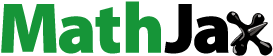
ABSTRACT
The food industry is faced with a significant challenge in maintaining the viability and stability of probiotics during processing and in model food. Encapsulation technology offers a promising solution to this issue. This study was aimed to investigate the effect of Sodium Alginate (SA) and arabinoxylan (AX) composite encapsulation on the viability & stability of Lactobacillus rhamnosus GG. The AX was extracted from maize and characterized, and then the SA-AX composite was used to encapsulate the probiotics. The resulting microbeads were analyzed for their morphological, molecular, and physicochemical properties. The MAX-SA microbeads demonstrated the highest efficiency at 97.9 ± 0.6%, followed by MAX at 95 ± 1.5% and SA at 92 ± 1.4%. The FTIR spectra revealed specific functional groups in the samples. The MAX-SA and MSA matrices had a dispersed structure, while the MAX matrix had a smooth microstructure. The microcapsules had an average size ranging from 718 ± 2 mm to 734 ± 2 mm. The viability of the encapsulated probiotics was assessed under storage conditions, simulated gastrointestinal conditions, and model food. Encapsulated probiotics showed higher viability than free probiotics in simulated gastrointestinal conditions, and pineapple juice fortified with encapsulated probiotics showed a higher probiotic count. Overall, the study found that MAX-SA was the most effective in maintaining probiotic viability under stressed conditions.
Introduction
Encapsulation of probiotics is a technique to create a protective micro-environment for the bacteria and to preserve their metabolic functions. The encapsulating material should offer a suitable micro-environment for the bacteria to survive during processing and storage and to be delivered to specific sites in the gastrointestinal tract. The research on probiotics encapsulation methods has grown in recent years because they can produce capsules with relatively high encapsulation efficiency without using heat during the processing.[Citation1,Citation2]
Alginate basil seed mucilages encapsulated with different concentration of prebiotics have greater ability to survival the probiotics. The incorporation of probiotics and prebiotics into one’s diet is of great significance for both the food and pharmaceutical sectors, as they offer numerous benefits to the overall health of the host. However, probiotic bacteria encounter various obstacles that could potentially impede their survival and functionality, including temperature variations, acidic and bile environments, specific ions, as well as levels of water and oxygen.[Citation3]
Probiotics are classified as functional foods that offer numerous health advantages, including the maintenance of a healthy gut bacteria balance, reduction of intestinal inflammation, and enhancement of the immune system. Consumers are increasingly willing to pay a premium for these benefits, leading to a surge in global demand for probiotics foods. The current value of the global probiotics market stands at USD 61.1 billion in 2021, with an anticipated annual growth rate of 8.3%, reaching USD 91.1 billion by 2026.[Citation4] This growth rate is driven by the health benefits of probiotic foods and the innovative technologies used to produce them. Consuming dietary fiber can yield numerous favorable health outcomes, including a reduced risk of developing cardiovascular diseases, diabetes, obesity, colorectal cancer, and other related conditions.[Citation5] Furthermore, different studies insisted some possible gut microbiota modulation factors such as probiotics, prebiotics and synbiotics to improve gut microbiota dysfunction under different diseases. In 2020, the International Scientific Association for Probiotics and Prebiotics (ISAPP) defined synbiotics as “a mixture comprising live microorganisms and substrates selectively utilized by host microorganisms that confer a health benefit on the host.” They also categorized synbiotics into two types: complementary synbiotics and synergistic synbiotics (s-syn).Synbiotics benefit the host by producing metabolites, regulating the immune system, and influencing neurotransmitter production.Therefore, various studies are identified a new synergistic synbiotic candidate (SSC) treatment with the potential to reduce gut inflammation and regulate brain function through its anti-inflammatory and antioxidant properties.[Citation6] Stevia and inulin have prebiotic potential which can be used on fermentation of milk.[Citation7]
Dietary fiber can help health mainly by making short-chain fatty acids (SCFA), which are good for the body. SCFA are made when gut bacteria break down dietary fiber.[Citation8] Arabinoxylans (AX) are a variety of grain-derived dietary fiber. They are found in many grains, such as rye, wheat, barley, oats, rice, sorghum, maize, millet, and others. They are also in some other plants, like psyllium flax seed, pangola grass, bamboo shoots, and ryegrass. Rye has the most arabinoxylans of all the grains, then wheat, barley, oats, rice, and sorghum. Arabinoxylans are in the cell walls of different parts of the grains, like the starchy endosperm, aleurone, bran tissues, and husks. But different plants have different amounts and kinds of arabinoxylans (Nino-Medina et al.).[Citation9] Its compounds have significant nutritional value and versatile applications, such as prebiotics or supplementary ingredients, in the food manufacturing industry.[Citation10] AX has a positive impact on human health through its anti-inflammatory and anti-cancer properties, ability to prevent constipation, lower blood cholesterol levels, regulate blood glucose levels, and enhance the absorption of minerals. When fermented in the large intestine, AX produces short-chain fatty acids (SCFA) with prebiotics properties, promoting the growth of lactic acid bacteria. This makes AX suitable for the development of targeted delivery systems for the colon.[Citation11]
AX structure can change depending on where this polysaccharide comes from and how it is extracted. So, the parts, the size, the amount of substitution, and other structural aspects, can vary among the AX.[Citation5] The polysaccharide AX exhibits the ability to withstand variations in temperature, pH, and ionic strength when formed into gels, making it highly advantageous for industrial applications. Another study has shown that AX gels can trap probiotics. But that study used AX from wheat flour that can be extracted with water as a simpler example of this polysaccharide than AX from cereal brands that cannot be extracted with water. In this case, maize bran has more and cheaper this polysaccharide, especially when AX is taken from food industry leftovers, which are more popular and would give new benefits for using this polysaccharide in the industry later.[Citation12,Citation13]
In a recent study conducted by Carvajal-Millan et al.[Citation14] it was demonstrated that AX gels acted as a prebiotic, benefiting rats with obesity. The gels promoted the growth of beneficial bacteria such as Bifidobacterium and contributed to achieving a balanced Bacteroidetes/Firmicutes ratio. Given the potential of AX to create protective systems for probiotic bacteria, the current study aims to investigate the efficiency of these shielded bacteria in breaking down AX. Yingying et al. (2023) studies the co-encapsulation of Lactobacillus plantarum by using AX and beta glucan polysaccharides, they concluded that co-encapsulation of probiotic with non-starch polysaccharides increase the storage and viability of Lactobacillus plantarum.[Citation15] The main objective of this research was to assess how encapsulation through extrusion, utilizing various wall materials such as sodium alginate (SA), Arabinoxylan (AX), and Arabinoxylan-sodium alginate (AX-SA), impact the survival and endurance of L. rhamnosus when subjected to simulated gastrointestinal conditions.
Materials and methods
Procurement of raw material
The food analysis lab, Department of Food Sciences, Government College University of Faisalabad, graciously provided the arabinoxylan. The probiotic culture (L. rhamnosus) was generously provided by NIFSAT, University of Agriculture Faisalabad, Pakistan. The study was carried out at the Food Safety and Biotechnology lab, Government College University Faisalabad, Pakistan. All chemicals and reagents utilized in the experiment were of reagent grade.
Activation of probiotic
The freeze-dried form of pure probiotic bacteria (L. rhamnosus) culture was obtained from NIFSAT, the National Institute of Food Science & Technology at the University of Agriculture Faisalabad, Pakistan. To activate the probiotic, the culture was inoculated in MRS (Man Rogosa Sharpe) broth and incubated at 37°C for 24 hours. The centrifuge (75005286 EA) used in the study was provided by Thermo Fisher Scientific Inc. in the USA, and the probiotic cells were centrifuged prior to being washed with a sterile saline solution. The collected probiotic cells were then utilized in the encapsulation process, with their concentration adjusted to 109 cfu/mL.
Probiotic encapsulation
Slight modifications were made to Yeung’s[Citation16] method to encapsulate L.rhamnosus using sodium alginate and Arabinoxylan extract. Solutions of Arabinoxylan (2.0% w/v) and sodium alginate (2.0% w/v) were prepared and subjected to sterilization at 121°C (15 psi) for 15 minutes. The resulting solution was autoclaved at 121°C for 15 minutes. After cooling, the prepared solutions were combined with the culture (1010log cfu/ml) suspended in 0.1% sterile peptone at a ratio of 9:1 (v/v). To facilitate the extrusion process, the mixtures were homogenized using an ultra-turret at 1,960 g for 2 minutes. Subsequently, the suspensions were gradually injected through a syringe into a gently stirred solution of 0.2 M CaCl2. The beads were made and then agitated in CaCl2 for 30 min at 300 rpm to harden them. They were then placed on sterile Petri dishes and maintained at 4°C after being filtered with a sterile filter and twice cleaned with sterile distilled water.
Encapsulation efficiency
To measure the encapsulation efficiency of probiotic bacteria, we followed the method of Cehran et al.[Citation17] We fragmented the probiotic micro-beads in a buffer solution using a stomacher (Seward, UK) for 15 min until all the beads released the probiotics inside them. Subsequently, a quantity of one gram of encapsulated probiotics was taken from each sample and combined with nine milliliters of phosphate buffer solution. The pour plate technique was then employed to determine the cell count within the beads. The plates were incubated under anaerobic conditions at 37°C. The encapsulation efficiency was calculated using the provided formula, expressed as colony-forming units per bead (cfu/bead).
In contrast, N: represents the number of viable cells released from the encapsulated beads. N0: signifies the count of unencapsulated cells present in the bio-polymer mixture before the encapsulation process.
Particle size
The size or diameter of the encapsulated cells was assessed by employing a stage micrometer. To determine their size and shape, the prepared beads were observed under a light microscope. The average sizes of the beads were recorded both before and after storage.
Zeta potential
The zeta potential of the prepared beads was evaluated using a method similar to that described by Ji et al.[Citation18] with slight modification. Purified water was employed to disperse the beads. The beads were dispersed before being placed into a zeta potentiometer sample cell (Zetasizer Nano ZSP, Malvern Analytical, Malvern, United Kingdom). The sample solution was given five minutes to naturally settle. A zeta potentiometer was then used to hold the sample solution in order to take measurements.
Moisture
The moisture content of probiotic bacteria that were encapsulated was measured using a moisture analyzer (Model XY-105 MW, Shanghai, China).
Scanning electron microscopy
To obtain a comprehensive understanding of the structural properties of micro-encapsulated beads SA, AX, and AX-SA, scanning electron microscopy (SEM) was employed. The samples were placed on stubs at a 5-kV accelerating voltage and examined using a scanning electron microscope (cube series company, Emcraft). The resulting micro-graphs were then analyzed to gain insights into their morphological characteristics.
Fourier transform-infrared (FT-IR) spectroscopy
Spectrometer (Shimadzu’s IR Prestige-21) was used to record the FT-IR spectra of EOC, MCD, and EOC/MCD-ICs in the wave number range of 4000–400 cm‒1. The discs were prepared using the KBr pellet method. Exactly 100 mg of spectroscopic grade KBr and 3 mg of dried samples were blended together and the mixture was then pressed into 1 mm pellets.
In-vitro study
The viability of both free and encapsulated probiotics under simulated conditions was assessed. The viability or survival of both free and encapsulated probiotics was assessed, utilizing a modified method based on Araújo Etchepare et al.[Citation19] procedure. The simulated gastric and intestinal solution was promptly prepared. To generate the simulated gastric juice, 0.03 M phosphate buffer and 10 mg/ml pepsin were added to a test tube filled with warm water. The pH was adjusted to 2 using 1 M HCL. The prepared solution was then combined with the free and encapsulated probiotics, followed by incubation at 37°C for a specific duration.
The protocol for preparing the simulated intestinal juice was executed. In summary, sterilized water was combined with 0.03 M phosphate buffer, 10 mg/ml trypsin, and 0.3% bile salt. The pH was adjusted to 7.5 using 1 M NaOH. The free and encapsulated probiotics were introduced into the prepared solution, which was subsequently incubated at 37°C. The survival of both encapsulated and free-suspending cells were recorded at specific time intervals (0, 30, 60, 90, and 120 minutes).
Storage study
For a duration of four weeks, the solution was retrieved from refrigeration on a weekly basis. It was transferred to clean glass vials containing 1 mL of peptone water and maintained at 37°C with continuous stirring at 150 rpm for 10 minutes. Subsequently, the solution was stirred at a higher speed of 500 rpm until it achieved complete mixing. A small volume (100 μL) of the solution was promptly extracted from the vials, diluted, and evenly spread onto MRS or TYL agar. The plates were then incubated at 37°C for 3 days, with or without oxygen (L. rhamnosus GG). The viable cells were enumerated following 7, 14, 21, and 28 days of storage at 5°C.
Results and discussion
Encapsulation efficiency
The encapsulation efficiency of microbeads using different wall materials is presented in . The results indicate that microbeads encapsulated with arabinoxylan + sodium alginate (AX-SA, 9%) achieved significantly higher efficiency (p ≤ .05) compared to those encapsulated with Arabinoxylan (AX, 95%) or sodium alginate (SA, 92%). The enhanced efficiency of AX-SA can be attributed to its emulsifying properties and gelling capacity, while AX exhibited higher efficiency due to its ability to form a versatile matrix. It is worth noting that various factors, including the concentration of CaCl2, choice of wall material, type of microorganisms being encapsulated, particle size, and the encapsulation method employed, can influence encapsulation efficiency.
Table 1. Encapsulation Efficiency, Size, Moisture and Zeta Potential.
The encapsulation of Lactobacillus plantarum in AX-alginate composite microspheres was investigated by Wu and Zhang.[Citation20] In this study, encapsulation matrices based on AX-SA, AX, and SA were prepared to assess their capacity for encapsulating probiotic bacteria, specifically L. rhamnosus. The findings of the present study align with the results of Mendez-Encinas et al.[Citation21] who observed that the coating of Arabinoxylan led to increased encapsulation efficiency. Our findings indicate that the AX-SA, AX, and SA matrices show potential as effective encapsulating systems, as they have demonstrated efficient encapsulation of probiotic bacteria.
Particle size
The probiotic bacteria were entrapped with different wall materials Arabinoxylan (AX) and Sodium alginate (SA) have been depicted in . Findings of the research show that the particle size of Arabinoxylan-sodium alginate was observed to be the greatest 734 μm while the particle size of Arabinoxylan was 729.7 μm and the particle size of Sodium alginate was 717.7 μm. According to the results, the size of the Arabinoxylan-sodium alginate microgels wall material was clearly larger in size than that of the other microgels wall material. This can be due to the fact that the solution containing Arabinoxylan was more viscous than the sodium alginate solution.
Praepanitchai et al.[Citation22] have reported similar observations, highlighting that the choice of encapsulation materials has an impact on the size of the beads.
Moisture content (%)
The quality of micro-beads may be determined by their moisture content. An important aspect of the encapsulation sample is its moisture content. The moisture content of probiotics is an important quality criterion to consider in micro-encapsulation, as it greatly influences product stability and can also impact the viability of probiotics during storage. The effect of different reactions can be considered effective in the amount of moisture depending on the type of wall materials. Micro-bead of SA show the moisture content of 13% while AX microbeads show 11% and AX-SA show the lower moisture content as compared to sodium alginate. AX-SA is more stable.
Zeta potential
The objective of employing Zeta potential was to evaluate the surface electrical properties of both unencapsulated and encapsulated micro-beads. The average findings are presented in demonstrate the Zeta potential of micro-beads with varying encapsulation materials. Zeta potential serves as a crucial parameter in defining electro-chemical properties. The results revealed a significant influence of the encapsulation materials on the Zeta potential of the beads. Specifically, the Zeta potential values for probiotics (L. rhamnosus GG) encapsulated with SA, AX, and AX-SA were recorded as −10.7 MV, −19.3 MV, and −24.3 MV, respectively. The disparities in Zeta potential arose from differences in the number of encapsulation materials, pH level of the medium, and ionic strength. Micro-beads exhibited both negative and positive Zeta potential values. Colloidal particles with a high Zeta potential exhibit improved physical stability and increased electrostatic repulsion forces within the system. The value of Zeta potential and surface charge is influenced by various factors including pH, type of carrier, and concentration, as reported by Hadidi et al.[Citation23]
FT-IR
The FTIR spectrum of different micro beads samples are presented in . Different peak values of SA were observed in the FT-IR graph which are 2358.2 and then 2321.8, 675.3, 644.5,625.0 which indicates the different bonds (C-H), (CO2), (C-C), (N-H) and the functional group is (Alcohols, phenols, alkane, aliphatic amines). A similar value was observed in the AX FT-IR graph which is 3736.4 cm−1, 2355.4 cm−1, 2335.8 cm−1, 661.3 cm−1, 636.2 cm−1, and 613.8 cm−1 which indicates the presence of different bonds (−OH), (-NH2), (C-H), (C-C), (N-H) and functional group (Alcohols, phenols, alkane, aliphatic amines). Another AX-SA FT-IR graph shows a similar observation in which the highest peak value is 33,739.2 cm−1,3621.7 cm−1, 3366.6 cm−1,2338.6 cm−1, 1648.1 cm−1,672.5 cm−1,641.7 cm−1, and 622.2 cm−1 which indicates the presence of different bonds (−OH), (-NH2), (CO2), (C=O), (N-H), and Functional group (Alcohols, phenols, alkane, aliphatic amines). These assignments distinctly demonstrate the material’s polysaccharide structure.
Scanning electron microscopy (SEM)
Figure 2. Scanning electron micrographs of probiotic-loaded Microbeads; (a) SA (Sodium alginate), (b) AX (Arabinoxylan), (c) AX-SA (Arabinoxylan-Sodium alginate).
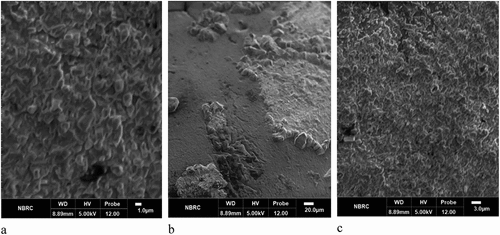
The scanning electron microscopy (SEM) images () of the microbeads revealed that the bacterial cells were fully embedded within the central matrix of the multi-layered microcapsules, exhibiting the highest level of survival following the encapsulation process. The confirmation of L. rhamnosus GG incorporation into the matrices was based on the distinctive morphology and size of the bacterium observed within the SA, AX, and AX-SA composite matrices. The SA and AX-SA matrices demonstrated a dispersed structure, while the AX matrix exhibited a smooth microstructure with internal cavities where bacteria were trapped, and their distribution on the surface was uniform. These study findings align with the observations made by Mendez-Encinas et al.[Citation21] who identified AXG-based matrices as promising materials for encapsulating and safeguarding probiotic bacteria, enabling targeted delivery to the colon.
Stability of encapsulated microbeads in simulated gastric condition
presents the average outcomes indicating the survival rate of L. rhamnosus microbeads, whether free or encapsulated when subjected to simulated gastric conditions (SGC) in various treatments. The study clearly demonstrates a significant reduction (p < .05) in the viability of both free and microencapsulated probiotics. Specifically, the viability of free cells notably declined under simulated stomach conditions at pH 2.0. The survival rate peaked at 0 minutes (9.9) and reached its lowest point at 120 minutes (none). However, when encapsulated with Arabinoxylan-Sodium alginate (AX-SA), the viability decreased from 9.9 to 5.2 log cfu/g. Similarly, encapsulation with Arabinoxylan (AX) resulted in a decrease from 9.9 to 4.8 log cfu/g, while encapsulation with sodium alginate led to a viability decrease to 4.7 log cfu/g. Arabinoxylan-Sodium alginate (AX-SA) microbeads exhibited greater protection compared to microbeads comprising Arabinoxylan (AX) and Sodium alginate (SA). To achieve the intended advantages of probiotics, it is essential to ensure the viability of cells in both stomach and intestinal conditions. The survival rate of L. rhamnosus in all treatments exhibited a significant decrease during the initial 30 minutes under simulated conditions, followed by a gradual reduction until reaching 120 minutes of exposure to Stimulated Gastric Conditions. These findings align with the findings of Çabuk et al.[Citation24] who observe that probiotics had a higher survival rate when encapsulated in sodium alginate. On the other hand, poor survival rates were observed for unencapsulated cells. These findings clearly indicate that encapsulation plays a crucial role in protecting probiotics under simulated intestinal conditions.
Table 2. The viability of free probiotic and encapsulated (MSA, MAX, and MAX-SA) micro-beads.
Survival of free and encapsulated microbeads in simulated intestinal conditions
The collective results illustrate the viability rates of L. rhamnosus microbeads, both in their free form and encapsulated, when subjected to simulated intestinal conditions (SIC) across different treatments, as depicted in . Upon exposure to elevated pH levels, the viability of free cells declined steadily, starting from an initial count of 9.9 log cfu/g and reaching complete eradication within a time span of 120 minutes. However, when encapsulated with Arabinoxylan-Sodium alginate (AX-SA), the viability decreased from 9.9 to 5.4 log cfu/g. Similarly, encapsulation with Arabinoxylan resulted in a decrease from 9.9 to 4.9 log cfu/g, while encapsulation with sodium alginate led to a viability decrease to 4.8 log cfu/g. Arabinoxylan-Sodium alginate (AX-SA) micro-beads exhibited greater protection compared to micro-beads comprising Arabinoxylan (AX) and Sodium alginate (SA). Maintaining cell viability under stomach and intestinal conditions is crucial to derive the desired benefits of probiotics. The survival rate of L. rhamnosus in all treatments exhibited a significant decrease during the initial 30 minutes under simulated conditions, followed by a gradual reduction until reaching 120 minutes of exposure to SIC. The results of this study are consistent with the findings of Moumita et al.[Citation25] who also reported reliable survival of probiotics in the gastrointestinal tract. In their research, lactic acid bacteria were encapsulated alongside prebiotics, and the viability of both free and encapsulated probiotics was assessed under simulated gastrointestinal conditions and storage. Their findings revealed a viability range of 72%–87% even in challenging environments.
Table 3. Stability of encapsulated microbeads in simulated intestinal condition.
Probiotic (L. rhamnosus GG) viability in encapsulation under storage conditions due to their beneficial effects on health, probiotic bacteria can be added to food, and many of these items are kept at a storage (3 to 5°C) temperature. Results regarding the probiotic count during storage of AX-SA, AX, and SA under different storage conditions were depicted in . The statistical findings for each treatment and their interaction were extremely significant (p < .05). During storage at temperatures between 3 to 5°C, the count of encapsulated L. rhamnosus in AX-SA, AX, and SA matrices exhibited a downward trend from day one to day 28. The results indicate a decrease of approximately 2 logs CFU/g in the probiotic count by the end of the experiment. The addition of AX to the matrix had a beneficial impact by strengthening the polymer network of the matrix through the formation of covalent cross-linking facilitated by laccase treatment. This resulted in the highest probiotic count observed in the AX-SA probiotic bacteria. This result correlates with the results of another study, in which sodium alginate and chitosan were used to coat the probiotic bacteria, and a decreasing trend was observed in the mortality of microencapsulated Bifidobacterium bifidum cells compared to free probiotic cells (Pourjavid et al.).[Citation26]
Table 4. Storage of encapsulated probiotics at 5°C.
Another study by Chaluvadi et al.[Citation27] revealed that cross linking in the calcium alginate synbiotic matrices led to a notable reduction in the number of viable probiotic bacterial cells after 21 days of storage. The cross-linked matrices exhibited a count of less than 3 logs CFU/mL, whereas the non-cross-linked matrices maintained a survival rate of over 7 logs CFU/mL at the same time point. According to this study’s findings, the AX wall material may be an effective matrix for delivering probiotics because it has no negative impact on bacterial survival.
Conclusion
The study has shown that the use of Arabinoxylan (AX) combined with sodium alginate (SA) can enhance the viability of probiotic bacteria, such as Lactobacillus rhamnosus GG, under stressed conditions. Encapsulating probiotics in micro-beads made from AX and SA protected them effectively, resulting in higher viability when compared to free probiotics in simulated gastrointestinal conditions. Fortified pineapple juice containing encapsulated probiotics had a higher probiotic count, indicating the practical applications of these micro-capsules in the food industry. The morphological, molecular, and structural analyses using Fourier transform infrared spectroscopy (FTIR) and Scanning electron microscopy (SEM), valuable insights were gained into the characteristics of the micro-beads. The observation of specific functional groups in the FTIR spectra confirmed the successful encapsulation of probiotics within the micro-capsules. AX is bio-compatible, abundant, and easily modified, making it a compelling candidate for applications in the food and agriculture sector. MAX-SA micro-beads, in particular, were highly efficient in maintaining probiotic viability, suggesting their potential as a valuable tool in the development of functional foods and dietary supplements aimed at promoting gut health. As the demand for probiotic-enhanced products continues to grow, the findings of this study offer a promising avenue for further exploration and commercialization of AX-based probiotic encapsulation technologies.
Acknowledgments
The authors appreciate the support from the Researchers Supporting Project number (RSPD2024R1073), King Saud University, Riyadh, Saudi Arabia.
Disclosure statement
No potential conflict of interest was reported by the author(s).
References
- Zhang, L. D. H. Recent Advances in Probiotics Encapsulation by Electrospinning. ES Food Agrofor. 2020, 2, 3–12.
- Murua‐Pagola, B.; Abadía‐García, L.; Vázquez‐Aguilar, M. I.; Amaya‐Llano, S. L.; Morales‐Sánchez, E. The Effect of Incorporating Microencapsulated Lactobacillus Casei ATCC 334 on the Physicochemical and Rheological Properties of Fortified Greek‐Style Yoghurt. Int. J. Dairy Technol. 2024, 77(2), 477–486. DOI: 10.1111/1471-0307.13047.
- Nami, Y.; Kiani, A.; Elieh‐Ali‐Komi, D.; Jafari, M.; Haghshenas, B. Impacts of Alginate–Basil Seed Mucilage–Prebiotic Microencapsulation on the Survival Rate of the Potential Probiotic Leuconostoc mesenteroides ABRIINW. N18 in Yogurt. Int. J. Dairy Technol. 2023, 76(1), 138–148. DOI: 10.1111/1471-0307.12909.
- Sehrawat, R.; Abdullah, S.; Khatri, P.; Kumar, L.; Kumar, A.; Mujumdar, A. S. Role of Drying Technology in Probiotic Encapsulation and Impact on Food Safety. Drying. Technol. 2022, 40(8), 1562–1581. DOI: 10.1080/07373937.2022.2044844.
- Chen, Z.; Li, S.; Fu, Y.; Li, C.; Chen, D.; Chen, H. Arabinoxylan Structural Characteristics, Interaction with Gut Microbiota and Potential Health Functions. J. Funct. Foods. 2019, 54, 536–551. DOI: 10.1016/j.jff.2019.02.007.
- Eor, J. Y.; Son, Y. J.; Kim, S. H. The Anti‐Inflammatory and Anti‐Oxidative Potential of Synbiotics in Two Independent Cell Lines. Int. J. Dairy Technol. 2021, 74(3), 518–527. DOI: 10.1111/1471-0307.12777.
- Ozcan, T.; Eroglu, E. Effect of Stevia and Inulin Interactions on Fermentation Profile and Short‐Chain Fatty Acid Production of Lactobacillus acidophilus in Milk and in vitro Systems. Int. J. Dairy Technol. 2022, 75(1), 171–181. DOI: 10.1111/1471-0307.12814.
- Bach Knudsen, E. K. Microbial Degradation of Whole-Grain Complex Carbohydrates and Impact on Short-Chain Fatty Acids and Health. Adv. Nutr. An Int. Rev. J. 2015, 6(1), 206–213. DOI: 10.3945/an.114.007450.
- Nino-Medina, G.; Carvajal-Millán, E.; Rascon-Chu, A.; Marquez-Escalante, J. A.; Guerrero, V.; Salas-Munoz, E. Feruloylated Arabinoxylans and Arabinoxylan Gels: Structure, Sources and Applications. Phytochem. Rev. 2010 , 9(1), 111–120. DOI: 10.1007/s11101-009-9147-3.
- Hernández-Pinto, F. J.; Miranda-Medina, J. D.; Natera-Maldonado, A.; Vara-Aldama, Ó.; Ortueta-Cabranes, M. P.; Del Mercado-Pardiño, J. A. V.; El-Aidie, S. A. M.; Siddiqui, S. A.; Castro-Muñoz, R. Arabinoxylans: A Review on Protocols for Their Recovery, Functionalities and Roles in Food Formulations. Int. J. Biol. Macromol. 2024, 259, 129309. DOI: 10.1016/j.ijbiomac.2024.129309.
- Neyrinck, A. M.; Possemiers, S.; Druart, C.; van de Wiele, T.; de Backer, F.; Cani, P. D.; Larondelle, Y.; Delzenne, N. M.; Brennan, L. Prebiotic Effects of Wheat Arabinoxylan Related to the Increase in Bifidobacteria, Roseburia and Bacteroides/Prevotella in Diet-Induced Obese Mice. PLOS ONE. 2011, 6(6), e20944. DOI: 10.1371/journal.pone.0020944.
- González-Estrada, C.-S. M.; Ascencio Valle, E.; Carvajal-Millan, F. J.; Ragazzo-Sánchez, J. A.; Rascón-Chu, F.; Brown-Bojorquez, A.; Rascón-Chu, A. Covalently Cross-Linked Arabinoxylans Films for Debaryomyces hansenii Entrapment. Molecules 2015, 20(6), 11373–11386. DOI: 10.3390/molecules200611373.
- Morales-Ortega, A.; Carvajal-Millan, E.; Brown-Bojorquez, F.; Rascón-Chu, A.; Torres-Chavez, P.; López-Franco, Y.; Lizardi-Mendoza, J.; Martínez-López, A.; Campa-Mada, A. Entrapment of Probiotics in Water Extractable Arabinoxylan Gels: Rheological and Microstructural Characterization. Molecules. 2014, 19(3), 3628–3637. DOI: 10.3390/molecules19033628.
- Carvajal-Millan, E.; Vargas-Albores, F.; Fierro-Islas, J. M.; Gollas-Galván, T.; MagdalenoMoncayo, D.; Rascon-Chu, A.; Martínez-Porchas, M.; Lago-Lestón, A. Arabinoxylans and Gelled Arabinoxylans Used As Anti-Obesogenic Agents Could Protect the Stability of Intestinal Microbiota of Rats Consuming High-Fat Diets. Int. J. Food Sci. Nutr. 2019, 71(1), 74–83. DOI: 10.1080/09637486.2019.1610729.
- Yingying, Z.; Lisha, Y.; Yan, D.; Huajun, W.; Xuewei, F.; Xiao, Y.; Ruiling, S. Effect of Co-Microencapsulation of Non-Starch Polysaccharides from Highland Barley and Lactobacillus Plantarum on the Survival of Probiotics. J. Chin. Inst. Food Sci. Amp; Technol. 2023, 23(11), 266.
- Yeung, T. W.; Arroyo-Maya, I. J.; Dj, M.; Sela, D. A Microencapsulation of Probiotics in Hydrogel Particles: Enhancing Lactobacillus lactis Subsp. Cremoris LM0230 Viability Using Calcium Alginate Beads. Food Funct. 2016, 7(4), 1797–1804. DOI: 10.1039/C5FO00801H.
- Cedran, M. F.; Rodrigues, F. J.; Bicas, J. L. Encapsulation of Bifidobacterium BB12® in Alginate-Jaboticaba Peel Blend Increases Encapsulation Efficiency and Bacterial Survival Under Adverse Conditions. Appl. Microbiol. Biotechnol. 2021, 105(1), 119–127. DOI: 10.1007/s00253-020-11025-9.
- Ji, Y. R.; Choi, S.; Lee, M. J.; Cho, Y.; Cho, Y. Microencapsulation of Probiotic Lactobacillus acidophilus kbl409 by Extrusion Technology to Enhance Survival Under Simulated Intestinal and Freeze-Drying Conditions. J. Microbiol. Biotechnol. 2019, 29(5), 721–730. DOI: 10.4014/jmb.1903.03018.
- Araújo Etchepare, M.; Nunes, G. L.; Nicoloso, B. R.; Barin, J. S.; Flores, E. M. M.; de Oliveira Mello, R.; de Menezes, C. R. Improvement of the Viability of Encapsulated Probiotics Using Whey Proteins. LWT. 2020, 117, 108601. DOI: 10.1016/j.lwt.2019.108601.
- Wu, Y.; Zhang, G. Synbiotic Encapsulation of Probiotic Lactobacillus Plantarum by Alginate -Arabinoxylan Composite Microspheres. LWT Food Sci. Technol. 2018, 93, 135–141. DOI: 10.1016/j.lwt.2018.03.034.
- Mendez-Encinas, M. A.; Carvajal-Millan, E.; Simon, S.; White, A. K.; Chau, H. K.; Yadav, M. P.; Valencia-Rivera, D. E.; Hotchkiss, A. T.; Rascon-Chu, A.; Astiazaran-Garcia, H. Arabinoxylans and Cross-Linked Arabinoxylans: Fermentation and Potential Application As Matrices for Probiotic Bacterial Encapsulation. Food Hydrocoll. Health 2022, 2, 100085. DOI: 10.1016/j.fhfh.2022.100085.
- Praepanitchai, O. A.; Noomhorm, A.; Anal, A. K. Survival and Behavior of Encapsulated Probiotics (Lactobacillus Plantarum) in Calcium-Alginate-Soy Protein Isolate-Based Hydrogel Beads in Different Processing Conditions (pH and Temperature) and in Pasteurized Mango Juice. Biomed Res. Int. 2019, 2019. DOI: 10.1155/2019/9768152.
- Hadidi, M.; Jafarzadeh, S.; Ibarz, A. Modified Mung Bean Protein: Optimization of Microwave-Assisted Phosphorylation and Its Functional and Structural Characterizations. LWT. 2021, 151, 112119. DOI: 10.1016/j.lwt.2021.112119.
- Çabuk, B.; Harsa, Ş. T. Protection of Lactobacillus Acidophilus NRRL-B 4495 Under in vitro Gastrointestinal Conditions with Whey Protein/Pullulan Microcapsules. J. Biosci. Bioeng 2015, 120(6), 650–656. DOI: 10.1016/j.jbiosc.2015.04.014.
- Moumita, S., Goderska, K., Johnson, E. M., Das, B., Indira, D., Yadav, R., Kumari, S., & Jayabalan, R. Evaluation of the Viability of Free and Encapsulated Lactic Acid Bacteria Using Invitro Gastro Intestinal Model and Survivability Studies of Synbiotic Microcapsules in Dry Food Matrix During Storage. LWT 2017, 77, 460–467. DOI: 10.1016/j.lwt.2016.11.079.
- Pourjavid, H.; Ataee, M.; Pourahmad, R.; Anvar, A. A.; Behmadi, H. Changes in Microbial, Rheological, and Sensory Characteristics of Probiotic Yogurt Sauce Containing Lactobacillus Rhamnosus During Cold Storage. J. Food Biosci. Technol. 2023, 13(2), 27–38.
- Chaluvadi, S.; Jr, A. T. H.; Call, J. E.; Luchansky, J. B.; Phillips, J. G.; Liu, L. S.; Yam, K. L. Protection of Probiotic Bacteria in a Synbiotic Matrix Following Aerobic Storage at 4°C. Benefic. Microbes 2012, 3(September), 175–187. DOI: 10.3920/BM2012.0005.