binding affinity of −8.4 kcal/mol was recorded. Hydrogen bonds were seen with Thr 75, Ser 129 and Trp 60 while hydrophobic interactions were observed with Tyr 47, Leu 40, Val 76, Ala 70, Leu 125 and Tyr 64 (). The redocked pose was superimposed with that of the crystal structure and an RMSD of 1.071 Å was observed (). With the RMSD < 2 Å, the docking protocol was eligible for further docking studies as it was close to the experimental prediction. Global docking of the compounds against LasR was used to explore the preferential binding sites of the compounds. From the results, 9 out of 30 compounds from the fruit EO bound at the AHL binding domain (). For the leaf EO, 26 out of 45 compounds were observed to bind at the AHL binding domain (). Compounds found in the AHL binding domain were selected for precision docking study to ascertain their binding affinities against LasR. For the fruit essential oil, heptadecanoic acid, hexadecanol, hexadecanoic acid methyl ester, 2-pentadecanone-6,10,14-trimethyl-, 2-propenoic acid,3-phenyl-, ethyl ester, nonanoic acid, palmitoleic acid, pentadecane, and dodecanoic acid bound at the AHL binding domain. For leaf essential oil, 1,6-octadien-3-ol, 3,7-dimethyl-, 1-cyclohexene-1-carboxaldehyde, 5,5-dimethyl-3-oxo-, 1-cyclohexene-1-carboxaldehyde, 2,6,6-trimethyl-, 1-dodecanol-2-hexyl-, 1 H-indene-2,3-dihydro-1,1,5,6-tetramethyl-, 2-furanmethanol, 2-methoxy-4-vinylphenol, 2-pentadecanone, 6,10,14-trimethyl-, 3-(2-Isopropyl-5-methylphenyl)-2-methylpropionic acid, 5,9,13-pentadecatrien-2-one, 6,10,14-trimethyl-(E,E)-, 5,9-undecadien-2-one,6,10-dimethyl-,(E)-, á-ionone, benzaldehyde, 4-methoxy-, benzaldehyde, benzene, 1,2,3,5-tetramethyl-, benzene, 2-(2-butenyl)-1,3,5-trimethyl-, cetene, cyclohexanol, 1-methyl-4-(1-methylethenyl)-, acetate, falcarinol, indolizine, methyl salicylate, nonanal, 2,6-dimethyloctane, p-cymene, benzene acetaldehyde, and p-xylene bound at the AHL binding domain. These compounds were selected for further docking studies since known inhibitors of LasR mostly target the AHL binding domain.
Figure 3. (a) 2D representation of interaction between AHL and LasR. (b) superimposition of co-crystallized AHL (blue) and redocked AHL (yellow), RMSD = 1.071 Å.
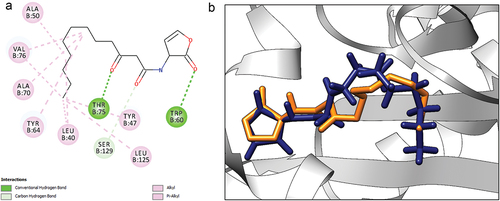
Figure 4. Molecular docking studies on compounds from fruit essential oil against LasR. (a) Global docking of fruit compounds against LasR. Circled region shows the ligand binding domain (b) 2-Pentadecanone, 6,10,14-trimethyl- (c) 2-Propenoic acid,3-phenyl-, ethyl ester.
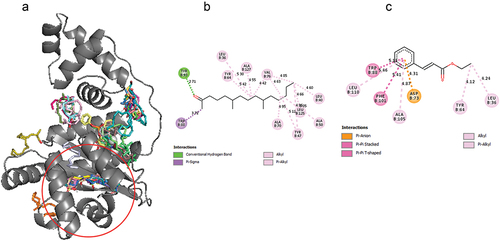
Figure 5. Molecular docking studies on compounds from leaf essential oil against LasR. (a) Global docking of leaf compounds against LasR (b) 1 H-Indene-2,3-dihydro-1,1,5,6-tetramethyl (c) 2-Pentadecanone-6,10,14-trimethyl.
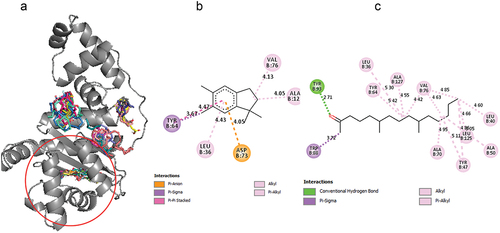
For precision docking, the binding affinities of the fruit EO components ranged from −6.4 kcal/mol to −8.1 kcal/mol. 2-pentadecanone, 6,10,14-trimethyl- recorded a relatively high binding affinity of −8.1 kcal/mol while nonanoic acid recorded a lower binding affinity of −6.4 kcal/mol. 2-pentadecanone, 6,10,14-trimethyl- (−8.1 kcal/mol), 2-Propenoic acid,3-phenyl-, ethyl ester (−7.8 kcal/mol), heptadecanoic acid (−7.5 kcal/mol), palmitoleic acid (−7.6 kcal/mol) and hexadecanol (−7.5 kcal/mol) had binding affinity close to AHL (). This suggests that these compounds could compete with AHL for the binding site and therefore serve as potential LasR inhibitors. Therefore, the binding interactions of these compounds with the LasR protein were further explored. 2-pentadecanone, 6,10,14-trimethyl- formed hydrogen bond with Tyr 93 and hydrophobic interactions with Leu 40, Leu 36, Ala 50, Tyr 47, Tyr 64, Ala 70, Trp 88, Val 76, Leu 125 and Ala 127. 2-Propenoic acid,3-phenyl-, ethyl ester established hydrophobic interactions with Leu 36, Tyr 64, Asp 73, Trp 88, Phe 101, Ala 105 and Leu 110. Heptadecanoic acid formed hydrogen bond with Tyr 93 and Leu 110 and hydrophobic interactions with Leu 36, Tyr 47, Leu 40, Ala 50, Trp 60, Tyr 56, Tyr 64, Trp 88, Val 76, and Phe 101. Hexadecanol formed hydrogen bonding interactions with Ala 127 and extensive hydrophobic interactions with Leu 36, Gly 38, Ile 52, Tyr 56, Tyr 64, Ala 70, Val 76, Trp 88, Ala 105 and Leu 110. Palmitoleic acid formed hydrophobic interactions with Leu 36, Ile 52, Tyr 56, Trp 64, Tyr 60, Ala 105, Val 76, Trp 88, Ala 70, Leu 110 and Gly 126 ().
Table 8. Docking results of LasR with compounds from leaf and fruit essential oils of D. guineense.
For compounds from the leaf EO, binding affinities ranged from −5.8 kcal/mol to −8.3 kcal/mol. In an attempt to identify potential inhibitors of LasR, compounds with binding affinities ranging from −8.3 kcal/mol to −7.5 kcal/mol were considered for further analysis since these binding affinities are close to that recorded for AHL. 1-dodecanol-2-hexyl- (−7.6 kcal/mol) interacted through hydrophobic interactions with Leu 40, Leu 36, Tyr 47, Ala 50, Tyr 64, Trp 60, Tyr 56, Ala 105, Val 76, Trp 88, Phe 101, Ala 70 and Leu 110, and hydrogen bonding interactions with Ser 129. 5,9,13-Pentadecatrien-2-one-6,10,14-trimethyl (−8.1 kcal/mol) formed extensive hydrophobic interactions with Leu 36, Tyr 56, Tyr 64, Ala 70, Val 76, Trp 88, Phe 101, Ala 105 and Ala 127. 2-pentadecanone-6,10,14-trimethyl (−8.1 kcal/mol) interacted with Leu 36, Leu 40, Tyr 47, Ala 50, Tyr 64, Ala 70, Val 76, Trp 88, Leu 125 and Ala 127 through hydrophobic interactions. Hydrogen bonding interaction was observed with Tyr 93. 1 H-indene-2,3-dihydro-1,1,5,6-tetramethyl-(−8.3 kcal/mol) interacted with Leu 36, Tyr 64, Asp 73, Val 76 and Ala127 through hydrophobic contacts. 3-(2-isopropyl-5-methylphenyl)-2-methylpropionic acid (−7.9 kcal/mol) formed hydrophobic interactions with Val 76, Leu 125 and Ala 127. Falcarinol (−7.9 kcal/mol) interacted with Leu 36, Leu 40, Tyr 47, Ile 52, Tyr 56, Trp 60, Tyr 64, Val 76, Trp 88, Phe 101, Ala 105, Leu 110 and Leu 125 through hydrophobic interactions. P-cymene (−7.6 kcal/mol) established hydrophobic contacts with Leu 36, Tyr 56, Tyr 64, Asp 73 and Trp 88. Most of the compounds were observed to interact with Val 76 and Trp 60. A summary has been provided in .
Discussion
Various plant parts such as the fruit, leaves, stem bark and twigs have been identified as important sources of EOs. In this study, EO was extracted from the fruit and leaves of D. guineense using the steam distillation method. EO yields of 0.094% and 0.04% were obtained from the fruit and leaves of the plant respectively. Moronkola extracted EOs from D. guineense and obtained yields of 0.06% for both fruit and leaves using the hydrodistillation method[Citation39] and these figures are similar to that obtained in this current study. Acids (84.29%) were the most abundant constituents in the fruit essential oils. About 65.7% of the compounds identified in the fruit essential oils of the Moronkola study were also acids. In this study, hexadecanoic acid made up 53.13% of all compounds identified in the fruit EOs, making it the most abundant component present. Moronkola also reported hexadecenoic acid (23.29%), as the second most abundant component in their oils obtained. For leaf EOs, the highest percentage compositions were observed for alkanes and alkenes (24.93%) with falcarinol as the most abundant compound. In Moronkola’s work, esters dominated in the leaf EO. The variation in the composition could be attributed to differences in the source, cultivation, vegetative stage and growing season of the plant in the various studies.[Citation26]
The presence of different compounds that gives different fragrances to EOs make them very important. Constituent of EOs are mostly terpenes, phenols, alcohols, ketones and aldehydes.[Citation40] The activities of the various EOs are attributed to the compounds that are present in the oils. EOs have shown promising activities acting as anti-inflammatory, antimicrobial, anti-malarial and anti-oxidant agents. The antioxidant activity of EOs is a of great interest because they may be used in the preservation of foods from the toxic effects of oxidants by scavenging free radicals.[Citation41] Such abilities also make them probable candidates in managing several diseased conditions like cancer, brain dysfunction, cardiac diseases and immune system decline.[Citation42] In this work, the antioxidant action of the oils was examined using the various assays reported in . The hydrogen peroxide scavenging assay revealed comparable scavenging capabilities between the leaf and fruit essential oils. Interestingly, the fruit oil exhibited superior efficacy in scavenging DPPH radicals compared to the leaf oil. Moreover, the fruit oil demonstrated greater potency in inhibiting lipid peroxidation when compared to the leaf oil, as indicated in . The presence of phytol and squalene in fruit EO could be a contributing factor to the observed high activity, since they have been reported to possess antioxidant activity.[Citation43,Citation44] Squalene has also been reported to be an effective antioxidant in a model of lipid peroxidation of liposomes.[Citation44] Additionally, the presence of relatively high amounts of acids and esters in the fruit EO may impact complimentary effects in the observed high activities of the fruit EO.[Citation45]
The likelihood of microbial resistance is decreased by the use of EOs. This is because, different compounds with different possible mode of actions are present, making it difficult to develop resistance against them.[Citation46] In the zone of inhibition experiment, the fruit EO showed a higher zone of inhibition compared to the leaf EO. At 40 mg/mL, both the fruit and leaf EOs showed the highest inhibition zones of 27 mm and 29 mm respectively against P. aeruginosa. Lower zones of inhibition against S. aureus were observed for fruit and leaf EOs respectively, at 22 mm and 18 mm. The fruit EOs showed higher potency against the test organisms. This can be due to the existence of p-cymene in the oil.[Citation47] P-cymene is known to permeate the cell membrane of bacteria, allowing compounds that can inhibit internal organelles like carvacrol to enter into the cell. Nonanoic acid found in the fruit essential oil is also known to possess antimicrobial activity although its mechanism of action is unknown.[Citation48] Thus, the compounds may act synergistically to inhibit the growth of microorganisms.[Citation49] Lower MICs were observed for the fruit EO against all test strains, except B. subtilis and E. faecalis which were susceptible to the oils at greater concentrations (>38 mg/mL). For the leaf EO, a lower MIC was recorded against S. pneumonia, E. faecalis and E. coli (). The leaf EO was observed to be more potent against E. faecalis, E. coli and S. pneumoniae when compared to the fruit EO. However, the fruit EO was observed to be potent against S. aureus, K. pneumoniae, P. aeruginosa, and C. albicans.
Biofilm formation is a key tactic utilized by microorganisms to render antimicrobials ineffective. Inhibition of biofilm is therefore crucial in eliminating bacteria. An investigation of the oils’ ability to inhibit biofilm growth reveals a moderate level of activity, with percentage inhibitions of 59% and 64% for the fruit and leaf EOs, respectively. EOs from several plants such as Spondias mombin, Dodartia orientalis L and Sargassum sp. (brown algae), have been shown to possess anti-biofilm activity.[Citation33,Citation50,Citation51] Some compounds found in the aforementioned plants such as 2-pentadecanone, 6,10,14-trimethyl-, 2-Propenoic acid,3-phenyl-, ethyl ester (ethyl cinnamate), methyl salicylate, nonanal and benzyl aldehyde were also present in D. guineense fruit and leaf EOs. It is therefore possible that these compounds could contribute to the antibiofilm potential of D. guineense EOs.
P. aeruginosa is an unscrupulous human pathogen of important medical interest due to its substantial role in infections of patients with compromised immune systems. This bacterium’s capacity to form a biofilm – a sessile population of cells residing in an extracellular polymeric matrix – is a key aspect of its pathogenicity and treatment resistance. In biofilm formation, LasR – a quorum sensing receptor agonized by an autoinducer called acyl homoserine lactone – serves as a key player. It has been demonstrated that P. aeruginosa‘s generation of virulence factors and total biofilm development are significantly reduced when the LasR quorum sensing circuit is inhibited.[Citation52]
Owing to the biofilm inhibitory potential of the various essential oils, their likely molecular level interactions were explored against LasR through a molecular docking study. Since the las system sits at the apex of the quorum sensing hierarchy, LasR has been reported to activate other quorum sensing systems, and also play a vital role in biofilm formation and virulence gene stimulation. LasI, an AI synthase, is responsible for the biosynthesis of N-(3-oxododecanoyl) homoserine lactone (3-O-C12-HSL). Binding of this molecule to LasR – a transcriptional activator – activates virulence genes such as lasB, lasA, apr, and toxA. LasR contains two binding domains, an AHL binding domain and a DNA binding domain. LasR exist as a homodimer in its active conformation. LasR interacts with AHL through Tyr56, Asp73, Ser 129, and Trp60 by hydrogen bonds and with Leu125, Ile52, Leu40, and Val76 by hydrophobic contact. This means that, compounds that bind strongly at the AHL binding domain, could inhibit signal transduction in the AHL binding domain. From the docking study, 10 out of 30 compounds from the fruit EO bind at AHL binding domain. For the leaf EO, 26 out of 45 bound at the AHL binding domain. Interestingly, from the in vitro study, the leaf EO showed relatively better biofilm inhibitory activity as compared to the fruit EO. This could be due to several compounds from leaf essential oils binding to LasR at the AHL binding domain. This correlates with the in-silico study as most compounds from the leaf EO were observed to bind at the AHL binding domain. From the fruit EO, 2-pentadecanone,6,10,14-trimethyl-, 2-propenoic acid,3-phenyl-, ethyl ester, pentadecanoic acid, hexadecanol, hexadecanoic acid methyl ester, and palmitoleic acid showed relatively good binding affinity compared to AHL. Probing the molecular interactions, the compounds interacted with amino acid residues such as Tyr56, Trp60, Ser 129, Asp73, Leu40, Ile52, Val76, and Leu125. Interactions between compounds and pocket resides were observed to be mostly hydrophobic. For leaf essential oils, 1-dodecanol-2-hexyl, 5,9,13-pentadecatrien-2-one, 6,10,14-trimethyl,2-pentadecanone-6,10,14-trimethyl,1 H-indene-2,3-dihydro-1,1,5,6-tetramethyl,3-(2-isopropyl-5-methylphenyl)-2-methylpropionic acid, falcarinol, and p-cymene showed good binding affinity compared to AHL. Similarly, most interactions were observed to be hydrophobic. This suggest that the compounds may contribute to biofilm inhibition by binding to the AHL binding site of LasR.
Conclusion
The work employed the use of antioxidant and antimicrobial assays to assess the antioxidant and antimicrobial activity of fruit and leaf EOs from Dialium guineense. The essential oils extracted from the fruit and leaf had percentage yields of 0.09% and 0.04%, respectively. The fruit essential oils were found to be rich in acids (84.29%), while the leaf essential oils contained abundant alkanes and alkenes (24.93%). The hydrogen peroxide scavenging assay demonstrated comparable scavenging abilities between the leaf and fruit essential oils. However, the fruit essential oil exhibited stronger potency as a hydrogen peroxide scavenger and in inhibiting lipid peroxidation, with IC50 values of 347.7 ± 0.5 μg/mL and 1107 ± 0.8 μg/mL, respectively. Regarding antimicrobial assessment, the fruit essential oil showed greater susceptibility against P. aeruginosa and S. pneumoniae, resulting in inhibition zones of 27 mm and 26 mm, respectively. On the other hand, the leaf essential oil demonstrated higher potency against E. faecalis, E. coli, and S. pneumoniae compared to the fruit essential oil. In terms of antibiofilm activity, the fruit and leaf essential oils exhibited percentage inhibitions of 59% and 64%, respectively. These findings suggest that the fruit essential oils possess promising antimicrobial and antioxidant capabilities, while the leaf essential oils show the highest potential for inhibiting biofilm formation.
Author contribution
LSB and MKL conceived the study. All experiments were designed by LSB, MKL, GAAA and JOM. GAAA and PA carried out all experimental works. Molecular docking work was done by AB and JOM. GAAA, JOM, PA, AB, MKL and LSB analyzed the data. Initial manuscript was drafted by AB, PA and JOM. All authors read and approved the final manuscript.
Ethical approval
Ethical Approval is not applicable for this article.
Statement of human and animal rights
This article does not contain any studies with human or animal subjects.
Statement of informed consent
There are no human subjects in this article and informed consent is not applicable.
Acknowledgments
Acknowledgment of the KNUST Central Laboratory and Department of Chemistry for facility use, and gratitude to Mrs. Rita Opoku of the Central Laboratory for providing valuable technical support.
Disclosure statement
No potential conflict of interest was reported by the author(s).
Data availability statement
All data generated or analyzed during this study are included in this article.
Additional information
Funding
References
- Dudareva, N.; Negre, F.; Nagegowda, D. A.; Orlova, I. Plant Volatiles: Recent Advances and Future Perspectives. Crit. Rev. Plant Sci. 2006, 25(5), 417–440. DOI: 10.1080/07352680600899973.
- Abdelgaleil, S. A. M.; Mohamed, M. I. E.; Shawir, M. S.; Abou-Taleb, H. K. Chemical Composition, Insecticidal and Biochemical Effects of Essential Oils of Different Plant Species from Northern Egypt on the Rice Weevil, Sitophilus oryzae L. J. Pest Sci. 2016, 89(1), 219–229. DOI: 10.1007/s10340-015-0665-z.
- Atif, M., Ilavenil S.; Devanesan S.; AlSalhi M. S.; Choi K. C.; Vijayaraghavan P.; Alfuraydi A. A.; Alanazi N. F. Essential Oils of Two Medicinal Plants and Protective Properties of Jack Fruits Against the Spoilage Bacteria and Fungi. Ind. Crops Prod. 2020, 147(January), 112239.
- Falleh, H.; Ben Jemaa, M.; Saada, M.; Ksouri, R. Essential Oils: A Promising Eco-Friendly Food Preservative. Food Chem. 2020, 330(January), 127268. DOI: 10.1016/j.foodchem.2020.127268.
- Mamattah, K. M. M.; Adomako, A. K.; Mensah, C. N.; Borquaye, L. S. Chemical Characterization, Antioxidant, Antimicrobial, and Antibiofilm Activities of Essential Oils of Plumeria Alba (Forget-Me-Not). Biochem. Res. Int. 2023, 2023, 1–10. DOI: 10.1155/2023/1040478.
- Odoom, J. F.; Aboagye, C. I.; Acheampong, P.; Asiamah, I.; Darko, G.; Borquaye, L. S.; Maneiro, M. Chemical Composition, Antioxidant, and Antimicrobial Activities of the Leaf and Fruit Essential Oils of the West African Plum, Vitex doniana. J. Chem. 2023, 2023, 1–18. DOI: 10.1155/2023/9959296.
- Ben Akacha, B.; Garzoli, S.; Ben Saad, R.; Brini, F.; Mnif, W.; Kačániová, M.; Ben Hsouna, A. Biopreservative Effect of the Tunisian Halophyte Lobularia maritima Flavonoid Fraction, Used Alone and in Combination with Linalool in Stored Minced Beef Meat. Metabolit Mar 2023, 13(3), 371. DOI: 10.3390/metabo13030371.
- Ben Akacha, B.; Švarc-Gajić, J.; Elhadef, K.; Ben Saad, R.; Brini, F.; Mnif, W.; Smaoui, S.; Ben Hsouna, A. The Essential Oil of Tunisian Halophyte Lobularia maritima: A Natural Food Preservative Agent of Ground Beef Meat. Life. Oct 2022, 12(10), 1571. DOI: 10.3390/life12101571.
- Nartey, D.; Accorley, E. D.; Opoku, R.; Darko, G.; Borquaye, L. S. Essential Oils from Averrhoa carambola L. (Oxalidaceae): Chemical Composition, Antioxidant, Antimicrobial and Anti-Biofilm Potential. Chem. Afr. 2021, 4(4), 741–752. DOI: 10.1007/s42250-021-00259-3.
- Nartey, D.; Gyesi, J. N.; Borquaye, L. S. Chemical Composition and Biological Activities of the Essential Oils of Chrysophyllum albidum G. Don (African Star Apple). Biochem. Res. Int. 2021, 2021, 1–11. DOI: 10.1155/2021/9911713.
- Lim, A. C.; Tang, S. G. H.; Zin, N. M.; Maisarah, A. M.; Ariffin, I. A.; Ker, P. J.; Mahlia, T. M. I. Chemical Composition, Antioxidant, Antibacterial, and Antibiofilm Activities of Backhousia citriodora Essential Oil. Molecules. Jan 2022, 27(15), 4895. DOI: 10.3390/molecules27154895.
- Caputo, L., Capozzolo F.; Amato G.; De Feo V.; Fratianni F.; Vivenzio G.; Nazzaro F. Chemical Composition, Antibiofilm, Cytotoxic, and Anti-Acetylcholinesterase Activities of Myrtus communis L. Leaves Essential Oil. BMC Complement. Med. Ther 2022 May, 22(1), 142. DOI: 10.1186/s12906-022-03583-4.
- Jugreet, B. S.; Suroowan, S.; Rengasamy, R. R. K.; Mahomoodally, M. F. Chemistry, Bioactivities, Mode of Action and Industrial Applications of Essential Oils. Trends Food Sci. Technol. Jul 2020, 101, 89–105. DOI: 10.1016/j.tifs.2020.04.025.
- Mensah, J. O., Boakye A.; Manu P.; Nketia P. B.; Gasu E. N.; Asiamah I.; Borquaye L. S. Computational Studies Provide a Molecular Basis for the Quorum Sensing Inhibitory Action of Compounds from Dioon spinulosum Dyer Ex Eichler. Chem. Select. 2023, 8(1), e202203773.
- Sandner, G.; Heckmann, M.; Weghuber, J. Immunomodulatory Activities of Selected Essential Oils. Biomolecul. 2020, 10(8). DOI: 10.3390/biom10081139.
- Ben Hsouna, A.; Gargouri, M.; Dhifi, W.; Ben Saad, R.; Sayahi, N.; Mnif, W.; Saibi, W. Potential Anti-Inflammatory and Antioxidant Effects of Citrus aurantium Essential Oil Against Carbon Tetrachloride-Mediated Hepatotoxicity: A Biochemical, Molecular and Histopathological Changes in Adult Rats. Environ. Toxicol. 2019, 34(4), 388–400. DOI: 10.1002/tox.22693.
- Gyesi, J. N.; Opoku, R.; Borquaye, L. S. Chemical Composition, Total Phenolic Content, and Antioxidant Activities of the Essential Oils of the Leaves and Fruit Pulp of Annona muricata L. (Soursop) from Ghana. Biochem. Res. Int. 2019, 2019, 1–9. DOI: 10.1155/2019/4164576.
- Besong, E. E.; Balogun, M. E.; Djobissie, S. F. A.; Obu, D. C.; Obimma, J. N. Medicinal and Economic Value of Dialium guineense. Afr. J. Biomed. Res. 2016, 19(3), 163–170.
- Arbonnier, M. Trees, Shrubs and Lianas of West African Dry Zones, 2nd ed.; CIRAD, MARGRAF Publishers Gmbh, MNHN: Paris-France, 2004; pp 1–574.
- Arogba, S. S.; Ajiboro, A. A.; Odukwe, I. J. A Physico‐Chemical Study of Nigerian Velvet Tamarind (Dialium guineense L) Fruit. J. Sci. Food Agric. 1994, 66(4), 533–534. DOI: 10.1002/jsfa.2740660417.
- Nwosu, M. O. Plant Resources Used by Traditional Woman as Herbal Cosmetics in South West Nigeria. Arzte Nat. Fahr. 2000, 41, 111.
- Bero, J., Ganfon H.; Jonville MC.; Frédérich M.; Gbaguidi F.; DeMol P.; Moudachirou M.; Quetin-Leclercq J. In vitro Antiplasmodial Activity of Plants Used in Benin in Traditional Medicine to Treat Malaria. J. Ethnopharmacol. 2009, 122(3), 439–444.
- Ajiboye, A. E.; Ameen, M. T.; Adedayo, M. R. Antimicrobial Activity and Phytochemical Screening of the Fruit Pulp of Dialium guineense (Velvet Tamarind) on Some Microbial Isolates. J. Microbiol. Antimicrob. 2015, 7(4), 33–41. DOI: 10.5897/jma2014.0332.
- Pélissier, Y.; Haddad, C.; Marion, C.; Milhau, M.; Bessière, J. M. Volatile Constituents of Fruit Pulp of Dialium guineense Wild. (Cesalpinaceae). J. Essent. Oil Res. 2001, 13(2), 103–104. DOI: 10.1080/10412905.2001.9699626.
- Essien, E.; Ogunwande, I. A.; Ogunbinu, A. O.; Flamini, G.; Cioni, P. L. Analysis of the Essential Oil of Dialium guineense Willd. J. Essent. Oil Res. 2007, 19(6), 545–547. DOI: 10.1080/10412905.2007.9699327.
- Dhifi, W.; Bellili, S.; Jazi, S.; Bahloul, N.; Mnif, W. Essential oils’ Chemical Characterization and Investigation of Some Biological Activities: A Critical Review. Medicine. 2016, 3(4), 25. DOI: 10.3390/medicines3040025.
- Nartey, D.; Accorley, E. D.; Opoku, R.; Darko, G.; Borquaye, L. S. Chemical Composition, Antimicrobial and Antioxidant Activities of the Leaf Essential Oil of Synsepalum dulcificum (Schum. Et Thonn.) Daniell. J. Ghana Sci. Assoc. 2021, 20(1), 9–17.
- Asante Ampadu, G. A.; Mensah, J. O.; Darko, G.; Borquaye, L. S.; Ramanathan, S. Essential Oils from the Fruits and Leaves of Spondias mombin Linn.: Chemical Composition, Biological Activity, and Molecular Docking Study. Evid. Based Complement. Alternat. Med. 2022, 2022, 1–14. DOI: 10.1155/2022/7211015.
- Afu, G.; Laryea, M. K.; Borquaye, L. S. Biological Efficacy and Toxicological Evaluation of Ethanolic Extract of Cassia nodosa Buch.-Ham. (Leguminosae). J. Chem. 2020, 2020, 1–13. DOI: 10.1155/2020/3983491.
- Borquaye, L. S.; Laryea, M. K.; Gasu, E. N.; Boateng, M. A.; Baffour, P. K.; Kyeremateng, A.; Doh, G.; Ahmad, L. Anti-Inflammatory and Antioxidant Activities of Extracts of Reissantia indica, Cissus cornifolia and Grosseria vignei. Cogent Biol. 2020, 6(1), 1785755. DOI: 10.1080/23312025.2020.1785755.
- Borquaye, L. S.; Saah, S. A.; Adu-Poku, D.; Adu-Gyamfi, L.; Bitian, K.; Bambil, W. Anti-Inflammatory, Antioxidant and Total Phenolic Content of the Ethanolic Extracts of Celtis africana Burm. F. Curr. Sci. Perspect. 2020, 6(3), 43–49.
- Laryea, M. K.; Borquaye, L. S. Antimalarial, Antioxidant, and Toxicological Evaluation of Extracts of Celtis africana, Grosseria vignei, Physalis micrantha, and Stachytarpheta angustifolia. Biochem. Res. Int. 2021, 2021, 1–10. DOI: 10.1155/2021/9971857.
- Asante Ampadu, G. A.; Mensah, J. O.; Darko, G.; Borquaye, L. S. Essential Oils from the Fruits and Leaves of Spondias mombin Linn.: Chemical Composition, Biological Activity, and Molecular Docking Study. Evid. Based Complement. Alternat. Med. 2022, 2022, 1–14. DOI: 10.1155/2022/7211015.
- Aboagye, C. I.; Ampomah, G. B.; Mensah, J. O.; Mensah, C. N.; Nartey, D.; Gasu, E. N.; Borquaye, L. S. N-Benzylimidazoles as Potential Antimicrobial and Antibiofilm Agents–Syntheses, Bioactivity and Molecular Docking Studies. Sci. Afr. 2023, 19, e01529. DOI: 10.1016/j.sciaf.2022.e01529.
- Mensah, C. N.; Ampomah, G. B.; Mensah, J. O.; Gasu, E. N.; Aboagye, C. I.; Ekuadzi, E.; Boadi, N. O.; Borquaye, L. S. N-Alkylimidazole Derivatives as Potential Inhibitors of Quorum Sensing in Pseudomonas aeruginosa. Heliyon. 2022, 8(12), e12581. DOI: 10.1016/j.heliyon.2022.e12581.
- Mensah, J. O.; Ampomah, G. B.; Gasu, E. N.; Adomako, A. K.; Menkah, E. S.; Borquaye, L. S. Allosteric Modulation of the Main Protease (MPro) of SARS-CoV-2 by Casticin—Insights from Molecular Dynamics Simulations. Chem. Afr. 2022, 5(5), 1305–1320. DOI: 10.1007/s42250-022-00411-7.
- Akakpo, L.; Gasu, E. N.; Mensah, J. O.; Borquaye, L. S. Oplodiol and Nitidine as Potential Inhibitors of Plasmodium falciparum Dihydrofolate Reductase: Insights from a Computational Study. J. Biomol. Struct. Dyn. 2023, 1–15. DOI: 10.1080/07391102.2023.2212815.
- Kyei, L. K.; Gasu, E. N.; Ampomah, G. B.; Mensah, J. O.; Borquaye, L. S. An in silico Study of the Interactions of Alkaloids from Cryptolepis sanguinolenta with Plasmodium falciparum Dihydrofolate Reductase and Dihydroorotate Dehydrogenase. J. Chem. 2022, 2022, 1–26. DOI: 10.1155/2022/5314179.
- Moronkola, D. O.; Oladosu, I. A. Chemical Compositions of Lonchocarpus cyanescens Benth., (Fabaceae)—Case Study of Its Volatile Oils, and Two Triterpenoids. Am. J. Plant Sci. 2013, 04(8), 1653–1659. DOI: 10.4236/ajps.2013.48199.
- Ahmed, A. F.; Attia, F. A. K.; Liu, Z.; Li, C.; Wei, J.; Kang, W. Antioxidant Activity and Total Phenolic Content of Essential Oils and Extracts of Sweet Basil (Ocimum basilicum L.) Plants. Food Sci. Hum. Wellness. 2019, 8(3), 299–305. DOI: 10.1016/j.fshw.2019.07.004.
- Maestri, D. M.; Nepote, V.; Lamarque, A. L.; Zygadlo, J. A. Natural Products as Antioxidants. Phytochem. Adv. Res. 2006, 37(661), 105–135.
- Aruoma, O. I. Free Radicals, Oxidative Stress, and Antioxidants in Human Health and Disease. J. Am. Oil Chem. Soc. 1998, 75(2), 199–212. DOI: 10.1007/s11746-998-0032-9.
- Santos, C. C.; Salvadori, M. S.; Mota, V. G.; Costa, L. M.; de Almeida, A. A. C.; de Oliveira, G. A. L.; Costa, J. P.; de Sousa, D. P.; de Freitas, R. M.; de Almeida, R. N. Antinociceptive and Antioxidant Activities of Phytol in vivo and in vitro Models. Neurosci. J. 2013, 2013, 1–9. DOI: 10.1155/2013/949452.
- Amarowicz, R. Squalene: A Natural Antioxidant? Eur. J. Lipid Sci. Technol. 2009, 111(5), 411–412. DOI: 10.1002/ejlt.200900102.
- Dosoky, N. S.; Setzer, W. N. Chemical Composition and Biological Activities of Essential Oils of Curcuma Species. Nutr. 2018, 10(9), 10–17. DOI: 10.3390/nu10091196.
- Kon, K. V.; Rai, M. K. Plant Essential Oils and Their Constituents in Coping with Multidrug-Resistant Bacteria. Expert Rev. Anti Infect. Ther. 2012, 10(7), 775–790. DOI: 10.1586/eri.12.57.
- Marchese, A.; Arciola, C.; Barbieri, R.; Silva, A.; Nabavi, S.; Tsetegho Sokeng, A.; Izadi, M.; Jafari, N.; Suntar, I.; Daglia, M., et al. Update on Monoterpenes as Antimicrobial Agents: A Particular Focus on P-Cymene. Mater. 2017, 10(8), 947.
- Sahin, N.; Kula, I.; Erdogan, Y. Investigation of Antimicrobial Activities of Nonanoic Acid Derivatives. Fresenius Environ. Bull. 2006, 15(2), 141–143.
- Tamgue, O.; Louis, B.; Nguefack, J.; Dongmo, B. L.; Dakole, C. D. Synergism and Antagonism of Essential Oil Fractions of Cymbopogon citratus, Ocimum gratissimum and Thymus vulgaris Against Penicillium expansum. Int J. Plant Pathol. 2011, 2(2), 51–62. DOI: 10.3923/ijpp.2011.51.62.
- Alreshidi, M.; Badraoui, R.; Adnan, M.; Patel, M.; Alotaibi, A.; Saeed, M.; Ghandourah, M.; Al-Motair, K. A.; Arif, I. A.; Albulaihed, Y., et al. Phytochemical Profiling, Antibacterial, and Antibiofilm Activities of Sargassum sp. (Brown Algae) from the Red Sea: ADMET Prediction and Molecular Docking Analysis. Algal Res. 2023, 69, 102912. DOI: 10.1016/j.algal.2022.102912.
- Wang, F.; Wei, F.; Song, C.; Jiang, B.; Tian, S.; Yi, J.; Yu, C.; Song, Z.; Sun, L.; Bao, Y., et al. Dodartia orientalis L. Essential Oil Exerts Antibacterial Activity by Mechanisms of Disrupting Cell Structure and Resisting Biofilm. Ind. Crops Prod. 2017, 109, 358–366. DOI: 10.1016/j.indcrop.2017.08.058.
- O’Brien, K. T.; Noto, J. G.; Nichols-O’Neill, L.; Perez, L. J. Potent Irreversible Inhibitors of LasR Quorum Sensing in Pseudomonas aeruginosa. ACS Med. Chem. Lett. 2015, 6(2), 162–167. DOI: 10.1021/ml500459f.