ABSTRACT
This study investigated the effect of NaNO2 on inhibiting myoglobin-mediated lipid oxidation in muscle using a washed bovine mince model. We measured various parameters, including TBARS and PV to determine lipid oxidation, non-heme iron release, protoporphyrin destruction, and nitrosylmyoglobin production. The results of the study showed that the addition of NaNO2 during myoglobin-mediated lipid oxidation significantly reduced the fading and lipid oxidation of washed muscle (p < 0.05). Additionally, the addition of NaNO2 reduced the release of non-heme iron and the degradation of protoporphyrin in myoglobin. Nitrosylmyoglobin was formed in the washed mince supplemented with NaNO2 and myoglobin. These findings suggest that NaNO2 can effectively inhibit the lipid oxidation process induced by myoglobin, which could be a crucial aspect of nitrite inhibition of lipid oxidation in cured meat products. Overall, the study provides evidence for the potential use of NaNO2 as a food preservative to prevent lipid oxidation in meat products.
Introduction
Lipid oxidation is the major cause of meat quality deterioration, mainly affecting its odor and color[Citation1]. Myoglobin (Mb), which constitutes about 75% of the heme protein in bovine back longissimus muscle, has been identified as a significant factor in promoting lipid oxidation.[Citation2] Several mechanisms have been proposed to explain how Mb promotes lipid oxidation in meat, though there is still some controversy. One such mechanism is the auto-oxidation of Mb, where the Fe (II) in the heme ring is oxidized to Fe (III) of MetMb, generating free radicals that stimulate lipid oxidation.[Citation3] Another hypothesis suggests that released non-heme iron ions during myoglobin oxidation promote lipid oxidation.[Citation4]
Sodium nitrite (NaNO2) is a commonly used additive in cured meat products due to its positive effects on fixing, bacteriostatic properties, and reducing lipid peroxidation of meat at low concentrations (<150 mg/kg).[Citation5] However, excessive addition of NaNO2 (>500 mg/kg) may lead to safety concerns due to the converted N-nitrosamines.[Citation6] Despite this, NaNO2 still plays an essential role in meat processing in practical production due to its low cost, anti-oxidation, color development, and bacteriostatic action.[Citation7] The inhibition effect of nitrite on the oxidation of cured meat fat is attributed to the interaction of NO and heme iron/Mb.[Citation8] Previous study have found that Mb-FeII-NO significantly inhibits oxygen consumption in the lipid peroxidation process using a carotene-linoleic acid model.[Citation9] However, the specific antioxidant mechanisms of NaNO2 in meat systems, particularly for the myoglobin-mediated lipid oxidation, are not yet clear.
Washed mince is a commonly used meat model in studying myoglobin-mediated lipid oxidation due to its ability to remove Mb and eliminate interference from inherent catalysts.[Citation10] Previous studies have utilized the washed mince model to elucidate the antioxidant mechanisms of caffeic acid and phenolic substances against myoglobin-mediated lipid oxidation.[Citation11,Citation12] In this work, we used the washed mince model to investigate the effect of NaNO2 on myoglobin-mediated lipid oxidation and to analyze the inhibitory mechanism. Our objective is to provide both theoretical basis and practical guidance for improving meat quality and safety.
Materials and methods
Sample collection and processing
Meat sample (Longissimus thoracis muscles, from 12th thoracic vertebrae to 5th lumbar vertebrae) used in this study was randomly selected from six bulls (Simmentallocal × Chinese yellow cattle, average age: 2 years old; average live weight: 200 kg) from Yuan Bo Meat Co. Ltd (Zhangye, Gansu, China). The sample was cut into small pieces with an average weight of 100 g and stored in liquid nitrogen (−196°C) for further use.
Preparation of Mb
The extraction of Mb was conducted following previously described methods.[Citation13] A meat sample weighing 100 g was chopped and mixed with 300 mL of extract solvent composed of 10 mmol/L pH 8.0 Tris-HCI, 1 mmol/L EDTA, and 25 g/L Triton X-100. The mixture was then homogenized at 13,000 r/min for 30 s before being centrifuged at 10,000 g for 10 min. The supernatant was filtered using a filter paper to obtain crude myoglobin extract, which was then precipitated using 65% and 95% ammonium sulfate. The precipitate was dialyzed and purified using a Sephadex G-75 gel chromatography column. The concentration of myoglobin was determined by measuring the absorbance at 540 nm and adjusted to a final concentration of 1 mg/mL for use in subsequent experiments.
Preparation of oxymyoglobin
Oxymyoglobin (OxyMb) was prepared according to the method of.[Citation14] Sodium hydrosulfite (50 mg) was added to 5 mL of Mb solution, and the mixture was then dialyzed using 50 mM phosphate buffer (pH 7.0). The OxyMb concentration was determined by measuring the absorbance at 525 nm.
Preparation of washed bovine mince
The preparation of washed mince from bovine followed the method described by.[Citation15] Initially, a meat sample weighing 800 g was ground using a household grinder. The bovine mince obtained was mixed with 2,400 mL of 50 mM phosphate buffer with a pH of 6.3 and homogenized at 12,000 rpm for 1 minute. The resulting suspension was centrifuged to collect the precipitate, and this procedure was repeated three times. The entire process was performed at a temperature of 4°C, and the pH value of the suspension was maintained at 6.3.[Citation16]
Streptomycin sulfate was added to the washed bovine mince at a final concentration of 200 ppm to inhibit microbial growth. The OxyMb solution was added to the washed mince at a final concentration of 40 μmol OxyMb/kg mince, resulting in a sample named M. The NaNO2 was then added to the M sample to obtain washed mince containing OxyMb and NaNO2, resulting in a sample named MN. This final concentration of NaNO2 (100 mg/kg) was set based on Chinese national standard (limit of nitrite added in meat product is 150 mg/kg), with the molar ratio between myoglobin and sodium nitrite being approximately 36:1. The control group was added with same amount of phosphate buffer, resulting in a sample named C. All samples were maintained at a pH of 6.3, with the moisture content adjusted to 90%. The samples were stored in 2 mL centrifuge tubes at 4°C for 9 days. Samples were taken for analysis on days 0, 1, 3, 5, 7, and 9, to monitor the effects of NaNO2 on myoglobin-mediated lipid oxidation in the washed mince model.
Measurement of redness
Sample color was measured using a colorimeter (CR-410, Japan Konica Minolta) with aperture size of 10 mm. The color is represented by the CIE-L*a*b* standard color space including L* value (brightness), a* value (−a* greenness and +a* redness), and b* value (−b* blueness and +b* yellowness). Each sample was measured for 3 times.
Visible spectrum scanning
One g of washed mince was added to 10 mL of cold 50 mM phosphate buffer with a pH of 6.3. The mixture was homogenized at 13,000 rpm for 20 seconds before being centrifuged. The resulting supernatant was filtered using filter paper to remove any impurities. The absorbance of the solution was scanned from 450 to 630 nm, and the content of MetMb was calculated using a modified version of the Krzywicki equation.[Citation14,Citation17]
Measurement of non-heme iron content
The non-heme iron content was determined by the method of Ahn et al.[Citation18] with some modification. Sample (4 g) was added to 12 mL of deionized water and then homogenized (13,000 r, 2 min), and then 1.5 mL solution was mixed with 0.5 mL of 1% ascorbic acid solution containing 0.2 M HCl and 1 mL 11.3% TCA. The mixture was incubated at room temperature for 1 hour and then centrifuged. The supernatant (2 mL) was mixed with 0.8 mL of 10% ammonium acetate and 0.2 mL of phenoxazine reagent, and stand at room temperature for 10 min. The absorbance of the mixture was measured at 562 nm.
Determination of hydrogen peroxide content (H2O2)
Hydrogen peroxide was measured by using the Micro Hydrogen Peroxide Assay Kit (Beijing Solarbio Science & Technology). Hydrogen peroxide reacts with titanium sulfate to form a yellow peroxotitanium complex, which can be measured using spectrophotometry at a wavelength of 415 nm.
Measurement of peroxide value (PV)
The measurement of peroxide value (PV) was conducted with minor modifications to the method described by.[Citation19] Sample (0.50 g) was added in 10 mL of methanol-chloroform mixture (methanol: chloroform = 1:1 (v:v)). After that, 3 mL of 0.5% sodium chloride solution was added. The mixture was centrifuged and 2.5 mL of the lower layer of chloroform was added om methanol-chloroform mixture with a total volume of 5 mL. Finally, 25 μL of a 3.94 M ammonium thiocyanate solution and 25 μL of a 20 mM ferrous chloride solution (containing 3.5% HCl) were added. The mixture was shaken and stand for 20 min. PV was determined by measuring the absorbance at 500 nm.
Measurement of thiobarbituric acid reactive substances (TBARS)
TBARS were determined according to the modified method of.[Citation20] Sample (100 mg) was added to 10 mL of TCA-TBA mixture (0.375% TBA + 15% TCA, containing 0.25 M HCl) and homogenized. The mixture was heated in the water bath at 95°C for 20 min. After cooling to room temperature, the mixture was centrifuged. The absorbance of the supernatant was measured at 532 nm and 600 nm (subtracting for turbidity). The standard curve was determined by using standard tetraethoxypropane.
Measurement of heme irons during storage
The extraction of heme irons followed the method of.[Citation15] The sample (0.5 g) was extracted using 4.5 mL of an acidic acetone reagent (acetone: H2O: 12 M HCl = 90:8:2) for 1.5 min. The mixture was stored in the dark at room temperature for 40 min and then centrifuged. The concentration of heme irons in the sample was determined by measuring the absorbance at 640 nm and 700 nm.
Statistical analysis
Statistical analysis was performed using one-way ANOVA by SPSS 12.0. Significance (p < .05) among the groups was determined with Duncan’s multiple range tests. Each measurement was conducted in triplicates and the results were reported as mean ± standard deviation.
Results
Effect of NaNO2 on redness of washed mince
Color is an important indicator that reflects the freshness of meat and affects consumer preferences. depicts the changes in the redness (a* value) of washed mince (C), washed mince containing Mb (M), and washed mince containing Mb and NaNO2 (MN) during storage. The washed mince (C) showed the lowest redness value (a* = −2.4) throughout the storage period due to the lack of Mb. Washed mince containing Mb (M) had the highest redness value (a* = 4.5) at the beginning of storage. However, its redness value decreased significantly (p < .05) with the increase of storage time, dropping to 1.9 on the 9th day. In contrast, the redness value of washed mince containing Mb and NaNO2 (MN) was 4.4 at the initial storage and remained relatively stable, slightly increasing with the prolongation of storage time (a* = 4.8 on the 9th day). These results indicated that the addition of NaNO2 could effectively inhibit the discoloration of Mb and maintain the redness of the washed mince during storage.
Figure 1. Changes in redness (a* value) of washed mince (C), washed mince containing Mb (M), and washed mince containing Mb and NaNO2 (MN) during storage (4°C).
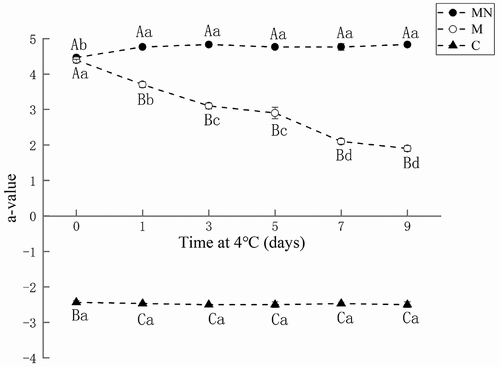
Changes in metmyoglobin (MetMb) and nitrosomyoglobin (Mb-NO) levels
The changes in the absorbance spectra of Mb in different storage periods were shown in . The spectra of Mb correspond to the soluble fraction of the added Mb. The absorbance intensity at 582 nm and 557 nm of the washed mince containing Mb (M) gradually decreased during storage, while the intensity of the absorbance at 503 nm gradually increased (). Moreover, the peak at around 500 nm, which corresponds to the characteristic absorbance peak of the ferric state of heme proteins, gradually increased, indicating that OxyMb in the sample was gradually converted to MetMb, and the content of MetMb increased from 62.02% on day 0 to 94.04% on day 9 (). However, washed mince containing Mb and NaNO2 (MN) has almost no change in the spectrum during storage () due to the formation of nitrosylmyoglobin (Mb-NO).[Citation20]
Changes of H2O2 content in washed mince
Hydrogen peroxide is an active oxygen produced during the oxidation process, and can promote the oxidation reaction in meat. The H2O2 content in samples during different storage periods was shown in . The H2O2 content in all three samples increased during storage. Washed mince containing Mb and NaNO2 (MN) had the lowest H2O2 content compared with other samples. The addition of NaNO2 significantly inhibited the formation of H2O2.
Changes in extractable non-heme iron contents
The content of non-heme iron in samples at different storage periods was shown in . The non-heme iron content in the washed mince (C) ranged from 0.14 μM to 0.27 μM and did not change significantly throughout the storage period. For the washed mince containing Mb (M), its average non-heme iron content increased significantly during storage, from 0.18 μM on day 0 to 0.95 μM on day 9. The concentration of Mb in this work was 40 μM, i.e., the concentration of iron ions bound to myoglobin was 40 μM (one iron atom per myoglobin molecule). These results indicated that about 2.5% of the iron ions were released during storage. However, for the washed mince containing Mb and NaNO2 (MN), there was no significant difference in non-heme iron content during storage, indicating that the addition of NaNO2 significantly inhibited the release of iron ions from myoglobin.
Changes of peroxide value (PV) and TBARS
depicts the PV and TBARS in washed mince (C), washed mince containing Mb (M), and washed mince containing Mb and NaNO2 (MN) during storage. The PV of all samples increased significantly with prolonged storage. Compared with other groups, the washed mince containing Mb (M) had the highest PV, indicating that Mb promoted lipid oxidation in the meat system. The lowest PV was observed in washed mince containing Mb and NaNO2 (MN) (). The TBARS value is used to describe the content of secondary products of lipid oxidation. The TBARS of all samples increased significantly with the increase of storage time. Similarly, washed mince containing Mb (M) had the highest TBARS value (). The TBARS value in washed mince (C) is slightly higher than that of washed mince containing Mb and NaNO2 (MN).
Degradation of heme
During storage, the degradation of heme was significantly inhibited by NaNO2 (). Without the addition of NaNO2, the heme iron content during oxidation was significantly degraded within 9 days of storage (p < 0.05). In contrast, the incorporation of NaNO2 maintained the structure of heme, and no significant loss was observed.
Discussion
Oxidation of meat products will cause nutrient loss and quality deterioration. It will also reduce the sensory quality and affect consumer preference.[Citation21,Citation22] Lipid oxidation and myoglobin oxidation are important causes of off-flavor and discoloration in meat. The oxidation will convert myoglobin into metmyoglobin, resulting in the color change of meat products.[Citation23] The oxidation of unsaturated fatty acids in phospholipids and triacylglycerols can lead to off-flavor development.[Citation24] These two oxidations occur independently but are also interrelated and further accelerate the oxidation process.[Citation25] The specific mechanism of sodium nitrite in the process of inhibiting meat oxidation, especially in the process of lipid oxidation mediated by myoglobin, still lacks a systematic and in-depth scientific explanation. In this work, we used the washed bovine mince model as an object to eliminate the interference caused by the auto-oxidation of hemoglobin (Hb) and myoglobin (Mb) contained in beef itself, to study the action mechanism of sodium nitrite on myoglobin-mediated lipid oxidation.
Mb is the major heme protein found in beef, especially in the Longissimus thoracis muscle, accounting for 75% of the total heme protein. It endows meat red color when existing in the form of oxygenated myoglobin (OxyMb).[Citation26] During storage, the redness of the washed mince containing Mb (M) decreased significantly during storage, which was because OxyMb was gradually oxidized to MetMb, causing the color of the meat to fade. Adding NaNO2 to Mb-containing washed mince can effectively inhibit color fading, because NaNO2 forms nitric oxide (NO), and then nitric oxide can react with Mb to form nitrosomyoglobin (Mb-NO).[Citation27] This compound has a bright red color, which gives the cured meat its characteristic color.
As the storage time increases, the OxyMb in sample M was gradually transformed into MetMb, showing a brown color. Previous study also observed that the Mb of sperm whale was converted from OxyMb to MetMb with a blue shift (418 nm-409 nm) soret band.[Citation8] The MN sample in which NaNO2 was added showed less discoloration during storage, and the spectrum showed similar pattern due to the formation of stable nitrosylmyoglobin (Mb-NO). In acidic environment (pH < 7), nitrous acid derived from NaNO2 tends to form N2O3 which can be reduced to NO.[Citation28] Myoglobin then reacts with NO to form a structurally stable nitrosylmyoglobin.[Citation29] The addition of NaCl accelerates the formation of Mb-NO. The dissociation of chloride ions from sodium chloride in cured meat is known to enhance the conversion of nitrite to nitric oxide, which can accelerate the formation of the characteristic color of cured meat.[Citation30]
Heme or other iron-containing proteins may denature/degrade over time, resulting in the release of iron. Previous work has shown that heme iron extracted from fresh oxeye scad muscle was reduced, and non-heme iron is increased during storage.[Citation31] The destruction of the porphyrin ring due to muscle protein degradation results in the release of free iron ions, which then acts as an oxidant to promote lipid oxidation.[Citation32] In this work, the non-heme iron content of sample M (washed mince containing Mb) was significantly increased at the end of the storage period. The binding capacity of heme prosthetic group to globin in MetMb was 60 times lower than that in OxyMb.[Citation33,Citation34] The oxidation process resulted in a reduction in the affinity of heme to the globin in the heme protein. However, when NaNO2 was added, the release of free iron was significantly inhibited. NO complexes with heme protein to prevent iron being released from porphyrins. The results indicated that NaNO2 can inhibit the release of free iron from myoglobin in the washed mince model.
The auto-oxidation of OxyMb can generate superoxide O2− which subsequently dismutates into H2O2. The O2− will trigger the oxidative rancidity of fresh meat.[Citation35] Our work shows that the H2O2 content of the washed mince containing Mb was significantly increased, which is in accordance with the increase of free iron content. H2O2 can further react with transition metal ions or iron-containing hemoproteins such as myoglobin and hemoglobin to generate hydroxyl radicals by the Fenton reaction.[Citation36] H2O2 interacts with heme proteins to liberate free iron from its porphyrin coordination. This reaction also accelerates the decomposition of hydroperoxides to free radicals, resulting in the formation of two active hypervalent myoglobin species, perferrylmyoglobin and ferrylmyoglobin, which participate in lipid oxidation catalysis.[Citation34] NaNO2 significantly inhibited the formation of H2O2. Although the C treatment removes myoglobin, some residual myoglobin may still be present. Therefore, the C treatment exhibited a slightly higher hydrogen peroxide content compared to the MN treatment. We contend that the formation of nitrosylmyoglobin inhibits a series of reactions in the automatic oxidation of OxyMb, and therefore, the formation of H2O2 was lowered due to the inhibited oxidation of OxyMb.
The TBARS and PV values of the sample containing Mb was significantly higher than washed mince (sample C), indicating that Mb promoted lipid oxidation. The reason could be attributed from two aspects. First, H2O2 produced during the auto-oxidation of OxyMb can react with MetMb to form a highly active H2O2-MetMb complex, which can generate ferrylmyoglobin (MbFe(IV)),[Citation37] and H2O2-MetMb and MbFe(IV) have strong pro-oxidative effects. The second reason is attributed to Mb hemin and free iron. Hemin can easily convert peroxide into free radicals, thereby promoting lipid oxidation.[Citation38] In our work, the inhibition of NaNO2 on Mb-mediated lipid oxidation was achieved by inhibiting the formation of H2O2 and free iron in the above-mentioned processes. The incorporation of NaNO2 inhibited Mb-mediated lipid oxidation (TBARS, PV), H2O2, and free iron. We contend that Mb and NaNO2 formed a stable Mb-NO, thereby retarding the transformation of OxyMb to MetMb, and then reduced the production H2O2 to inhibit lipid oxidation. Previous work has evaluated the in vitro antioxidant activity of NaNO2. In the carotene-linoleic acid model, 2–10 μM of MetMb or OxyMb was found to act as a pro-oxidant. In contrast, Mb-Fe(II)-NO prevented the prooxidative effects of myoglobin toward lipid peroxidation.[Citation39] It was further found that Mb-FeII-NO significantly inhibited oxygen consumption in the lipid peroxidation model by using methyl linoleate as the substrate,[Citation40] also reported similar results that Mb-NO acted as a bioactive reservoir to scavenge hydroxyl radicals or lipid-derived free radicals.[Citation41]
In Mb-mediated lipid oxidation, free iron and heme react with lipid hydroperoxide (LOOH) located in the cell membrane to produce lipid free radicals that extracts hydrogen atoms from polyunsaturated fatty acids, which further forms a primary lipid oxidation product LOOH and a secondary lipid oxidation product.[Citation42] These lipid groups also have the potential to degrade protoporphyrin.[Citation43] Based on this method of detecting intact porphyrin content by acidic acetone extraction, most of the heme degradation during Mb-mediated lipid oxidation was observed in our study. This indicates that the free radicals formed by hemin-mediated LOOH decomposition not only extract hydrogen atoms from the PUFA but also degrade the heme ring by attacking MetMb during storage of washed mince. After the addition of NaNO2, the degradation of the heme was significantly inhibited. This is because NaNO2 inhibited myoglobin-mediated lipid oxidation, reduced the generation of lipid free radicals and inhibited the further degradation of MetMb, thus maintaining the complete heme content.
Conclusion
In this study, the washed bovine mince containing Mb was used as a model system to investigate the inhibitory effect of NaNO2 on Mb-mediated lipid oxidation during storage. The results showed that the addition of Mb induced significant discoloration and lipid oxidation in the washed bovine mince during storage. However, the addition of NaNO2 significantly reduced sample discoloration and the release of non-heme iron during storage. Further analysis revealed that NaNO2 and Mb formed a stable Mb-NO structure, which reduced the degradation of protoporphyrin IX in Mb and inhibited the release of iron ions in myoglobin. This slowed down the transformation of OxyMb to MetMb and reduced the production of H2O2, which in turn inhibited lipid oxidation. These findings provide important insights into the specific inhibitory mechanism by which NaNO2 inhibits Mb-mediated lipid oxidation.
Acknowledgments
This work was supported by the National Natural Science Foundation of China (No. 31771905), Gansu Province Science and Technology Key Research and Development Program (No. 18YF1NA075), the Program for China Agriculture Research System (No. CARS-37), and Fostering Foundation for the Excellent Ph.D. Dissertation of Gansu Agricultural University (No. 2017003; 2018003).
Disclosure statement
No potential conflict of interest was reported by the author(s).
References
- Horbanczuk, O. K.; Kurek, M. A.; Atanasov, A. G.; Brncic, M.; Brncic, S. R. The Effect of Natural Antioxidants on Quality and Shelf Life of Beef and Beef Products. Food Technol. Biotechnol. 2019, 57(4), 439–447. DOI: 10.17113/ftb.57.04.19.6267.
- Purohit, A.; Singh, R.; Kerr, W.; Mohan, A. Effects of Heme and Nonheme Iron on Meat Quality Characteristics During Retail Display and Storage. J. Food Meas. Charact. 2015, 9(2), 175–185. DOI: 10.1007/s11694-015-9222-y.
- Wang, X. T.; Wang, Z. B.; Zhuang, H.; Nasiru, M. M.; Yuan, Y.; Zhang, J. H.; Yan, W. J. Changes in Color, Myoglobin, and Lipid Oxidation in Beef Patties Treated by Dielectric Barrier Discharge Cold Plasma During Storage. Meat. Sci. 2021a, 176, 108456. DOI: 10.1016/j.meatsci.2021.108456.
- Wongwichian, C.; Klomklao, S.; Panpipat, W.; Benjakul, S.; Chaijan, M. Interrelationship Between Myoglobin and Lipid Oxidations in Oxeye Scad (Selar Boops) Muscle During Iced Storage. Food. Chem. 2015, 174, 279–285. DOI: 10.1016/j.foodchem.2014.11.071.
- Ma, G.; Wang, Z.; Chen, H.; Yu, Q.; Han, L. Effect of Low-Dose Sodium Nitrite Treatment on the Endogenous Antioxidant Capacity of Yak Meat During Wet Curing: Pros and Cons. LWT. 2021, 141, 110879. DOI: 10.1016/j.lwt.2021.110879.
- Lee, H. S. Literature Compilation of Volatile N -Nitrosamines in Processed Meat and Poultry Products - an Update. 2019, 36(10), 1491–1500. DOI: 10.1080/19440049.2019.1649472.
- Gassara, F.; Kouassi, A. P.; Brar, S. K.; Belkacemi, K. Green Alternatives to Nitrates and Nitrites in Meat-Based Products–A Review. Crit. Rev. Food Sci. Nutr. 2016, 56(13), 2133–2148. DOI: 10.1080/10408398.2013.812610.
- Ma, G. Y.; Chen, H.; Zhang, Q.; Ma, J. B.; Yu, Q. L.; Han, L.; Chen, C.; Song, R. D. Protective Characterization of Low Dose Sodium Nitrite on Yak Meat Myoglobin in a Hydroxy Radical Oxidation Environment: Fourier Transform Infrared Spectroscopy and Laser Micro-Raman Spectroscopy. LWT Food Sci. Technol. 2019, 116, 108556. DOI: 10.1016/j.lwt.2019.108556.
- Moller, J. K. S.; Sosniecki, L.; Skibsted, L. H. Effect of Nitrosylmyoglobin and Saturated Fatty Acid Anions on Metmyoglobin-Catalyzed Oxidation of Aqueous Methyl Linoleate Emulsions. Biochimica. Et Biophysica Acta-General Sub. 2002, 1570(2), 129–134. DOI: 10.1016/S0304-4165(02)00186-1.
- Liu, L.; Yin, J.; Richards, M. P. Role of Maillard Reaction Products as Antioxidants in Washed Cod and Washed Turkey Muscle Oxidized by Added Hemoglobin. Eur. J. Lipid Sci. Technol. 2022, 124(7), 2100204. DOI: 10.1002/ejlt.202100204.
- Park, S. Y.; Undeland, I.; Sannaveerappa, T.; Richards, M. P. Novel Interactions of Caffeic Acid with Different Hemoglobins: Effects on Discoloration and Lipid Oxidation in Different Washed Muscles. Meat. Sci. 2013, 95(1), 110–117. DOI: 10.1016/j.meatsci.2013.04.003.
- Thiansilakul, Y.; Benjakul, S.; Grunwald, E. W.; Richards, M. P. Retardation of Myoglobin and Haemoglobin-Mediated Lipid Oxidation in Washed Bighead Carp by Phenolic Compounds. Food. Chem. 2012, 134(2), 789–796. DOI: 10.1016/j.foodchem.2012.02.182.
- Thiansilakul, Y.; Benjakul, S.; Richards, M. P. Isolation, Characterisation and Stability of Myoglobin from Eastern Little Tuna (Euthynnus Affinis) Dark Muscle. Food. Chem. 2011, 124(1), 254–261. DOI: 10.1016/j.foodchem.2010.06.028.
- Tang, J.; Faustman, C.; Hoagland, T. A. Krzywicki Revisited: Equations for Spectrophotometric Determination of Myoglobin Redox Forms in Aqueous Meat Extracts. J. Food Sci. 2004, 69(9), C717–C720. DOI: 10.1111/j.1365-2621.2004.tb09922.x.
- Lee, S. K.; Tatiyaborworntham, N.; Grunwald, E. W.; Richards, M. P. Myoglobin and Haemoglobin-Mediated Lipid Oxidation in Washed Muscle: Observations on Crosslinking, Ferryl Formation, Porphyrin Degradation, and Haemin Loss Rate. Food. Chem. 2015, 167, 258–263. DOI: 10.1016/j.foodchem.2014.06.098.
- Richards, M. P.; Modra, A. M.; Li, R. Role of Deoxyhemoglobin in Lipid Oxidation of Washed Cod Muscle Mediated by Trout, Poultry and Beef Hemoglobins. Meat. Sci. 2002, 62(2), 157–163. DOI: 10.1016/S0309-1740(01)00242-X.
- Krzywicki, K. The Determination of Haem Pigments in Meat. Meat .Sci. 1982, 7(1), 29–36. DOI: 10.1016/0309-1740(82)90095-X.
- Ahn, D.; Wolfe, F.; Sim, J. Three Methods for Determining Nonheme Iron in Turkey Meat. J. Food Science. 1993, 58(2), 288–291. DOI: 10.1111/j.1365-2621.1993.tb04257.x.
- Richards, M. P.; Hultin, H. O. Effect of pH on Lipid Oxidation Using Trout Hemolysate as a Catalyst: A Possible Role for Deoxyhemoglobin. J. Agric. Food Chem. 2000, 48(8), 3141–3147. DOI: 10.1021/jf991059w.
- Otaiza-González, S. N.; Mary, V. S.; Arias, S. L.; Bertrand, L.; Velez, P. A.; Rodriguez, M. G.; Rubinstein, H. R.; Theumer, M. G. Different Salicylic and Jasmonic Acids Imbalances are Involved in the Oxidative Stress-Mediated Cell Death, Induced by Fumonisin B1 in Maize Seedlings with Contrasting Resistance to Fusarium Verticillioides Ear Rot in the Field. BioRxiv, 2019.2012. 2025.882597. 2019.
- Amaral, A. B.; da Silva, M. V.; Lannes, S. C. D. Lipid Oxidation in Meat: Mechanisms and Protective Factors – a Review. Food Sci. Technol. 2018, 38(suppl 1), 1–15. DOI: 10.1590/fst.32518.
- Domínguez, R.; Pateiro, M.; Gagaoua, M.; Barba, F. J.; Zhang, W.; Lorenzo, J. M. A Comprehensive Review on Lipid Oxidation in Meat and Meat Products. Antioxidants. 2019, 8(10), 429. DOI: 10.3390/antiox8100429.
- Wang, Z. M.; Tu, J. C.; Zhou, H.; Lu, A.; Xu, B. C. A Comprehensive Insight into the Effects of Microbial Spoilage, Myoglobin Autoxidation, Lipid Oxidation, and Protein Oxidation on the Discoloration of Rabbit Meat During Retail Display. Meat.Sci. 2021b, 172, 108359. DOI: 10.1016/j.meatsci.2020.108359.
- Han, J. Y.; Lee, S. J. Mathematical Modeling of Off-Flavor Development During Beef Storage. Meat. Sci. 2011, 88(4), 712–717. DOI: 10.1016/j.meatsci.2011.03.001.
- Faustman, C.; Sun, Q.; Mancini, R.; Suman, S. P. Myoglobin and Lipid Oxidation Interactions: Mechanistic Bases and Control. Meat. Sci. 2010, 86(1), 86–94. DOI: 10.1016/j.meatsci.2010.04.025.
- Gupta, J.; Bower, C. G.; Cavender, G. A.; Sullivan, G. A. Effectiveness of Different Myoglobin States to Minimize High Pressure Induced Discoloration in Raw Ground Beef. LWT Food Sci. Technol. 2018, 93, 32–35. DOI: 10.1016/j.lwt.2018.03.008.
- Sindelar, J. J.; Milkowski, A. L. Human Safety Controversies Surrounding Nitrate and Nitrite in the Diet. Nitric. Oxide. 2012, 26(4), 259–266. DOI: 10.1016/j.niox.2012.03.011.
- Pereira, C.; Ferreira, N. R.; Rocha, B. S.; Barbosa, R. M.; Laranjinha, J. The Redox Interplay Between Nitrite and Nitric Oxide: From the Gut to the Brain. Redox. Biol. 2013, 1(1), 276–284. DOI: 10.1016/j.redox.2013.04.004.
- Adamsen, C. E.; Moller, J. K. S.; Laursen, K.; Olsen, K.; Skibsted, L. H. Zn-Porphyrin Formation in Cured Meat Products: Effect of Added Salt and Nitrite. Meat. Sci. 2006, 72(4), 672–679. DOI: 10.1016/j.meatsci.2005.09.017.
- SEBRANEK, J. G.; Fox, J., Jr. Rate of Nitric Oxide Formation from Nitrite as Affected by Chloride Ion Concentration. J. Muscle Foods. 1991, 2(1), 11–20. DOI: 10.1111/j.1745-4573.1991.tb00437.x.
- Wongwichian, C.; Chaijan, M.; Panpipat, W.; Klomklao, S.; Benjakul, S. Autolysis and Characterization of Sarcoplasmic and Myofibril Associated Proteinases of Oxeye Scad (Selar Boops) Muscle. J. Aquat. Food Prod. Technol. 2016, 25(7), 1132–1143. DOI: 10.1080/10498850.2015.1032462.
- Thiansilakul, Y.; Benjakul, S.; Richards, M. P. Changes in Heme Proteins and Lipids Associated with Off-Odour of Seabass (Lates Calcarifer) and Red Tilapia (Oreochromis mossambicus×O. Niloticus) During Iced Storage. Food. Chem. 2010, 121(4), 1109–1119. DOI: 10.1016/j.foodchem.2010.01.058.
- Baron, C. P.; Andersen, H. J. Myoglobin-Induced Lipid Oxidation. A Review. J. Agric. Food Chem. 2002, 50(14), 3887–3897. DOI: 10.1021/jf011394w.
- Grunwald, E. W.; Richards, M. P. Studies with Myoglobin Variants Indicate That Released Hemin is the Primary Promoter of Lipid Oxidation in Washed Fish Muscle. J. Agric. Food Chem. 2006, 54(12), 4452–4460. DOI: 10.1021/jf0603228.
- Burns, J. M.; Cooper, W. J.; Ferry, J. L.; King, D. W.; DiMento, B. P.; McNeill, K.; Miller, C. J.; Miller, W. L.; Peake, B. M.; Rusak, S. A., et al. Methods for Reactive Oxygen Species (ROS) Detection in Aqueous Environments. Aquat. Sci. 2012, 74(4), 683–734.
- Zainudin, M. A. M.; Poojary, M. M.; Jongberg, S.; Lund, M. N. Light Exposure Accelerates Oxidative Protein Polymerization in Beef Stored in High Oxygen Atmosphere. Food. Chem. 2019, 299, 125132. DOI: 10.1016/j.foodchem.2019.125132.
- Kanner, J.; Harel, S. LIPID-PEROXIDATION and OXIDATION of SEVERAL COMPOUNDS by H2O2 ACTIVATED METMYOGLOBIN. Lipids. 1985, 20(9), 625–628. DOI: 10.1007/BF02534290.
- Wu, H. Z.; Xiao, S. L.; Yin, J.; Zhang, J. H.; Richards, M. P. Impact of Lipid Composition and Muscle Microstructure on Myoglobin-Mediated Lipid Oxidation in Washed Cod and Pig Muscle. Food. Chem. 2021, 336, 127729. DOI: 10.1016/j.foodchem.2020.127729.
- Kanner, J. Nitric Oxide and Metal-Catalyzed Reactions. Nitric. Oxide, Pt B. 1996, 269, 218–229.
- Moller, J. K. S.; Skibsted, L. H. Mechanism of Nitrosylmyoglobin Autoxidation: Temperature and Oxygen Pressure Effects on the Two Consecutive Reactions. Chemistry. 2004, 10(9), 2291–2300. DOI: 10.1002/chem.200305368.
- Baron, C. P.; Moller, J. K. S.; Skibsted, L. H.; Andersen, H. J. Nitrosylmyoglobin as Antioxidant—Kinetics and Proposed Mechanism for Reduction of Hydroperoxides. Free. Radical Res. 2007, 41(8), 892–902. DOI: 10.1080/10715760701416475.
- Czech, A.; Ognik, K.; Laszewska, M.; Sembratowicz, I. The Effect of Raw and Extruded Linseed on the Chemical Composition, Lipid Profile and Redox Status of Meat of Turkey Hens. Anim. Sci. Papers Rep. 2017, 35, 57–69.
- Nagababu, E.; Rifkind, J. M. Heme Degradation by Reactive Oxygen Species. Antioxid. Redox Signaling. 2004, 6(6), 967–978. DOI: 10.1089/ars.2004.6.967.