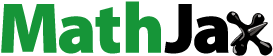
ABSTRACT
Nutrient and toxicant levels as well as their bioavailability in S. latissima and Ulva species (fenestrata, intestinalis, rigida) were reviewed. Nutritional quality was assessed by nutrient contribution to daily reference intake (DRI) per portion (5 g dry weight), nutrient density score NRF21.3, and comparisons to reference foods. Toxicological assessments comprised tolerable daily intake (TDI)-levels. Based on mean %DRI per portion, S. latissima and Ulva species were good sources (%DRI >15) of calcium, magnesium, iron, selenium, and vitamin B12. Mean %DRI was <10% for fiber, sodium, and protein. Toxicological concerns were mainly due to iodine (mean %TDI per portion: 3160% for S. latissima and 41–91% for Ulva species). Mean %TDIs for inorganic arsenic, cadmium, and lead were <20% for S. latissima and 9–97%, 6–15%, and 21–46%, for the selected Ulva species, respectively. Bioavailability data were scarce and is, together with nutritional impact of processing, an important aspect to address in future studies.
Introduction
The market of seaweed – marine macroalgae – for human consumption is growing rapidly in Europe and globally, driven by the search for novel tasty and nutritious foods with low environmental impact.[Citation1,Citation2] Production of seaweed supplied from aquaculture or wild stocks is suggested to have great potential as a resource-efficient source of nutrients, including protein[Citation3] with lower environmental impact compared to most animal-based foods and without competing for arable land.[Citation4,Citation5] Mapping the variability in nutritional composition of different seaweed species is suggested as an important prerequisite to guide producers and consumers toward healthier choices.[Citation6]
Seaweed provides essential macro- and micronutrients.[Citation7] The high content of minerals[Citation8] as well as the presence of vitamin B12 and long-chain n-3 fatty acids distinguish seaweed from conventional plant-based foods. However, improved knowledge of bioavailability and risk-benefit analyses of seaweed are identified as main research priorities to support sustainability in future European seaweed-based product development.[Citation2] A concern when considering seaweed as food is the risk for toxic levels of heavy metals and high concentrations of iodine in some species.[Citation9,Citation19]
Saccharina latissima (S. latissima) and Ulva spp. are seaweeds of increasing interest in a European production and consumption perspective.[Citation12] Previous studies have reviewed the nutritional composition of seaweed biomass in general;[Citation6,Citation13] of red seaweed species;[Citation14] or have focused on selected nutrients.[Citation8,Citation15–17] However, the complete nutritional quality and levels of potentially toxic elements of S. latissima and Ulva spp. remain to be thoroughly compiled and investigated.
Therefore, this study aimed to assess the nutritional and toxicological characteristics of S. latissima and three species of Ulva; Ulva rigida, Ulva fenestrata and Ulva intestinalis, based on data from the scientific literature. Furthermore, to provide new insights on the potential role of seaweed in future sustainable diets, the nutritional profiles of S. latissima and Ulva species were compared with reference foods which seaweeds could serve as alternatives to in the human diet.
Materials and methods
Literature search
Scientific original articles providing information on the content and/or bioavailability of nutrients and heavy metals in Saccharina latissima, Ulva fenestrata, Ulva intestinalis and Ulva rigida were identified by literature search using the databases Scopus, SciFinder and PubMed (details on the search strategies are specified in the Supplementary material Table S1a,b) and were further cross-checked with references from the Harvard Aquatic Food Composition Database[Citation18] (assessed October–November 2021). Articles included in this review are those published in peer-reviewed scientific journals between 2011 and October 2021, written in English. Articles were included if they reported content of one or more nutrient(s) or one or more heavy metal(s), based on chemical analyses of whole and full-grown biomass, of one or more of the selected seaweed specie(s). In case studies comprised dried biomass, they were included regardless of the drying-technique, e.g., freeze-drying, air-drying, sun-drying, or oven-drying. Studies were also included if they investigated bioavailability of one or more nutrient(s) in any of the selected seaweed species, either in studies on humans, monogastric mammals, or in in-vitro models. The following exclusion criteria were defined: (i) papers on cosmetic, pharmaceutical, or agricultural applications of seaweed; (ii) seaweed harvested in locations with reported or determined contamination (as areas with contamination were assumed not to be representative for an average production site); (iii) papers only reporting data on seedlings, parts of the thallus or on extracts of the selected seaweed species; (iv) papers presenting data in a format that could not be harmonized to content per 100 g dry weight (DW) of seaweed; (v) papers investigating bioavailability of nutrient(s) in ruminants or non-mammals. All articles identified (after removal of duplicates) were screened based on information in titles and abstracts and those that were judged to meet the inclusion criteria were reviewed in full text.
Data source, harmonization and reporting
In this review, content of energy, macronutrients, minerals and heavy metals, and information on bioavailability of nutrients, were retrieved from the original scientific studies identified through the literature search. Harvest location and harvest season were screened for in all articles included. Data on Ulva spp. were extracted whenever it was identified in the retrieved original articles. In this review, three kinds of data for Ulva are revealed; (1) data for the individual targeted species (U. fenestrata, U. rigida and U. intestinalis); (2) data for Ulva spp., meaning data reported for unknown Ulva species, and (3) data for “Ulva all,” meaning merged data reported for all the three selected Ulva species and Ulva spp. The term “Ulva species” is used whenever referring to Ulva genus. Literature regarding vitamin content in the investigated seaweed species was found to be scarce.[Citation10] Therefore, data on vitamins for S. latissima and Ulva spp. were retrieved from a publicly accessible food composition database published by Centre for the Study and Valorization of Algae (CEVA)[Citation11,Citation19] (accessed October 2021 and March 2022). Content of nutrients and heavy metals was harmonized to the unit “content per 100 g DW.” A portion size of 5 g DW was used as reference for seaweed consumption as suggested by the European Food Safety Authority (EFSA)[Citation9] and used in the CEVA database.[Citation20] Data is reported as mean and median (min-max).
Regarding protein, fat, carbohydrates and fiber, data was included as reported, regardless of method of determination used by the original study. For example, we have used the term “fat” regardless of whether data were reported as total fatty acids or as gravimetrically determined total lipids/total fat. For protein, data on crude protein content were included independently of the nitrogen-to-protein conversion factor used. Only when nitrogen content was reported, a conversion factor of 5.0 was used to convert it to crude protein, as suggested by Angell et al..[Citation21]
Nutritional quality assessments
Nutritional quality was assessed as the percentage contribution of one portion (5 g DW) of seaweed to Dietary Reference Intakes (DRI) of selected nutrients, as defined by the Nordic Nutrition Recommendations[Citation22] (Supplementary material, Table S2). In addition, the nutrient density of seaweeds and reference foods was assessed by using the index Nutrient Rich Foods (NRF), conceptualized by Drewnowski[Citation23] (Equation 1).
where x indicates the number of nutrients to encourage and y the number of nutrients to limit, nutrient i/j describes the content of nutrient i or nutrient j per reference unit, and MRI is the Maximum Recommended Intake for the non-desirable nutrient (Supplementary material, Table S2).
In this review, the nutrient density was calculated according to NRF21.3 which has been applied to seafood elsewhere.[Citation24,Citation25] This NRF index includes 21 nutrients to encourage (protein, dietary fiber, n-3 fatty acids, calcium, iron, iodine, magnesium, phosphorus, potassium, selenium, zinc, vitamin A, vitamin B6, folate, thiamine, riboflavin, niacin, vitamin B12, vitamin C, vitamin D, vitamin E) and three nutrients to limit (sodium, saturated fat, added sugars). Content of n-3 fatty acids was assessed as the sum of available data for alpha-linolenic acid (ALA), eicosapentaenoic acid (EPA) and docosahexaenoic acid (DHA), even when one or two of them were missing.
Here, NRF21.3 was calculated using portion size as reference amount to reflect differences in the dietary role between compared foods. Capping was applied when nutrients exceeded DRI per portion size (for details on capped nutrients, please refer to Supplementary material Figure S1). Briefly, capping is used to avoid over-crediting nutrient contents that exceed their DRIs by rounding off their nutrient content per reference unit to 100% of DRI. Although earlier research suggests that capping might not be necessary when comparing the nutritional quality of foods across food groups,[Citation26] the use of capping may be justified in cases where one or few nutrients are largely exceeding the DRI, as is the case for e.g., iodine in S. latissima and the targeted Ulva species. However, both capping and the number as well as choice of nutrients may affect the nutrient density, therefore we conducted a sensitivity analysis including NRF21.3 without capping and a second variant of the index (NRF11.3 based on 11 nutrients to encourage) (Supplementary material Table S2). The NRF11.3 index has been suggested as useful, in particular, when comparing foods across different food groups.[Citation26]
Assessment of toxicological risk
The potential toxicological risks of heavy metals, vitamins, and minerals were assessed as percentage contribution of the content in one portion (5 g DW) relative to the tolerable daily intake (%TDI). Tolerable daily intake for heavy metals was defined as either one-seventh of the tolerable weekly intake (TWI) or, when TWI was not available, as the benchmark dose lower confidence limits (BMDL), as defined by EFSA.[Citation27–30] TWI estimates the amount per unit body weight of a potentially harmful substance that can be ingested weekly over a lifetime without risk of adverse health effects. BMDL is presented whenever a tolerable intake cannot be determined and represents the minimum dose that gives a clear, low-level health risk, usually in the range of 1–10% of a particular adverse health effect. If different BMDLs were available for a specific substance, e.g., one for adults and one for children, the lowest level was used. The contents of minerals and vitamins per portion (5 g DW) of seaweed were assessed against the daily upper limits (UL) as defined by NNR[Citation22] when such a limit is defined. The TWI, BMDL, and UL for heavy metals and nutrients are specified in the Supplementary material (Table S3).
Reference foods
Depending on species, seaweed could be seen as a carbohydrate, a protein, salad, or spice alternative on the plate. Until the dietary role of different seaweed species is more clearly defined, it is useful to compare their nutritional performance to different food categories. The %DRI and nutrient density of one portion (5 g DW) of seaweeds were compared to selected reference foods within four different food groups: herbs (basil, dill, parsley, and chives), salad components (lettuce, kale, broccoli, spinach), legumes (chickpeas, peas, red beans, and soybeans) and fish/shellfish (herring, salmon, cod, and oysters). Nutrient content of the reference foods was obtained from the Swedish Food Composition database[Citation31](Supplementary material, Table S4a-c). Portion sizes were retrieved from the software Dietist NetPro (version 20,230,111). Comparisons of %DRI per portion of seaweed and reference foods were reported for nutrients that contributed to at least 15% of DRI per 5 g DW (mean or median) of S. latissima and/or Ulva all.
Results
Search results
In total, 245 articles were assessed in full text, whereof 114 articles were included in this review (, ).[Citation9,Citation32–142] Of these 114 articles, 45 contained data for S. latissima and 82 contained data for Ulva species (U. fenestrata n = 35; U. intestinalis n = 23, U. rigida n = 30 and/or Ulva spp. n = 6) (i.e., some articles contained data for more than one species). The majority (n = 105) of the included studies contained information on nutrient content of one or several of the selected seaweed species, while fewer studies (n = 37) contained information on heavy metals and only two studies provided information on bioavailability aspects (). For S. latissima, almost all data reported were for samples harvested in Europe (n = 44), except for two studies in North America. Studies on Ulva species reported data from harvest sites in Europe (n = 34), Asia (n = 20), Africa (n = 7), and South America (n = 3). Included studies represent samples harvested from January to December. Excluded studies and reasons for exclusion are listed in the Supplementary material (Table S5).
Table 1. Overview of included articles (n = 114).
Nutrient content and nutritional performance of S. latissima and Ulva species
Nutrient content: Content of energy, macronutrients, minerals, and trace elements per 100 g DW of S. latissima and Ulva species is presented in . Comparing the mean macronutrient content of S. latissima and Ulva all showed that S. latissima was higher in EPA and DHA while it was lower in protein, saturated fatty acids and ALA. The mean content of total carbohydrate, fiber, and fat were within similar ranges for S. latissima and Ulva all. Eight studies reported soluble fibers separately, indicating that soluble fiber varies between 30% and 70% of the total fiber content in S. latissima[Citation70,Citation92,Citation115] and between 20% and 60% of total fiber in Ulva species[Citation41,Citation70,Citation92,Citation100,Citation115,Citation134] (data not shown).
Table 2. Content of energy, macronutrients, minerals and trace elements for dried unprocessed S. latissima and Ulva species per 100 g dry weight. Data presented as mean//median (min-max).1
Regarding minerals and trace elements, the mean content of calcium, iodine, phosphorus, and potassium was higher in S. latissima, while this species was lower in iron, magnesium, and selenium, compared to Ulva all (). For some minerals and trace elements, the content varied substantially between different Ulva species. For example, U. intestinalis had a higher mean content of iodine, iron, and potassium, while U. rigida had a higher mean content of selenium, compared to the other Ulva species. Discrepancies between mean and median values were found for some nutrients; e.g., median content of iron was lower compared to mean content in S. latissima, U. intestinalis, and U. fenestrata while median content of selenium and zinc was substantially higher compared to mean content in U. intestinalis.
Based on data from the CEVA food composition database[Citation11,Citation19]S. latissima was lower in vitamin A, riboflavin, niacin, vitamin B6, B12, and C, compared to Ulva spp., the difference being most pronounced for vitamin B12 (). Only vitamin E and folate were higher in S. latissima compared to Ulva spp. The content of vitamin D and thiamine was similar in S. latissima and Ulva spp.
Table 3. Content per 100 g DW and percentage contribution to daily reference intake (%DRI) per portion (5 g DW) of vitamins for dried unprocessed S. latissima and Ulva spp. Data presented as mean (min-max).1
Nutrition quality in relation to recommended intake: Percentage contribution to DRI for energy, macronutrients, minerals, and trace elements per portion (5 g DW) of S. latissima and Ulva species is presented in . For most macronutrients, the mean %DRI per portion was lower than 1%, for S. latissima and the targeted Ulva species (). Only for fiber, the mean %DRI reached higher levels, varying between 3% and 6% per portion. Among minerals and trace elements, iodine stands out with the highest mean %DRI per portion (12600% for S. latissima and 279% for Ulva all), followed by iron (23% for S. latissima and 67% for Ulva all). In addition, calcium, magnesium, and selenium exceeded 15% of DRI, for at least one seaweed species (mean or median) (). Among the vitamins, only vitamin B12 exceeded 15% of DRI, in at least one of the seaweeds (7% in S. latissima and 34% in Ulva spp) ().
Table 4. Percentage contribution to daily reference intake (%DRI) for energy, macronutrients, minerals, and trace elements from dried unprocessed S. latissima, and Ulva species, per portion (5 g dry weight). Data is presented as mean//median (min-max).1.
Nutrition quality in relation to reference foods: Comparison of the %DRI of those nutrients exceeding 15% (mean or median) per portion of S. latissima and/or Ulva all to content in reference foods is illustrated in . Mean %DRI contribution from the seaweeds was commonly higher compared to reference foods. However, legumes showed a higher contribution of calcium compared to Ulva all, as well as higher contribution of magnesium and iron compared to S. latissima. In addition, fish/shellfish showed higher contribution of selenium and vitamin B12 per portion, compared to both S. latissima and Ulva all.
Nutrient density, assessed as NRF21.3 per portion with capping, was higher for Ulva all compared to S. latissima (). Both S. latissima and Ulva all had a higher nutrient density than herbs and salad components and a lower nutrient density compared to fish/shellfish and legumes. More detailed information on the contribution of different nutrients to NRF21.3 per portion with capping (Table S6) and the relative ranking of the seaweeds and individual reference food items, when applying different versions of the NRF index (capped vs uncapped, NRF21.3 vs NRF11.3) (Figure S1), are provided in the Supplementary material. When uncapped values were used instead of capped values, S. latissima ranked substantially higher than Ulva all and all reference foods, explained by the very high iodine content of S. latissima. Applying NRF11.3 per portion with capping (instead of NRF21.3) did not change the overall pattern, compared to .
Figure 2. Percentage contribution to daily reference intakes (%DRI) (y-axis) of selected nutrients from one portion of seaweed and selected reference food groups. Portion size for seaweed is 5 g DW. Fish/shellfish includes raw salmon (125 g), herring (125 g), cod (125 g) and oysters (70 g). Salad components includes fresh broccoli (75 g), kale (70 g), lettuce (40 g) and spinach (30 g). Herbs includes fresh basil, chives, dill, parsley (all 5 g). Legumes includes dry red beans, chickpeas, peas and soya beans (all 80 g). Bars illustrates mean, the minimum and maximum values are illustrated by the black line for each bar.
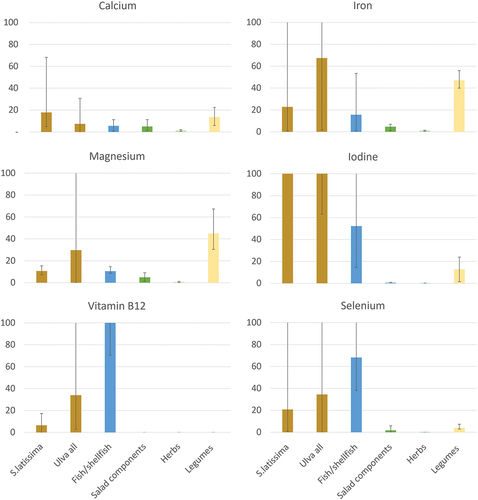
Figure 3. NRF21.3 per portion with capping for S. latissima, Ulva all and selected reference food groups. Portion sizes for seaweeds are 5 g DW. Fish/shellfish includes raw salmon (125 g), herring (125 g), cod (125 g) and oysters (70 g). Salad components includes fresh broccoli (75 g), kale (70 g), lettuce (40 g) and spinach (30 g). Herbs includes fresh basil, chives, dill, parsley (all 5 g). Bars illustrates mean, the minimum and maximum values are illustrated by the black line for each bar.
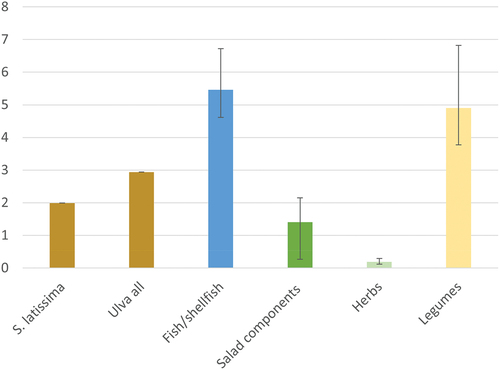
Content of heavy metals and toxicological risk assessment
Content of heavy metals per 100 g DW of S. latissima and Ulva species is presented in . S. latissima had a higher mean content of total arsenic than Ulva all, while the opposite was found for inorganic arsenic. However, only few data points were identified for inorganic arsenic in the targeted Ulva species, with large variation. Ulva all was also higher in lead and mercury. None of the included studies reported inorganic mercury (iHg) or methylmercury (MeHg), the two forms of mercury that can lead to adverse health effects.[Citation30] For cadmium, the mean content was slightly higher in S. latissima than in Ulva all. Comparing mean and median content of heavy metals showed large discrepancies for cadmium and lead in all species except Ulva spp, and for mercury in U. fenestrata.
Table 5. Content of heavy metals for dried unprocessed S. latissima and Ulva species per 100 g dry weight. Data is presented as mean//median (min-max).1.
The percentage contribution to daily tolerable intake (%TDI) of heavy metals and iodine from one portion (5 g DW) of seaweed is presented in . For heavy metals, the mean %TDI per portion generally varied between appr. 10–30%, except for lead in U. intestinalis reaching appr. 50% and inorganic arsenic in Ulva spp. reaching almost 100%. For iodine, the content in one portion of S. latissima exceeded the TDI more than 30 times (mean), while Ulva all contributed to appr. 70% of TDI, per portion.
Table 6. Percentage contribution to tolerable daily intake (%TDI) of heavy metals and iodine for dried unprocessed S. latissima and Ulva species per portion (5 g dry weight). Data is presented as mean//median (min-max).1
Bioavailability
Only two studies investigating the bioavailability of nutrients or heavy metals in the targeted seaweed species were identified and included in this review. Flores et al.[Citation57] used the Caco-2 cell model to assess iron bioavailability in U. fenestrata after in vitro gastrointestinal digestion. The relative fractional uptakes of iron were similar from U. fenestrata and spinach and was suggested to be lower than 7% for both. Addition of physiological levels of vitamin C increased the iron bioavailability of U. fenestrata more than three-fold, while addition of vitamin C did not enhance iron bioavailability of other seaweed species.[Citation57]
The effect of vitamin C on iron bioavailability from spinach was not assessed by Flores et al.,[Citation57] but a 2-fold enhancement has previously been reported.[Citation143]
Trigo et al.[Citation131] assessed protein digestibility and amino acid bioavailability of U. fenestrata and S. latissima, also using the Caco-2 cell model following in vitro gastrointestinal digestion. The protein digestibility of crude U. fenestrata was similar as for control casein, while the protein digestibility of S. latissima was statistically significantly lower compared to casein (17% vs 33%) and to U. fenestrata (28%). The amino acid bioavailability was similar between crude S. latissima (8.5%), crude U. fenestrata (7.3%) and casein (7.6%) with no statistical differences detected.
Discussion
Based on information retrieved from 114 scientific articles and the CEVA database, this review summarizes the content and bioavailability of nutrients and heavy metals in S. latissima, U. fenestrata, U. intestinalis, U. rigida, and Ulva spp. Overall, our findings show that one portion of S. latissima or Ulva species can provide nutritionally relevant contributions (>15% of DRI) to the intake of iron, selenium, calcium (S. latissima) and magnesium (Ulva species). Ulva species may also be a source of vitamin B12, even though it remains unknown if this vitamin in Ulva is biologically active in humans.[Citation144] Further, it was observed that none of the seaweeds included in this study provide nutritionally meaningful amounts per portion of most other nutrients, including protein, fat, and fiber. An exception is iodine, for which the content raises toxicological concerns, especially for S. latissima, which can contribute to more than 30 times the UL of iodine, per portion. The content of inorganic arsenic, lead, and cadmium may also constitute a risk for health if seaweeds, especially Ulva species, would be consumed frequently. The review showed that research on vitamin content and bioavailability of nutrients and toxic substances in S. latissima and the targeted Ulva species are very limited and thus represent important knowledge gaps.
Nutritional performance compared to reference foods
A crucial point in the assessment of the nutritional value of seaweed for human consumption is their role in the diet. However, little is known on how consumers in the European and Nordic countries will include seaweed in the diet, which complicates the selection of reference foods for nutritional comparisons. In this review, a selection of different reference food categories was included, to elucidate how the selected seaweed species, perform nutritionally, compared to foods that seaweed may replace or complement. Furthermore, comparisons were made using realistic portion sizes, since these varies between the selected reference foods. For seaweed, the suggested portion size by EFSA is 5 g DW,[Citation9] corresponding to 30–40 g fresh weight, assuming an average DW percentage of 12.5 and 14.5 for S. latissima and Ulva species, respectively.[Citation145] The suggested portion size for seaweed is lower compared to normal portions of protein-rich foods, such as fish/shellfish (70–125 g fresh weight) and legumes (80 g DW, corresponding to appr. 190 g fresh weight), and more comparable to salad components such as iceberg lettuce and spinach (35 g fresh weight). On the contrary, one portion of seaweed may be larger compared to one portion of herbs (5 g fresh weight).
In relation to the reference foods, S. latissima and the targeted Ulva species appear to have both nutritional strengths and limitations. For example, per portion, S. latissima may be a better source of calcium and the selected Ulva species may be a better source of iron, compared to all reference food groups. In addition, the Ulva species may be a better source of magnesium, compared to fish/shellfish, salad components, and herbs, and both S. latissima and Ulva species may be better sources of selenium and vitamin B12 compared to salad components, herbs, and legumes. However, the contribution of vitamin B12 and selenium from one portion of S. latissima and the selected Ulva species is <10% and ≤50%, respectively, of the contribution from one portion of fish/shellfish. For vitamin D, folate, and fiber, the included seaweeds are less interesting sources than fish/shellfish, salad components, and legumes, respectively, per portion. When applying a broader nutritional assessment, based on the nutrient density (assessed as NRF 21.3, capped) per portion, this study showed that the targeted seaweeds were ranked higher than herbs and salad components, but lower than legumes and fish/shellfish.
Toxicological concerns and legal regulations
This study shows that toxicological concerns related to the use of seaweed as food may be raised due to the content of iodine, inorganic arsenic, lead, and cadmium, although it is highly species dependent. Data for iHg and MeHg were missing, so %TDI for these substances can only be estimated theoretically. If total mercury would be either 100% iHg or MeHg, the mean %TDI per portion would be below 5% for both, in both S. latissima and Ulva all (data not shown).
The large variations in content of heavy metals within seaweed species complicate the comparison between data sources. However, for heavy metals, the present study reported higher lead content in S. latissima compared to data compiled from food composition databases (mean/median: under detection limit; max: 47 µg per 100 g)[Citation146] and a recent Nordic report (mean: 32 µg per 100 g).[Citation147] Furthermore, food composition databases indicate much lower level of total mercury in S. latissima (mean/median: under detection limit; max: 0.9 µg per 100 g)[Citation146]compared to our findings. For U. fenestrata, data compiled in this review were 1.5- and 5-times higher mean content of lead and cadmium, respectively, compared to food composition databases[Citation146] and 3-, 4- and 5-times higher mean content of lead, cadmium, and total mercury, respectively, compared to a recent Nordic report (Hogstad et al. 2022). Neither Hornborg et al.[Citation146] nor the Nordic report[Citation147] reported data for other Ulva species.
So far there are no specific regulations in EU or internationally on maximum levels for food hazards in seaweed foods (not including food supplements). However, general legislation on foods applies also for seaweed. For example, for mercury the legislation on maximum residue levels of pesticides in food and feed (EC Regulation No 296/2005) set a maximum level of 0.01 mg per kg wet seaweed. Assuming 12.5% and 14.5% DW for S. latissima and Ulva species, this corresponds to an upper limit of 8.0 and 6.9 µg total mercury per 100 g DW, for S. latissima and Ulva all, respectively. Even though this study did not identify mercury as a major toxicological concern considering the small portion addressed (5 g DW), it shows that the content of total mercury in samples of both S. latissima and Ulva species may exceed the allowed upper level, per 100 g DW. Certain seaweed species may also require approval according to the regulation on novel food (Regulation EC 2015/2283) before they can be used as food. Whole non-fractionated S. latissima and U. fenestrata are not classified as novel food, while novel food status has not yet been decided for the other Ulva species included here.
Protein, fiber, and fat
Based on our results, seaweeds are unlikely to become a major source of protein, fiber, or fat in the diet. The portion sizes that would be required to provide a nutritionally relevant contribution of these nutrients may be a concern from a toxicological perspective. Up-concentrating proteins, fibers, or fat through extraction protocols could however be a promising strategy to produce new food ingredients while reducing the content of specific heavy metals such as cadmium.[Citation148]
As a source of protein, the Ulva species are more interesting than S. latissima, due to higher protein content, that may reach similar levels as legumes.[Citation149] Similar ratios of essential amino acids to non-essential amino acids as for soybean flour have been reported for both Ulva spp. and S. latissima.[Citation115] According to this review, S. latissima and Ulva species appear to have similar potential as provider of total fiber and fat. However, S. latissima has a greater proportion of soluble fiber (30–70% vs 20–60% of total fiber) and long-chain n-3 fatty acids (50–90% vs 10–30% of the total n-3 fatty acids), compared to the Ulva species.
Bioavailability
Health impact of foods depends not only on the content but also on the bioavailability and biological activity of nutrients and other substances. This can vary greatly between different food items, depending on e.g., its ingredients and its specific matrix structure. As far as we know, the bioavailability of nutrients and heavy metals in S. latissima and the selected Ulva species has yet to be addressed through human clinical trials. However, two in vitro studies on this matter were identified.[Citation57,Citation131] Flores et al.[Citation57] concluded that Caco-2 cell uptake of iron from U. fenestrata is similar to that of spinach and can be further enhanced by addition of vitamin C. The second study concluded that amino acid bioavailability of S. latissima and U. fenestrata was equal to that of casein.[Citation131] Interestingly, Trigo et al.[Citation131] reported a higher in vitro protein digestibility in U. fenestrata compared to S. latissima. Factors such as the amount of cell wall-bound protein and fiber content can influence the access of digestive proteases to seaweed proteins, thus explaining the different digestibility values between species.[Citation150,Citation151] Also, levels of antinutrients, such as polyphenols, play an important role. A recent review article concluded that iodine bioavailability for Saccharina species varies between 57% and 71%.[Citation152–158] Regarding vitamin B12, both biologically active and inactive forms may be present in foods. Whether vitamin B12 in S. latissima and Ulva species contains biologically active vitamin B12 or not, remains to be elucidated.[Citation144]
Strengths, limitations, method considerations
This study collected nutritional data for S. latissima, U. rigida, U. intestinalis, and U. fenestrata, with complementary data on Ulva spp., allowing to assess the nutritional composition, the nutritional quality, content of potentially toxic elements and bioavailability aspects. Nutritional assessments were performed with multiple methods (%DRI and nutrient density index NRF, in comparison to reference foods), thus strengthening the conclusions of the nutritional performance of the seaweeds. However, our review only provided information for four seaweed species whose properties may differ from other edible species. Additionally, the impact of harvest season and geographical location on content of nutrients and heavy metals was not the focus of this review, but would be useful additional information to guide toward optimal future seaweed production systems.
Another limitation was that nutritional and toxicological assessments were made without taking into account possible impact of post-harvest processing techniques, such as blanching. Despite blanching being an effective technique at reducing iodine, it significantly changes total amino acid content and total monosaccharide content,[Citation148] thus likely changing the nutritional quality of the original biomass. Finally, as stated in the methods section, we have included data as reported in the included original studies, without adjusting for any differences in analysis methods. Depending on method, the content of some nutrients may however be under- or overestimated. For example, some studies reported crude fiber, which represents only fractions of insoluble fiber and no soluble fibers, meaning that fiber content, and thereby %DRI of fiber, may be underestimated. For carbohydrate, there is the opposite risk, since many studies report total carbohydrate, including indigestible carbohydrates, while DRI for carbohydrates refers to digestible carbohydrates only. Furthermore, the fat content can be overestimated if determined via solvent extraction and gravimetric determination, but slightly underestimated if done via fatty acid analysis. For protein, the choice of nitrogen-to-protein conversion factor may lead to slight under- or overestimations. However, the main conclusions of this review remain, independently of the possible misestimations of macronutrient content.
Future perspectives
To support the development of nutritious and healthy foods from S. latissima and the selected Ulva species, it would be useful with more studies on the content of aqueous and lipophilic vitamins and on how different harvest season and harvesting location impact the content of nutrients and heavy metals. Further investigations on how different post-harvest processing techniques influence nutritional and toxicological characteristics of the included seaweeds would also be useful. This is because it would help identifying processing parameters for maximal reduction of heavy metals and iodine, in parallel with maintenance or improved nutritional content and quality, including bioavailability. Besides blanching, such processing could comprise rinsing, ensilaging, fermentation, brining, freeze-thawing, together with upcoming and established methods to concentrate e.g., protein, specific polysaccharides, and phenolic compounds. Furthermore, better understanding of the dietary role of S. latissima and Ulva species, e.g., portion sizes and which food items these species can replace or complement on the plate, is crucial to better assess their nutritional contribution and toxicological risks as food. Randomized controlled clinical trials are also necessary to clarify the bioavailability of nutrients and heavy metals in foods based on Ulva and S. latissima or with these species as ingredients. Other aspects to explore are the presence of allergens and microbiological hazards. Finally, based on our findings, we call for a shift from considering “seaweed” as a single food commodity to address it by the individual species; similarly, to fruits (e.g., apple and orange) or vegetables (e.g., cabbage and spinach). Future studies, on the above-mentioned perspectives, will be useful for seaweed producers and seaweed food manufacturers, and not the least as guidance to the EU Commission, soon expected to evaluate the need for risk management actions targeted to different seaweed species as foods in EU.[Citation147]
Conclusion
Data compiled in this review show that one portion of whole dried S. latissima and selected Ulva species (U. rigida, U. fenestrata, and U. intestinalis) may be nutritionally meaningful sources of some micronutrients, while their nutritional contribution of macronutrients is small. For iron, magnesium, selenium, calcium (S. latissima) and vitamin B12 (Ulva species) one portion of seaweed may contribute to ≥15% of recommended daily intake. The content of iodine was identified as the main toxicological concern, especially for S. latissima. The content of inorganic arsenic, lead, and cadmium may also constitute a health risk, especially for Ulva species, if consumed frequently. The considered seaweed species were shown to have both nutritional strengths and limitations compared to selected reference foods. Based on a nutrient density index score taking 24 nutrients into account, the two species ranked higher than herbs and salad components, and lower than legumes and fish/shellfish. Bioavailability data were scarce and is an important future research aspect to clarify the nutritional quality of different seaweeds. Further work is also warranted regarding the biological activity of vitamin B12 and impact of post-harvest processing on nutrients and heavy metals. In conclusion, the results from this review indicate that S. latissima and Ulva species may provide a meaningful contribution of some micronutrients often limited in plant-based foods; however, toxicological concerns especially related to high iodine content may restrict the intake levels that can be considered safe. Ultimately, the nutritional potential of the addressed seaweeds will depend on their role in future diets and this review provides new insights on what their nutritional impacts could become, depending on intake levels and foods replaced.
Author contributions
M. Jacobsen: Conceptualization; Methodology; Data collection; Literature search; Writing original draft, review, and editing. M. Bianchi: Conceptualization; Methodology; Data collection; Writing – original draft, review, and editing. J. P. Trigo: Conceptualization; Methodology; Writing – review and editing. I. Undeland: Conceptualization; Methodology; Data collection; Literature search; Writing – original draft, review, and editing. E. Hallström: Conceptualization; Methodology; Data collection; Literature search; Writing – original draft, review and editing; Funding acquisition. S. Bryngelsson: Conceptualization; Methodology; Data collection; Literature search; Writing – original draft, review, and editing. All authors have read and agreed to the published version of the manuscript.
Acknowledgments
Assoc. Prof. Sabine Samples, SLU, Uppsala, Sweden, is acknowledged for supervision of M.J. during her Master Thesis, forming the basis for this publication. Prof. Anders Glynn, SLU, Uppsala, Sweden, is acknowledged for consultations regarding toxicological evaluation.
Disclosure statement
No potential conflict of interest was reported by the author(s).
Additional information
Funding
References
- Araújo, R.; Vázquez Calderón, F.; Sánchez López, J.; Azevedo, I. C.; Bruhn, A.; Fluch, S.; Garcia Tasende, M.; Ghaderiardakani, F.; Ilmjärv, T.; Laurans, M., et al. Current Status of the Algae Production Industry in Europe: An Emerging Sector of the Blue Bioeconomy. Front. Mar. Sci. 2021, 7, 1247. DOI: 10.3389/fmars.2020.626389.
- Mendes, M. C.; Navalho, S.; Ferreira, A.; Paulino, C.; Figueiredo, D.; Silva, D.; Gao, F.; Gama, F.; Bombo, G.; Jacinto, R., et al. Algae as Food in Europe: An Overview of Species Diversity and Their Application. Foods. 2022, 11(13). DOI: 10.3390/foods11131871.
- Cai, J.; Lovatelli, A.; Aguilar-Manjarrez, J.; Cornish, L.; Dabbadie, L.; Desrochers, A.; Diffey, S.; Garrido Gamarro, E.; Geehan, J.; Hurtado, A. Seaweeds and Microalgae: An Overview for Unlocking Their Potential in Global Aquaculture Development. FAO Fish. Aquac Circular. 1229. DOI: 10.4060/cb5670en.
- Parodi, A.; Leip, A.; De Boer, I. J. M.; Slegers, P. M.; Ziegler, F.; Temme, E. H. M.; Herrero, M.; Tuomisto, H.; Valin, H.; Van Middelaar, C. E., et al. The Potential of Future Foods for Sustainable and Healthy Diets. Nat. Sustain. 2018, 1(12), 782–789. DOI: 10.1038/s41893-018-0189-7.
- Gephart, J. A.; Henriksson, P. J. G.; Parker, R. W. R.; Shepon, A.; Gorospe, K. D.; Bergman, K.; Eshel, G.; Golden, C. D.; Halpern, B. S.; Hornborg, S., et al. Environmental Performance of Blue Foods. Nature. 2021, 597(7876), 360–365. DOI: 10.1038/s41586-021-03889-2.
- Cherry, P.; O’Hara, C.; Magee, P. J.; McSorley, E. M.; Allsopp, P. J. Risks and Benefits of Consuming Edible Seaweeds. Nutr. rev. 2019, 77(5), 307–329. DOI: 10.1093/nutrit/nuy066.
- Nova, P.; Martins, A. P.; Teixeira, C.; Abreu, H.; Silva, J. G.; Silva, A. M.; Freitas, A. C.; Gomes, A. M. Foods with Microalgae and Seaweeds Fostering Consumers Health: A Review on Scientific and Market Innovations. J. Appl. Phycol. 2020, 32(3), 1789–1802. DOI: 10.1007/s10811-020-02129-w.
- Lozano Muñoz, I.; Díaz, N. F. Minerals in Edible Seaweed: Health Benefits and Food Safety Issues. Crit. Rev. Food Sci. Nutr. 2022, 62(6), 1592–1607. DOI: 10.1080/10408398.2020.1844637.
- Sá Monteiro, M.; Sloth, J.; Holdt, S.; Hansen, M. Analysis and Risk Assessment of Seaweed. Efsa J. 2019, 17(Suppl 2), e170915. DOI: 10.2903/j.efsa.2019.e170915.
- Kumar, M. S.; Sharma, S. Toxicological Effects of Marine Seaweeds: A Cautious Insight for Human Consumption. Crit. Rev. Food Sci. Nutr. 2020, 61(3), 500–521. DOI: 10.1080/10408398.2020.1738334.
- CEVA [Centre d’Étude et de Valorisation des Algues]. 2021. Ulva Spp - Nutritional Data Sheet. https://www.ceva-algues.com/wp-content/uploads/2021/04/EN-Ulva-spp.pdf. (Assessed March, 2022).
- Vincent, A.; Stanely, A.; Ring, J. Hidden Champion of the Ocean: Seaweed as a Growth Engine for a Sustainable European Future. Seaweed For Europe. https://www.seaweedeurope.com/wp-content/uploads/2020/10/Seaweed_for_Europe-Hidden_Champion_of_the_ocean-Report.pdf (2020).
- MacArtain, P.; Gill, C. I. R.; Brooks, M.; Campbell, R.; Rowland, I. R. Nutritional Value of Edible Seaweeds. Nutr. rev. 2008, 65(12), 535–543. DOI: 10.1111/j.1753-4887.2007.tb00278.x.
- Gamero-Vega, G.; Palacios-Palacios, M.; Quitral, V. Nutritional Composition and Bioactive Compounds of Red Seaweed: A Mini-Review. J. Food Nutr. Res. 2020, 8(8), 431–440. DOI: 10.12691/jfnr-8-8-7.
- Kumar, M. S.; Sharma, S. A. Toxicological Effects of Marine Seaweeds: A Cautious Insight for Human Consumption. Crit. Rev. Food Sci. Nutr. 2021, 61(3), 500–521. DOI: 10.1080/10408398.2020.1738334.
- Circuncisão, A. R.; Catarino, M. D.; Cardoso, S. M.; Silva, A. M. S. Minerals from Macroalgae Origin: Health Benefits and Risks for Consumers. Mar. Drugs. 2018, 16(11). DOI: 10.3390/md16110400.
- Nielsen, C. W.; Rustad, T.; Holdt, S. L. Vitamin C from Seaweed: A Review Assessing Seaweed as Contributor to Daily Intake. Foods. 2021, 10(1). DOI: 10.3390/foods10010198.
- Golden, C. D.; Koehn, J. Z.; Vaitla, B.; DeSisto, C.; Kelahan, H.; Manning, K.; Fiorella, K. J.; Kjellevold, M.; Thilsted, S. H., Aquatic Food Composition Database. 2021, Harvard Dataverse. V2. [ Assessed November 2021].
- CEVA [Centre d’Étude et de Valorisation des Algues]. 2021. Saccharina Latissima - Nutritional Data Sheet. https://www.ceva-algues.com/wp-content/uploads/2021/04/EN-Saccharina-latissima-1.pdf (Assessed March, 2022).
- CEVA [Centre d’Étude et de Valorisation des Algues]. Nutritional Data Sheets On Algae; 2021. https://www.ceva-algues.com/en/document/nutritional-data-sheets-on-algae/ (Accessed March, 2022).
- Angell, A. R.; Mata, L.; de Nys, R.; Paul, N. A. The Protein Content of Seaweeds: A Universal Nitrogen-To-Protein Conversion Factor of Five. J. Appl. Phycol. 2016, 28(1), 511–524. DOI: 10.1007/s10811-015-0650-1.
- Nordic Council of Ministers, Nordic Nutrition Recommendations 2012. Integrating Nutrition and Physical Activity. Copenhagen: Nordic Council of Ministers, 2014. DOI: 10.26530/OAPEN_483279.
- Drewnowski, A. Defining Nutrient Density: Development and Validation of the Nutrient Rich Foods Index. J. Am. Coll. Nutr. 2009, 28(4), 421S–426S. DOI: 10.1080/07315724.2009.10718106.
- Hallström, E.; Bergman, K.; Mifflin, K.; Parker, R.; Tyedmers, P.; Troell, M.; Ziegler, F. Combined Climate and Nutritional Performance of Seafoods. J. Clean. Prod. 2019, 230, 402–411. DOI: 10.1016/j.jclepro.2019.04.229.
- Bianchi, M.; Hallström, E.; Parker, R. W. R.; Mifflin, K.; Tyedmers, P.; Ziegler, F. Assessing Seafood Nutritional Diversity Together with Climate Impacts Informs More Comprehensive Dietary Advice. Commun Earth Environ. 2022, 3(1), 188. DOI: 10.1038/s43247-022-00516-4.
- Bianchi, M.; Strid, A.; Winkvist, A.; Lindroos, A.-K.; Sonesson, U.; Hallström, E. Systematic Evaluation of Nutrition Indicators for Use within Food LCA Studies. Sustainability. 2020, 12(21), 8992. DOI: 10.3390/su12218992.
- EFSA [European Food Safety Authority]. Cadmium in Food ‐ Scientific Opinion of the Panel on Contaminants in the Food Chain. Efsa J., 2009, (980), 1–139. DOI: 10.2903/j.efsa.2009.980.
- EFSA [European Food Safety Authority]. Scientific Opinion on Arsenic in Food. Efsa J. 2009, 7(10), 1351. DOI: 10.2903/j.efsa.2009.1351.
- EFSA [European Food Safety Authority]. Scientific Opinion on Lead in Food. Efsa J. 2010, 8(4), 1570. DOI: 10.2903/j.efsa.2010.1570.
- EFSA [European Food Safety Authority]. Scientific Opinion on the Risk for Public Health Related to the Presence of Mercury and Methylmercury in Food. Efsa J. 2012, 10(12), 2985. DOI: 10.2903/j.efsa.2012.2985.
- Livsmedelsverket [Swedish Food Agency]. 2021. Livsmedelsdatabasen [Food Composition Database], Version 20210503. https://soknaringsinnehall.livsmedelsverket.se (Accessed January 10, 2023).
- Abdollahi, M.; Axelsson, J.; Carlsson, N.-G.; Nylund, G. M.; Albers, E.; Undeland, I. Effect of Stabilization Method and Freeze/thaw-Aided Precipitation on Structural and Functional Properties of Proteins Recovered from Brown Seaweed (Saccharina Latissima). Food Hydrocoll. 2019, 96, 140–150. DOI: 10.1016/j.foodhyd.2019.05.007.
- Afonso, C.; Cardoso, C.; Ripol, A.; Varela, J.; Quental-Ferreira, H.; Pousão-Ferreira, P.; Ventura, M. S.; Delgado, I. M.; Coelho, I.; Castanheira, I., et al. Composition and Bioaccessibility of Elements in Green Seaweeds from Fish Pond Aquaculture. Food. Res. Int. 2018, 105, 271–277. DOI: 10.1016/j.foodres.2017.11.015.
- Afonso, C.; Matos, J.; Guarda, I.; Gomes-Bispo, A.; Gomes, R.; Cardoso, C.; Gueifão, S.; Delgado, I.; Coelho, I.; Castanheira, I., et al. Bioactive and Nutritional Potential of Alaria Esculenta and Saccharina Latissima. J. Appl. Phycol. 2021, 33(1), 501–513. DOI: 10.1007/s10811-020-02298-8.
- Akalya, K.; Kumar, S. D.; Manigandan, G.; Santhanam, P.; Perumal, P.; Krishnaveni, N.; Arthikha, R.; Begum, A.; Dhanalakshmi, B.; Kim, M.-K. The Influence of the Macroalgae Liquid Extracts on the Pigments and Fatty Acids Profile of the Marine Microalga, Picochlorum Maculatum (PSDK01). Thalassas. 2021, 38(1), 1–12. DOI: 10.1007/s41208-021-00338-9.
- Akköz, C.; Arslan, D.; Ünver, A.; Özcan, M.; Yilmaz, B. Chemical Composition, Total Phenolic and Mineral Contents of Enteromorpha Intestinalis (L.) Kütz. and Cladophora Glomerata (L.) Kütz. Seaweeds. J. Food Biochem. 2011, 35(2), 513–523. DOI: 10.1111/j.1745-4514.2010.00399.x.
- Bak, U. G.; Nielsen, C. W.; Marinho, G. S.; Gregersen, Ó.; Jónsdóttir, R.; Holdt, S. L. The Seasonal Variation in Nitrogen, Amino Acid, Protein and Nitrogen-To-Protein Conversion Factors of Commercially Cultivated Faroese Saccharina Latissima. Algal Res. 2019, 42. DOI: 10.1016/j.algal.2019.101576.
- Barbosa, M.; Fernandes, F.; Pereira, D. M.; Azevedo, I. C.; Sousa-Pinto, I.; Andrade, P. B.; Valentão, P. Fatty Acid Patterns of the Kelps Saccharina Latissima, Saccorhiza Polyschides and Laminaria Ochroleuca: Influence of Changing Environmental Conditions. Arab J. Chem. 2020, 13(1), 45–58. DOI: 10.1016/j.arabjc.2017.01.015.
- Batista, S.; Pintado, M.; Marques, A.; Abreu, H.; Silva, J. L.; Jessen, F.; Tulli, F.; Valente, L. M. Use of Technological Processing of Seaweed and Microalgae as Strategy to Improve Their Apparent Digestibility Coefficients in European Seabass (Dicentrarchus Labrax) Juveniles. J. Appl. Phycol. 2020, 32(5), 3429–3446. DOI: 10.1007/s10811-020-02185-2.
- Beacham, T. A.; Cole, I. S.; DeDross, L. S.; Raikova, S.; Chuck, C. J.; Macdonald, J.; Herrera, L.; Ali, T.; Airs, R. L.; Landels, A., et al. Analysis of Seaweeds from South West England as a Biorefinery Feedstock. Appl. Sci. 2019, 9(20), 4456. DOI: 10.3390/app9204456.
- Benjama, O.; Masniyom, P. Nutritional Composition and Physicochemical Properties of Two Green Seaweeds (Ulva Pertusa and U. Intestinalis) from the Pattani Bay in Southern Thailand. Songklanakarin J. Sci. Technol. 2011, 33(5), 575–583.
- Berik, N.; Cankiriligil, E. C. The Elemental Composition of Green Seaweed (Ulva Rigida) Collected from Çanakkale, Turkey. Aquat. Sci. Eng. 2019, 34(3), 74–79. DOI: 10.26650/ASE2019557380.
- Biancarosa, I.; Espe, M.; Bruckner, C. G.; Heesch, S.; Liland, N.; Waagbø, R.; Torstensen, B.; Lock, E. J. Amino Acid Composition, Protein Content, and Nitrogen-To-Protein Conversion Factors of 21 Seaweed Species from Norwegian Waters. J. Appl. Phycol. 2017, 29(2), 1001–1009. DOI: 10.1007/s10811-016-0984-3.
- Bikker, P.; Stokvis, L.; van Krimpen, M. M.; van Wikselaar, P. G.; Cone, J. W. Evaluation of Seaweeds from Marine Waters in Northwestern Europe for Application in Animal Nutrition. Anim. Feed Sci. Technol. 2020, 263. DOI: 10.1016/j.anifeedsci.2020.114460.
- Blikra, M. J.; Løvdal, T.; Vaka, M. R.; Roiha, I. S.; Lunestad, B. T.; Lindseth, C.; Skipnes, D. Assessment of Food Quality and Microbial Safety of Brown Macroalgae (Alaria Esculenta and Saccharina Latissima). J. Sci. Food Agr. 2019, 99(3), 1198–1206. DOI: 10.1002/jsfa.9289.
- Bogolitsyn, K. G.; Kaplitsin, P. A.; Pochtovalova, A. S. Amino-Acid Composition of Arctic Brown Algae. Chem. Nat. Comp. 2014, 49(6), 1110–1113. DOI: 10.1007/s10600-014-0831-1.
- Cabrita, A. R. J.; Maia, M. R. G.; Oliveira, H. M.; Sousa-Pinto, I.; Almeida, A. A.; Pinto, E.; Fonseca, A. J. M. Tracing Seaweeds as Mineral Sources for Farm-Animals. J. Appl. Phycol. 2016, 28(5), 3135–3150. DOI: 10.1007/s10811-016-0839-y.
- Cañedo-Castro, B.; Piñón-Gimate, A.; Carrillo, S.; Ramos, D.; Casas-Valdez, M. Prebiotic Effect of Ulva Rigida Meal on the Intestinal Integrity and Serum Cholesterol and Triglyceride Content in Broilers. J. Appl. Phycol. 2019, 31, 3265–3273. DOI: 10.1007/s10811-019-01785-x.
- Correia, H.; Soares, C.; Morais, S.; Pinto, E.; Marques, A.; Nunes, M. L.; Almeida, A.; Delerue-Matos, C. Seaweeds Rehydration and Boiling: Impact on Iodine, Sodium, Potassium, Selenium, and Total Arsenic Contents and Health Benefits for Consumption. Food. Chem. Toxicol. 2021, 155, 112385. DOI: 10.1016/j.fct.2021.112385.
- Costa, M.; Henriques, B.; Pinto, J.; Fabre, E.; Viana, T.; Ferreira, N.; Amaral, J.; Vale, C.; Pinheiro-Torres, J.; Pereira, E. Influence of Salinity and Rare Earth Elements on Simultaneous Removal of Cd, Cr, Cu, Hg, Ni and Pb from Contaminated Waters by Living Macroalgae. Environ. Poll. 2020, 266(Pt 1), 115374. DOI: 10.1016/j.envpol.2020.115374.
- Dammak Walha, L.; Hamza, A.; Abdmouleh Keskes, F.; Cibic, T.; Mechi, A.; Mahfoudi, M.; Sammari, C. Heavy Metals Accumulation in Environmental Matrices and Their Influence on Potentially Harmful Dinoflagellates Development in the Gulf of Gabes (Tunisia). Estuarine Coastal Shelf Sci. 2021, 254, 107317. DOI: 10.1016/j.ecss.2021.107317.
- Díaz, O.; Tapia, Y.; Muñoz, O.; Montoro, R.; Velez, D.; Almela, C. Total and Inorganic Arsenic Concentrations in Different Species of Economically Important Algae Harvested from Coastal Zones of Chile. Food. Chem. Toxicol. 2012, 50(3–4), 744–749. DOI: 10.1016/j.fct.2011.11.024.
- Diop, M.; Howsam, M.; Diop, C.; Goossens, J. F.; Diouf, A.; Amara, R. Assessment of Trace Element Contamination and Bioaccumulation in Algae (Ulva Lactuca), Mussels (Perna Perna), Shrimp (Penaeus Kerathurus), and Fish (Mugil Cephalus, Saratherondon Melanotheron) Along the Senegalese Coast. Mar. Pollut. Bull. 2016, 103(1–2), 339–343. DOI: 10.1016/j.marpolbul.2015.12.038.
- El-Said, G. F. Bioaccumulation of Key Metals and Other Contaminants by Seaweeds from the Egyptian Mediterranean Sea Coast in Relation to Human Health Risk. Hum. Ecol. Risk Assess. 2013, 19(5), 1285–1305. DOI: 10.1080/10807039.2012.708253.
- Escobido, H. R. S.; Orbita, M. L. S.; Orbita, R. R. Evaluation of the Biochemical and Phytochemical Components of Green Seaweed Enteromorpha Intestinalis (Linnaeus) in Initao, Misamis Oriental, Mindanao, Philippines. Int. J. Biosci. 2016, 9, 114–122. DOI: 10.12692/ijb/9.4.114-122.
- Ferreira, J. P.; Sharma, A.; Zannad, F. The Future of Meat: Health Impact Assessment with Randomized Evidence. Am. j. med. 2021, 134(5), 569–575. DOI: 10.1016/j.amjmed.2020.11.007.
- Flores, S. R. L.; Dobbs, J.; Dunn, M. A. Mineral Nutrient Content and Iron Bioavailability in Common and Hawaiian Seaweeds Assessed by an in vitro Digestion/caco-2 Cell Model. J. Food Comp. Anal. 2015, 43, 185–193. DOI: 10.1016/j.jfca.2015.06.008.
- Frikha, F.; Kammoun, M.; Hammami, N.; McHirgui, R. A.; Belbahri, L.; Gargouri, Y.; Miled, N.; Ben-Rebah, F. Chemical Composition and Some Biological Activities of Marine Algae Collected in Tunisia. Ciencias Marinas. 2011, 37(2), 113–124. DOI: 10.7773/cm.v37i2.1712.
- Gaillard, C.; Bhatti, H. S.; Novoa-Garrido, M.; Lind, V.; Roleda, M. Y.; Weisbjerg, M. R. Amino Acid Profiles of Nine Seaweed Species and Their in situ Degradability in Dairy Cows. Anim. Feed Sci. Technol. 2018, 241, 210–222. DOI: 10.1016/j.anifeedsci.2018.05.003.
- Gao, G.; Clare, A.; Rose, C.; Caldwell, G. Reproductive Sterility Increases the Capacity to Exploit the Green Seaweed Ulva Rigida for Commercial Applications. Algal Res. 2017, 24, 64–71. DOI: 10.1016/j.algal.2017.03.008.
- García-Sartal, C.; Romarís-Hortas, V.; Del Carmen Barciela-Alonso, M.; Moreda-Piñeiro, A.; Dominguez-Gonzalez, R.; Bermejo-Barrera, P. Use of an in vitro Digestion Method to Evaluate the Bioaccessibility of Arsenic in Edible Seaweed by Inductively Coupled Plasma-Mass Spectrometry. Microchem. J. 2011, 98(1), 91–96. DOI: 10.1016/j.microc.2010.12.001.
- García-Sartal, C.; Del Carmen Barciela-Alonso, M.; Moreda-Piñeiro, A.; Bermejo-Barrera, P. Study of Cooking on the Bioavailability of As, Co, Cr, Cu, Fe, Ni, Se and Zn from Edible Seaweed. Microchem. J. 2013, 108, 92–99. DOI: 10.1016/j.microc.2012.10.003.
- Ghanemi, K.; Navidi, M.-A.; Fallah-Mehrjardi, M.; Dadolahi-Sohrab, A. Ultra-Fast Microwave-Assisted Digestion in Choline Chloride–Oxalic Acid Deep Eutectic Solvent for Determining Cu, Fe, Ni and Zn in Marine Biological Samples. Anal. Meth. 2014, 6(6), 1774–1781. DOI: 10.1039/C3AY41843J.
- Harrysson, H.; Krook, J. L.; Larsson, K.; Tullberg, C.; Oerbekke, A.; Toth, G.; Pavia, H.; Undeland, I. Effect of Storage Conditions on Lipid Oxidation, Nutrient Loss and Colour of Dried Seaweeds, Porphyra Umbilicalis and Ulva Fenestrata, Subjected to Different Pretreatments. Algal. Res. 2021, 56, 102295. DOI: 10.1016/j.algal.2021.102295.
- Hossain, M. T.; Sohag, A. A. M.; Haque, M. N.; Tahjib-Ul-Arif, M.; Dash, R.; Chowdhury, M. T. H.; Hossain, M. A.; Moon, I. S.; Hannan, M. A. Nutritional Value, Phytochemical Profile, Antioxidant Property and Agar Yielding Potential of Macroalgae from Coasts of Cox’s Bazar and St. Martin’s Island of Bangladesh. J. Aq. Food Prod. Technol. 2021, 30(2), 217–227. DOI: 10.1080/10498850.2020.1869876.
- Ismail, M. M.; El Zokm, G. M.; El-Sayed, A. A. Variation in Biochemical Constituents and Master Elements in Common Seaweeds from Alexandria Coast, Egypt, with Special Reference to Their Antioxidant Activity and Potential Food Uses: Prospective Equations. Env. Monit. Assess. 2017, 189(12), 1–17. DOI: 10.1007/s10661-017-6366-8.
- Jannat-Alipour, H.; Rezaei, M.; Shabanpour, B.; Tabarsa, M. Edible Green Seaweed, Ulva Intestinalis as an Ingredient in Surimi-Based Product: Chemical Composition and Physicochemical Properties. J. Appl. Phycol. 2019, 31, 2529–2539. DOI: 10.1007/s10811-019-1744-y.
- Jara-Marini, M. E.; Molina-García, A.; Martínez-Durazo, Á.; Páez-Osuna, F. Trace Metal Trophic Transference and Biomagnification in a Semiarid Coastal Lagoon Impacted by Agriculture and Shrimp Aquaculture. Environ. Sci. Pollut. Res. 2020, 27(5), 5323–5336. DOI: 10.1007/s11356-019-06788-2.
- Jard, G.; Jackowiak, D.; Carrère, H.; Delgenès, J.-P.; Torrijos, M.; Steyer, J.-P.; Dumas, C. Batch and Semi-Continuous Anaerobic Digestion of Palmaria Palmata: Comparison with Saccharina Latissima and Inhibition Studies. Chem. Eng. J. 2012, 209, 513–519. DOI: 10.1016/j.cej.2012.08.010.
- Jard, G.; Marfaing, H.; Carrère, H.; Delgenes, J. P.; Steyer, J. P.; Dumas, C. French Brittany Macroalgae Screening: Composition and Methane Potential for Potential Alternative Sources of Energy and Products. Biores. Technol. 2013, 144, 492–498. DOI: 10.1016/j.biortech.2013.06.114.
- Jayasinghe, G.; Jinadasa, B.; Chinthaka, S. D. M. Nutritional Composition and Heavy Metal Content of Five Tropical Seaweeds. Open Sci. J. Anal. Chem. 2018, 3(2), 17–22. DOI: 10.1016/j.foodchem.2022.133866.
- Kisten, K.; Moodley, R.; Jonnalagadda, S. B. Elemental Analysis and Nutritional Value of Seaweed from the East Coast of KwaZulu-Natal, South Africa. Anal. Lett. 2017, 50(3), 580–590. DOI: 10.1080/00032719.2016.1182545.
- Kreissig, K. J.; Hansen, L. T.; Jensen, P. E.; Wegeberg, S.; Geertz-Hansen, O.; Sloth, J. J.; Hu, Y. Characterisation and Chemometric Evaluation of 17 Elements in ten Seaweed Species from Greenland. PLoS One. 2021, 16(2), e0243672. DOI: 10.1371/journal.pone.0243672.
- Krogdahl, Å.; Jaramillo-Torres, A.; Ahlstrøm, Ø.; Chikwati, E.; Aasen, I. M.; Kortner, T. M. Protein Value and Health Aspects of the Seaweeds Saccharina Latissima and Palmaria Palmata Evaluated with Mink as Model for Monogastric Animals. Anim. Feed Sci. Technol. 2021, 276. DOI: 10.1016/j.anifeedsci.2021.114902.
- Kumar, M.; Kumari, P.; Trivedi, N.; Shukla, M. K.; Gupta, V.; Reddy, C.; Jha, B. Minerals, PUFAs and Antioxidant Properties of Some Tropical Seaweeds from Saurashtra Coast of India. J. Appl. Phycol. 2011, 23, 797–810. DOI: 10.1007/s10811-010-9578-7.
- Kumar, Y.; Tarafdar, A.; Kumar, D.; Verma, K.; Aggarwal, M.; Badgujar, P. C.; Durazzo, A. Evaluation of Chemical, Functional, Spectral, and Thermal Characteristics of Sargassum Wightii and Ulva Rigida from Indian Coast. J. Food Qual. 2021, 2021, 1–9. DOI: 10.1155/2021/9133464.
- Leri, A. C.; Dunigan, M. R.; Wenrich, R. L.; Ravel, B. Particulate Organohalogens in Edible Brown Seaweeds. Food Chem. 2019, 272, 126–132. DOI: 10.1016/j.foodchem.2018.08.050.
- Machado, M.; Machado, S.; Pimentel, F. B.; Freitas, V.; Alves, R. C.; Oliveira, M. B. P. Amino Acid Profile and Protein Quality Assessment of Macroalgae Produced in an Integrated Multi-Trophic Aquaculture System. Foods. 2020, 9(10), 1382. DOI: 10.3390/foods9101382.
- Mæhre, H. K.; Malde, M. K.; Eilertsen, K. E.; Elvevoll, E. O. Characterization of Protein, Lipid and Mineral Contents in Common Norwegian Seaweeds and Evaluation of Their Potential as Food and Feed. J. Sci. Food Agr. 2014, 94(15), 3281–3290. DOI: 10.1002/jsfa.6681.
- Maia, M. R. G.; Fonseca, A. J. M.; Oliveira, H. M.; Mendonça, C.; Cabrita, A. R. J. The Potential Role of Seaweeds in the Natural Manipulation of Rumen Fermentation and Methane Production. Sci. Rep. 2016, 6(1). DOI: 10.1038/srep32321.
- Maia, M. R. G.; Fonseca, A. J. M.; Cortez, P. P.; Cabrita, A. R. J. In vitro Evaluation of Macroalgae as Unconventional Ingredients in Ruminant Animal Feeds. Algal. Res. 2019, 40, 101481. DOI: 10.1016/j.algal.2019.101481.
- Manns, D.; Nielsen, M. M.; Bruhn, A.; Saake, B.; Meyer, A. S. Compositional Variations of Brown Seaweeds Laminaria Digitata and Saccharina Latissima in Danish Waters. J. Appl. Phycol. 2017, 29(3), 1493–1506. DOI: 10.1007/s10811-017-1056-z.
- Milinovic, J.; Noronha, J.; Diniz, M.; Mata, P.; Mata, P.; Diniz, M.; Noronha, J.; Milinovic, J. Umami Taste in Edible Seaweeds: The Current Comprehension and Perception. Int. J. Gastr. Food Sci. 2021, 23, 100301. DOI: 10.1016/j.ijgfs.2020.100301.
- Monteiro, J. P.; Rey, F.; Melo, T.; Moreira, A. S. P.; Arbona, J. F.; Skjermo, J.; Forbord, S.; Funderud, J.; Raposo, D.; Kerrison, P. D., et al. The Unique Lipidomic Signatures of Saccharina Latissima Can Be Used to Pinpoint Their Geographic Origin. Biomolecules. 2020, 10(1), 107. DOI: 10.3390/biom10010107.
- Monteiro, J. P.; Melo, T.; Skjermo, J.; Forbord, S.; Broch, O. J.; Domingues, P.; Calado, R.; Domingues, M. R. Effect of Harvesting Month and Proximity to Fish Farm Sea Cages on the Lipid Profile of Cultivated Saccharina Latissima. Algal. Res. 2021, 54, 102201. DOI: 10.1016/j.algal.2021.102201.
- Moreda-Piñeiro, J.; Moreda-Piñeiro, A.; Romarís-Hortas, V.; Domínguez-González, R.; Alonso-Rodríguez, E.; López-Mahía, P.; Muniategui-Lorenzo, S.; Prada-Rodríguez, D.; Bermejo-Barrera, P. Trace Metals in Marine Foodstuff: Bioavailability Estimation and Effect of Major Food Constituents. Food Chem. 2012, 134(1), 339–345. DOI: 10.1016/j.foodchem.2012.02.165.
- Moreda-Piñeiro, J.; Moreda-Piñeiro, A.; Romarís-Hortas, V.; Dominguez-Gonzalez, R.; Alonso-Rodríguez, E.; Lopez-Mahia, P.; Muniategui-Lorenzo, S.; Prada-Rodriguez, D.; Bermejo-Barrera, P. ICP-MS for the Determination of Selenium Bioavailability from Seafood and Effect of Major Food Constituents. Microchem. J. 2013, 108, 174–179. DOI: 10.1016/j.microc.2012.10.019.
- Moreira, A. S.; da Costa, E.; Melo, T.; Lopes, D.; Pais, A. C.; Santos, S. A.; Pitarma, B.; Mendes, M.; Abreu, M. H.; Collén, P. N. Polar Lipids of Commercial Ulva Spp. of Different Origins: Profiling and Relevance for Seaweed Valorization. Foods. 2021, 10(5), 914. DOI: 10.3390/foods10050914.
- Moutinho, S.; Linares, F.; Rodríguez, J.; Sousa, V.; Valente, L. Inclusion of 10% Seaweed Meal in Diets for Juvenile and On-Growing Life Stages of Senegalese Sole (Solea Senegalensis). J. Appl. Phycol. 2018, 30, 3589–3601. DOI: 10.1007/s10811-018-1482-6.
- Mwalugha, H. M.; Wakibia, J. G.; Kenji, G. M.; Mwasaru, M. A. Chemical Composition of Common Seaweeds from the Kenya Coast. J. Food Res. 2015, 4(6), 28. DOI: 10.5539/JFR.V4N6P28.
- Negreanu-Pîrjol, B.; Negreanu-Pîrjol, T.; Paraschiv, G.; Bratu, M.; Sîrbu, R.; Roncea, F.; Meghea, A. Physical-Chemical Characterization of Some Green and Red Macrophyte Algae from the Romanian Black Sea Littoral. Sci. Study Res. Chem. Chem. Eng, Biotechnol. Food Ind. 2011, 12(2), 173.
- Neto, R. T.; Marçal, C.; Queirós, A. S.; Abreu, H.; Silva, A. M. S.; Cardoso, S. M. Screening of Ulva Rigida, Gracilaria sp., Fucus Vesiculosus and Saccharina Latissima as Functional Ingredients. Int. J. Mol. Sci. 2018, 19(10). DOI: 10.3390/ijms19102987.
- Nielsen, M. M.; Manns, D.; D’Este, M.; Krause-Jensen, D.; Rasmussen, M. B.; Larsen, M. M.; Alvarado-Morales, M.; Angelidaki, I.; Bruhn, A. Variation in Biochemical Composition of Saccharina Latissima and Laminaria Digitata Along an Estuarine Salinity Gradient in Inner Danish Waters. Algal. Res. 2016, 13, 235–245. DOI: 10.1016/j.algal.2015.12.003.
- Nielsen, C. W.; Holdt, S. L.; Sloth, J. J.; Marinho, G. S.; Sæther, M.; Funderud, J.; Rustad, T. Reducing the High Iodine Content of Saccharina Latissima and Improving the Profile of Other Valuable Compounds by Water Blanching. Foods. 2020, 9(5), 569. DOI: 10.3390/foods9050569.
- Nitschke, U.; Stengel, D. B. Quantification of Iodine Loss in Edible Irish Seaweeds During Processing. J. Appl. Phycol. 2016, 28, 3527–3533. DOI: 10.1007/s10811-016-0868-6.
- Nunes, N.; Ferraz, S.; Valente, S.; Barreto, M. C.; Pinheiro de Carvalho, M. Biochemical Composition, Nutritional Value, and Antioxidant Properties of Seven Seaweed Species from the Madeira Archipelago. J. Appl. Phycol. 2017, 29, 2427–2437. DOI: 10.1007/s10811-017-1074-x.
- Olsson, J.; Toth, G. B.; Albers, E. Biochemical Composition of Red, Green and Brown Seaweeds on the Swedish West Coast. J. Appl. Phycol. 2020, 32(5), 3305–3317. DOI: 10.1007/s10811-020-02145-w.
- Oucif, H.; Benaissa, M.; Ali Mehidi, S.; Prego, R.; Aubourg, S. P.; Abi-Ayad, S.-M. E.-A. Chemical Composition and Nutritional Value of Different Seaweeds from the West Algerian Coast. J. Aq Food Prod. Technol. 2020, 29(1), 90–104. DOI: 10.1080/10498850.2019.1695305.
- Paiva, L.; Lima, E.; Neto, A. I.; Marcone, M.; Baptista, J. Health-Promoting Ingredients from Four Selected Azorean Macroalgae. Food. Res. Int. 2016, 89, 432–438. DOI: 10.1016/j.foodres.2016.08.007.
- Paiva, L.; Lima, E.; Neto, A. I.; Marcone, M.; Baptista, J. Nutritional and Functional Bioactivity Value of Selected Azorean Macroalgae: Ulva Compressa, Ulva Rigida, Gelidium Microdon, and Pterocladiella Capillacea. J. Food Sci. 2017, 82(7), 1757–1764. DOI: 10.1111/1750-3841.13778.
- Paul, J.; Sheeba, M. Atomic Absorption Spectroscopic Determination and Comparison of Some Mineral Elements in Ulva Rigida C. AG. from Hare Island, Thoothukudi Tamil Nadu, India. World J. Pharm. Res. 2014, 3(4), 785–795.
- Peasura, N.; Laohakunjit, N.; Kerdchoechuen, O.; Wanlapa, S. Characteristics and Antioxidant of Ulva Intestinalis Sulphated Polysaccharides Extracted with Different Solvents. Int J. Biol. Macromol. 2015, 81, 912–919. DOI: 10.1016/j.ijbiomac.2015.09.030.
- Pétursdóttir, Á. H.; Gunnlaugsdóttir, H. Selective and Fast Screening Method for Inorganic Arsenic in Seaweed Using Hydride Generation Inductively Coupled Plasma Mass Spectrometry (HG-ICPMS). Microchem. J. 2019, 144, 45–50. DOI: 10.1016/j.microc.2018.08.055.
- Pirian, K.; Piri, K.; Sohrabipour, J.; Jahromi, S. T.; Blomster, J. Nutritional and Phytochemical Evaluation of the Common Green Algae, Ulva Spp. (Ulvophyceae), from the Persian Gulf. Fund. Appl. Limnol. 2016, 188(4), 315–327. DOI: 10.1127/fal/2016/0947.
- Queirós, A. S.; Circuncisão, A. R.; Pereira, E.; Válega, M.; Abreu, M. H.; Silva, A. M.; Cardoso, S. M. Valuable Nutrients from Ulva Rigida: Modulation by Seasonal and Cultivation Factors. Appl. Sci. 2021, 11(13), 6137. DOI: 10.3390/app11136137.
- Raab, A.; Newcombe, C.; Pitton, D.; Ebel, R.; Feldmann, J. Comprehensive Analysis of Lipophilic Arsenic Species in a Brown Alga (Saccharina Latissima). Anal. Chem. 2013, 85(5), 2817–2824. DOI: 10.1021/ac303340t.
- Ramin, M.; Franco, M.; Roleda, M. Y.; Aasen, I. M.; Hetta, M.; Steinshamn, H. In vitro Evaluation of Utilisable Crude Protein and Methane Production for a Diet in Which Grass Silage Was Replaced by Different Levels and Fractions of Extracted Seaweed Proteins. Anim. Feed Sci. Technol. 2019, 255, 114225. DOI: 10.1016/j.anifeedsci.2019.114225.
- Rasyid, A. Evaluation of Nutritional Composition of the Dried Seaweed Ulva Lactuca from Pameungpeuk Waters, Indonesia. Trop. Life Sci. Res. 2017, 28(2), 119. DOI: 10.21315/tlsr2017.28.2.9.
- Reka, P.; Banu, T. A.; Seethalakshmi, M. Elemental Composition of Selected Edible Seaweeds Using SEM-Energy Dispersive Spectroscopic Analysis. Int. Food Res. J. 2017, 24(2), 600.
- Ripol, A.; Cardoso, C.; Afonso, C.; Varela, J.; Quental-Ferreira, H.; Pousão-Ferreira, P.; Bandarra, N. M. Composition, Anti-Inflammatory Activity, and Bioaccessibility of Green Seaweeds from Fish Pond Aquaculture. Nat. Prod. Comm. 2018, 13(5), 1934578X1801300. DOI: 10.1177/1934578X1801300521.
- Rohani-Ghadikolaei, K.; Abdulalian, E.; Ng, W.-K. Evaluation of the Proximate, Fatty Acid and Mineral Composition of Representative Green, Brown and Red Seaweeds from the Persian Gulf of Iran as Potential Food and Feed Resources. J. Food Sci. Techol. 2012, 49(6), 774–780. DOI: 10.1007/s13197-010-0220-0.
- Roleda, M. Y.; Skjermo, J.; Marfaing, H.; Jónsdóttir, R.; Rebours, C.; Gietl, A.; Stengel, D. B.; Nitschke, U. Iodine Content in Bulk Biomass of Wild-Harvested and Cultivated Edible Seaweeds: Inherent Variations Determine Species-Specific Daily Allowable Consumption. Food Chem. 2018, 254, 333–339. DOI: 10.1016/j.foodchem.2018.02.024.
- Roleda, M. Y.; Marfaing, H.; Desnica, N.; Jónsdóttir, R.; Skjermo, J.; Rebours, C.; Nitschke, U. Variations in Polyphenol and Heavy Metal Contents of Wild-Harvested and Cultivated Seaweed Bulk Biomass: Health Risk Assessment and Implication for Food Applications. Food Cont. 2019, 95, 121–134. DOI: 10.1016/j.foodcont.2018.07.031.
- Samarasinghe, M. B.; Sehested, J.; Weisbjerg, M. R.; Vestergaard, M.; Hernández-Castellano, L. E. Milk Supplemented with Dried Seaweed Affects the Systemic Innate Immune Response in Preweaning Dairy Calves. J. Dairy. Sci. 2021, 104(3), 3575–3584. DOI: 10.3168/jds.2020-19528.
- Samarasinghe, M. B.; van der Heide, M. E.; Weisbjerg, M. R.; Sehested, J.; Sloth, J. J.; Bruhn, A.; Vestergaard, M.; Nørgaard, J. V.; Hernández-Castellano, L. E. A Descriptive Chemical Analysis of Seaweeds, Ulva sp., Saccharina Latissima and Ascophyllum Nodosum Harvested from Danish and Icelandic Waters. Anim. Feed Sci. Technol. 2021, 278. DOI: 10.1016/j.anifeedsci.2021.115005.
- Sappati, P. K.; Nayak, B.; VanWalsum, G. P.; Mulrey, O. T. Combined Effects of Seasonal Variation and Drying Methods on the Physicochemical Properties and Antioxidant Activity of Sugar Kelp (Saccharina Latissima). J. Appl. Phycol. 2019, 31(2), 1311–1332. DOI: 10.1007/s10811-018-1596-x.
- Schiener, P.; Black, K. D.; Stanley, M. S.; Green, D. H. The Seasonal Variation in the Chemical Composition of the Kelp Species Laminaria Digitata, Laminaria Hyperborea, Saccharina Latissima and Alaria Esculenta. J. Appl. Phycol. 2015, 27(1), 363–373. DOI: 10.1007/s10811-014-0327-1.
- Schiener, P.; Zhao, S.; Theodoridou, K.; Carey, M.; Mooney-McAuley, K.; Greenwell, C. The Nutritional Aspects of Biorefined Saccharina Latissima, Ascophyllum Nodosum and Palmaria Palmata. Biomass Convers. Biorefin. 2017, 7(2), 221–235. DOI: 10.1007/s13399-016-0227-5.
- Sharma, S.; Neves, L.; Funderud, J.; Mydland, L. T.; Øverland, M.; Horn, S. J. Seasonal and Depth Variations in the Chemical Composition of Cultivated Saccharina Latissima. Algal. Res. 2018, 32, 107–112. DOI: 10.1016/j.algal.2018.03.012.
- Shuuluka, D.; Bolton, J. J.; Anderson, R. J. Protein Content, Amino Acid Composition and Nitrogen-To-Protein Conversion Factors of Ulva Rigida and Ulva Capensis from Natural Populations and Ulva Lactuca from an Aquaculture System, in South Africa. J. Appl. Phycol. 2013, 25(2), 677–685. DOI: 10.1007/s10811-016-0984-3.
- Stévant, P.; Marfaing, H.; Rustad, T.; Sandbakken, I.; Fleurence, J.; Chapman, A. Nutritional Value of the Kelps Alaria Esculenta and Saccharina Latissima and Effects of Short-Term Storage on Biomass Quality. J. Appl. Phycol. 2017, 29(5), 2417–2426. DOI: 10.1007/s10811-017-1126-2.
- Stévant, P.; Indergård, E.; Ólafsdóttir, A.; Marfaing, H.; Larssen, W. E.; Fleurence, J.; Roleda, M. Y.; Rustad, T.; Slizyte, R.; Nordtvedt, T. S. Effects of Drying on the Nutrient Content and Physico-Chemical and Sensory Characteristics of the Edible Kelp Saccharina Latissima. J. Appl. Phycol. 2018, 30(4), 2587–2599. DOI: 10.1007/s10811-018-1451-0.
- Stévant, P.; Marfaing, H.; Duinker, A.; Fleurence, J.; Rustad, T.; Sandbakken, I.; Chapman, A. Biomass Soaking Treatments to Reduce Potentially Undesirable Compounds in the Edible Seaweeds Sugar Kelp (Saccharina Latissima) and Winged Kelp (Alaria Esculenta) and Health Risk Estimation for Human Consumption. J. Appl. Phycol. 2018, 30(3), 2047–2060. DOI: 10.1007/s10811-017-1343-8.
- Susanto, E.; Fahmi, A. S.; Hosokawa, M.; Miyashita, K. Variation in Lipid Components from 15 Species of Tropical and Temperate Seaweeds. Mar. Drugs. 2019, 17(11), 630. DOI: 10.3390/md17110630.
- Tabarsa, M.; Rezaei, M.; Ramezanpour, Z.; Waaland, J. R. Chemical Compositions of the Marine Algae Gracilaria Salicornia (Rhodophyta) and Ulva Lactuca (Chlorophyta) as a Potential Food Source. J. Sci. Food Agr. 2012, 92(12), 2500–2506. DOI: 10.1002/jsfa.5659.
- Taboada, C.; Millán, R.; Míguez, I. Evaluation of the Marine Alga Ulva Rigida as a Food Supplement: Effect of Intake on Intestinal, Hepatic, and Renal Enzyme Activities in Rats. J. Med. Food. 2011, 14(1–2), 161–166. DOI: 10.1089/jmf.2009.0291.
- Taylor, V. F.; Jackson, B. P. Concentrations and Speciation of Arsenic in New England Seaweed Species Harvested for Food and Agriculture. Chemosphere. 2016, 163, 6–13. DOI: 10.1016/j.chemosphere.2016.08.004.
- Thodhal Yoganandham, S.; Raguraman, V.; Muniswamy, G.; Sathyamoorthy, G.; Rajan Renuka, R.; Chidambaram, J.; Rajendran, T.; Chandrasekaran, K.; Santha Ravindranath, R. R. Mineral and Trace Metal Concentrations in Seaweeds by Microwave-Assisted Digestion Method Followed by Quadrupole Inductively Coupled Plasma Mass Spectrometry. Biol. Trace Elem. Res. 2019, 187, 579–585. DOI: 10.1007/s12011-018-1397-8.
- Tibbetts, S. M.; Milley, J. E.; Lall, S. P. Nutritional Quality of Some Wild and Cultivated Seaweeds: Nutrient Composition, Total Phenolic Content and in vitro Digestibility. J. Appl. Phycol. 2016, 28(6), 3575–3585. DOI: 10.1007/s10811-016-0863-y.
- Tolpeznikaite, E.; Bartkevics, V.; Ruzauskas, M.; Pilkaityte, R.; Viskelis, P.; Urbonaviciene, D.; Zavistanaviciute, P.; Zokaityte, E.; Ruibys, R.; Bartkiene, E. Characterization of Macro- and Microalgae Extracts Bioactive Compounds and Micro- and Macroelements Transition from Algae to Extract. Foods. 2021, 10(9). DOI: 10.3390/foods10092226.
- Trigo, J. P.; Engström, N.; Steinhagen, S.; Juul, L.; Harrysson, H.; Toth, G. B.; Pavia, H.; Scheers, N.; Undeland, I. In vitro Digestibility and Caco-2 Cell Bioavailability of Sea Lettuce (Ulva Fenestrata) Proteins Extracted Using Ph-Shift Processing. Food Chem. 2021, 356, 129683. DOI: 10.1016/j.foodchem.2021.129683.
- Turan, G.; Tekogul, H. The Turkish Mezzes Formulated with Protein-Rich Green Sea Vegetable (Chlorophyta), Ulva Rigida, Cultured in Onshore Tank System. J. Aqua. Food Prod. Technol. 2014, 23(5), 447–452. DOI: 10.1080/10498850.2012.723307.
- Turan, F.; Ozgun, S.; Sayın, S.; Ozyılmaz, G. Biochemical Composition of Some Red and Green Seaweeds from Iskenderun Bay, the Northeastern Mediterranean Coast of Turkey. J. Black Sea/Mediterranean Environ. 2015, 21, 239–249.
- Uribe, E.; Vega-Gálvez, A.; García, V.; Pastén, A.; López, J.; Goñi, G. Effect of Different Drying Methods on Phytochemical Content and Amino Acid and Fatty Acid Profiles of the Green Seaweed, Ulva Spp. J. Appl. Phycol. 2019, 31, 1967–1979. DOI: 10.1007/s10811-018-1686-9.
- Verma, P.; Kumar, M.; Mishra, G.; Sahoo, D. Multivariate Analysis of Fatty Acid and Biochemical Constitutes of Seaweeds to Characterize Their Potential as Bioresource for Biofuel and Fine Chemicals. Bioresources Technol. 2017, 226, 132–144. DOI: 10.1016/j.biortech.2016.11.044.
- Viera, M.; De Vicose, G. C.; Gómez-Pinchetti, J.; Bilbao, A.; Fernandez-Palacios, H.; Izquierdo, M. Comparative Performances of Juvenile Abalone (Haliotis Tuberculata Coccinea Reeve) Fed Enriched Vs Non-Enriched Macroalgae: Effect on Growth and Body Composition. Aquaculture. 2011, 319(3–4), 423–429. DOI: 10.1016/j.aquaculture.2011.07.024.
- Viera, M.; de Viçose, G. C.; Robaina, L.; Izquierdo, M. First Development of Various Vegetable-Based Diets and Their Suitability for Abalone Haliotis Tuberculata Coccinea Reeve. Aquaculture. 2015, 448, 350–358. DOI: 10.1016/j.aquaculture.2015.05.031.
- Vilg, J. V.; Undeland, I. pH-Driven Solubilization and Isoelectric Precipitation of Proteins from the Brown Seaweed Saccharina Latissima-Effects of Osmotic Shock, Water Volume and Temperature. J. Appl. Phycol. 2017, 29(1), 585–593. DOI: 10.1007/s10811-016-0957-6.
- Wan, A. H. L.; Wilkes, R. J.; Heesch, S.; Bermejo, R.; Johnson, M. P.; Morrison, L. Assessment and Characterisation of Ireland’s Green Tides (Ulva Species). PLoS One. 2017, 12(1), e0169049. DOI: 10.1371/journal.pone.0169049.
- Yildiz, G.; Dere, E.; Dere, S. Comparison of the Antioxidative Components of Some Marine Macroalgae from Turkey. Pak. J. Bot. 2014, 46(2), 753–757.
- Zeroual, S.; El Bakkal, S. E.; Mansori, M.; Lhernould, S.; Faugeron-Girard, C.; El Kaoua, M.; Zehhar, N. Cell Wall Thickening in Two Ulva Species in Response to Heavy Metal Marine Pollution. Reg. Stud Mar. Sci. 2020, 35, 101125. DOI: 10.1016/j.rsma.2020.101125.
- Chirapart, A.; Praiboon, J.; Puangsombat, P.; Pattanapon, C.; Nunraksa, N. Chemical Composition and Ethanol Production Potential of Thai Seaweed Species. J. Appl. Phycol. 2014, 26, 979–986. DOI: 10.1007/s10811-013-0235-9.
- Rutzke, C. J.; Glahn, R. P.; Rutzke, M. A.; Welch, R. M.; Langhans, R. W.; Albright, L. D.; Combs, G. F., Jr; Wheeler, R. M. Bioavailability of Iron from Spinach Using an in Vitro/Human Caco-2 Cell Bioassay Model. Habitation (Elmsford). 2004, 10(1), 7–14. DOI: 10.3727/154296604774808900.
- Marques de Brito, B.; Campos, V. D. M.; Neves, F. J.; Ramos, L. R.; Tomita, L. Y. Vitamin B12 Sources in Non-Animal Foods: A Systematic Review. Crit. Rev. Food Sci. Nutr. 2022, 1–15. DOI: 10.1080/10408398.2022.2053057.
- Duinker, A.; Kleppe, M.; Fjære, E.; Biancarosa, I.; Hilde, E. H.; Dahl, L.; Lunestad, B. T. Knowledge Update on Macroalgae Food and Feed Safety - Based on Data Generated in the Period 2014-2019 by the Institute of Marine Research, Norway; Bergen, Norway: National Institutes of Nutrition and Seafood Research (NIFES), 2020.
- Hornborg, S.; Ziegler, F.; Thomas, J. B.; Axelsson, A.; Wocken, Y.; Sanders, C.; Jacobsen, M.; Hallström, E.; Bianchi, M.; Bryngelsson, S. Environmental and Nutritional Perspectives of Algae with Emphasis on Swedish Conditions; In print. RISE Research Institutes of Sweden: Gothenburg, Sweden, 2023.
- Hogstad, S.; Cederberg, D.; Eriksen, H.; Kollander, B.; Ólafsson, G.; Mikkelsen, B. A Nordic Approach to Food Safety Risk Management of Seaweed for Use as Food. Current Status and Basis for Future Work; Nordic Council of Ministers, 2022; 564.
- Trigo, J. P.; Stedt, K.; Schmidt, A. E. M.; Kollander, B.; Edlund, U.; Nylund, G.; Pavia, H.; Abdollahi, M.; Undeland, I. Mild Blanching Prior to pH-Shift Processing of Saccharina Latissima Retains Protein Extraction Yields and Amino Acid Levels of Extracts While Minimizing Iodine Content. Food Chem. 2023, 404, 8. DOI: 10.1016/j.foodchem.2022.134576.
- Stedt, K.; Steinhagen, S.; Trigo, J. P.; Kollander, B.; Undeland, I.; Toth, G. B.; Wendin, K.; Pavia, H. Post-Harvest Cultivation with Seafood Process Waters Improves Protein Levels of Ulva Fenestrata While Retaining Important Food Sensory Attributes. Front. Mar. Sci. 2022, 9. DOI: 10.3389/fmars.2022.991359.
- Bikker, P.; van Krimpen, M. M.; van Wikselaar, P.; Houweling-Tan, B.; Scaccia, N.; van Hal, J. W.; Huijgen, W. J.; Cone, J. W.; López-Contreras, A. M. Biorefinery of the Green Seaweed Ulva Lactuca to Produce Animal Feed, Chemicals and Biofuels. J. Appl. Phycol. 2016, 28(6), 3511–3525. DOI: 10.1007/s10811-016-0842-3.
- Mæhre, H. K.; Jensen, I.-J.; Eilertsen, K.-E. Enzymatic Pre-Treatment Increases the Protein Bioaccessibility and Extractability in Dulse (Palmaria Palmata). Mar. Drugs. 2016, 14(11), 196. DOI: 10.3390/md14110196.
- Blikra, M. J.; Henjum, S.; Aakre, I. Iodine from Brown Algae in Human Nutrition, with an Emphasis on Bioaccessibility, Bioavailability, Chemistry, and Effects of Processing: A Systematic Review. Compr. Rev. Food Sci. Food Sa. 2022, 21(2), 1517–1536. DOI: 10.1111/1541-4337.12918.
- Akalya, K.; Kumar, S. D.; Manigandan, G.; Santhanam, P.; Perumal, P.; Krishnaveni, N.; Arthikha, R.; Begum, A.; Dhanalakshmi, B.; Kim, M.-K. The Influence of the Macroalgae Liquid Extracts on the Pigments and Fatty Acids Profile of the Marine Microalga, Picochlorum Maculatum (PSDK01). Thalassas. 2022, 38(1), 553–564. DOI: 10.1007/s41208-021-00338-9.
- Sabeena Farvin, K. H.; Jacobsen, C. Phenolic Compounds and Antioxidant Activities of Selected Species of Seaweeds from Danish Coast. Food Chem. 2013, 138(2–3), 1670–1681. DOI: 10.1016/j.foodchem.2012.10.078.
- Ferreira, M.; Teixeira, C.; Abreu, H.; Silva, J.; Costas, B.; Kiron, V.; Valente, L. M. P. Nutritional Value, Antimicrobial and Antioxidant Activities of Micro- and Macroalgae, Single or Blended, Unravel Their Potential Use for Aquafeeds. J. Appl. Phycol. 2021, 33(6), 3507–3518. DOI: 10.1007/s10811-021-02549-2.
- Jard, G.; Jackowiak, D.; Carrère, H.; Delgenes, J. P.; Torrijos, M.; Steyer, J. P.; Dumas, C. Batch and Semi-Continuous Anaerobic Digestion of Palmaria Palmata: Comparison with Saccharina Latissima and Inhibition Studies. Chem. Eng. J. 2012, 209, 513–519. DOI: 10.1016/j.cej.2012.08.010.
- Stévant, P.; Rebours, C.; Chapman, A. Seaweed Aquaculture in Norway: Recent Industrial Developments and Future Perspectives. Aquac. Inter. 2017, 25(4), 1373–1390. DOI: 10.1007/s10499-017-0120-7.
- Vilg, J. V.; Nylund, G. M.; Werner, T.; Qvirist, L.; Mayers, J. J.; Pavia, H.; Undeland, I.; Albers, E. Seasonal and Spatial Variation in Biochemical Composition of Saccharina Latissima During a Potential Harvesting Season for Western Sweden. Botanica Marina. 2015, 58(6). DOI: 10.1515/bot-2015-0034.