ABSTRACT
Anthocyanins are natural pigments that exhibit a wide range of protective effects with potential benefits for human health related to their antioxidant activities. Grape berries are rich anthocyanins, and these compounds have considerable influence on wine quality. However, these pigments are known to only be present in coloured grape berries. In the present study, we investigated three distinctive grapes, in which only a few reports of mutant strains on the skin colour of the grape berry were reported. Anthocyanin and non-anthocyanin phenolic profiles of grape berry skin, pulp, and seeds at different growth stages were analysed using high performance liquid chromatography of flight mass spectrometry (HPLC-ESI-MS/MS). Meanwhile, grape antioxidant properties were evaluated at growth stages, including the early stage, veraison, and mature stage. The results showed that some anthocyanins contents, such as malvidin 3-O-glucoside, are significantly different between white skin grapes and their corresponding coloured genotypes, with high content in coloured but not in white skin grapes. However, other anthocyanins, such as delphinidin 3-O-glucoside, showed no significant differences between white skin grapes and their corresponding coloured genotypes. Moreover, non-anthocyanin phenolic profiles and antioxidant properties, except cultivars Pinot Blanc and Pinot Noir, showed no significant differences between white skin grapes and their corresponding coloured genotypes at any growth stage, including the pulp and seeds. However, significant differences were noted between different cultivars, for example, white-ciputao and Pinot Noir, Pinot Blanc and Muscat Rouge, and coloured-ciputao and Muscat Blanc, showing that the antioxidant capacity was not always in correlation with skin colour of grape berries.
Introduction
It is well known that grape extracts and wine have high antioxidant capacities, and these correlate well with their anthocyanin contents [Citation1]. Recently, there has been considerable interest in the possible beneficial effects of anthocyanins on human health. Anthocyanins, which make up the majority of the flavonoids, exhibit strong antioxidation activities that can reduce the risk of cardiovascular disease, oxidative stress, and diabetes. They also may have anticarcinogenic effects and reduce the induction of apoptosis in tumour cells [Citation2–4]. Many studies have suggested that various fruits, especially berries, contain high anthocyanin levels [Citation5]. Anthocyanins are the most abundant polyphenolic compounds in coloured grapes, and grape skin colour varies mainly due to the anthocyanin composition and content [Citation6]. White-, red-, purple-, or black-coloured grapes are some of the most important sources of anthocyanins. The anthocyanin pigments derived from grapes are mainly found in red wine and in the coloured species from veraison to maturation [Citation7,Citation8]. However, anthocyanin compounds have been detected in white skin grape berries when they are mature [Citation9–11], although it is generally thought that there are no anthocyanin pigments in white-grape berries [Citation12]. Moreover, a previous report suggested that white-grape cultivars might synthesize anthocyanins during the final period of ripening [Citation13]. However, the report did not compare the differences in anthocyanin profiles and content between white and coloured grapes. Therefore, these results confirm differences in antioxidant capacities between different cultivars, but not between grape-skin colours.
In this study, we investigated three distinctive cultivars. Each cultivar contained the same genetic background, but one cultivar produced white grapes, whereas the other corresponding cultivars produced black or red grapes. The aim was to explore the anthocyanin composition, characteristics, and contents during growth, to determine the antioxidant capacities of white skin grapes compared to their corresponding coloured genotypes.
Materials and methods
Materials
We selected three grape cultivars that had both coloured- and white-grape varieties. The coloured and white skinned cultivars had relatively similar genetic backgrounds. The Chinese wild grape Vitis davidii was the only species that contained a white-ciputao and a coloured-ciputao grape type [Citation14]. Vitis vinifera Pinot Blanc, a white grapevine, is thought to be a white skinned mutant of the red-grapevine cultivar Pinot Noir [Citation15], and the red grapevine Muscat Rouge is a mutant of the white grapevine Muscat Blanc [Citation16]. We used growth curves, to choose the representative growth stages for this study. These were 40–60 (early stage of growth), 80–90 (veraison), and 100–120 (ripening stage of growth) days after anthesis (DAA) (). All samples were planted in the vineyards of the National Grape Germplasm Repository, Zhengzhou Fruit Research Institute of the Chinese Academy of Agricultural Sciences (113° 67′ E, 34° 75′ N). At each growth stage, about 100 grapes were collected from each individual cultivar type. After washing in deionized water, all the skin, pulp, and seeds were removed and macerated in liquid nitrogen, then stored at –80 °C for further processing. The samples were replicated three times for each cultivar.
Chemicals
The anthocyanin standards were purchased from Phytolab (Phytolab, Vestenbergsgreuth, Germany), and included cyanidin 3-O-glucoside (CyG), cyanidin 3-O-glucoside-5-O-glucoside (Cy2G), delphinidin 3-O-glucoside (Dp), malvidin 3-O-glucoside (Mv), pelargonidin 3-O-glucoside (Pg), pelargonidin 3-O-glucoside-5-O-glucoside (Pg2G), and petunidin 3-O-glucoside (Pt). The non-anthocyanin phenolic standards included epicatechin (EC), coumaric acid (CO), phlorizin (PO), taxifolin (TO), quercetin (QC), myricitrin (MC), caffeic acid (CA), gallic acid (GC), and 2,2-diphenyl-1-picrylhydrazyl (DPPH), 6-hydroxy-2,5,7,8-tetramethylchroman-2-carboxylic acid (trolox), and neocuproine were obtained from Sigma (Sigma, USA). The other chemicals used were analytical or high performance liquid chromatography (HPLC) grade.
Anthocyanin and polyphenol extract preparation
Anthocyanins and polyphenols were extracted as described by Liang et al. [Citation10]. The frozen grapes were crushed using a mortar and pestle and the powdered samples were ground in 2 mL of extract solution (2:28:70, formic acid:water:methanol). The extracts were then shaken for 10 min in a thermo mixer (Eppendorf, USA), and centrifuged at 13,000 ×g at 4 °C for 10 min. About 1 mL of extract was filtered through a 0.2 µm membrane filter (Agilent Technologies, USA) for analysis.
Anthocyanins and non-anthocyanin phenolics detected by HPLC-ESI-MS/MS
HPLC of flight mass spectrometry Triple Quad LC-MS/MS, (Agilent 6460B, USA) was used to identify the anthocyanins, and a ZORBAX SB-C18 column (100 mm × 2.1 mm, 1.8 µm) was used for the analysis. The system was coupled directly to the sprayer needle where ions were generated by electrospray ionization (ESI) in both positive and negative ionization modes. The mobile phase consisted of water:formic acid (99:1) as solvent A, and acetonitrile as solvent B. The gradient profile began at 94% solvent A, to 60% A at 2 min, 20% A at 3 min, then A went back to 94% at 5 min, and was kept there for 1.0 min. Non-anthocyanin phenolics after the following adjustments had been made: the gradient profile began at 90% solvent A, to 50% A at 2 min, 10% A at 3 min, then A went back to 90% at 5 min, and was kept there for 1 min. The flow rate was 0.3 mL min−1 and the column temperature was set at 30 °C. The injection volume was 5 µL. In the MS analyses, nitrogen was used as the drying and nebulizing gas and the nebulizer pressure was 275,790 Pa. Gas flow was set at 10 L·min−1 and temperature was 350 °C. Data were collected and analysed using MassHunter data acquisition and processing software (Agilent, USA).
Antioxidant assays
Antioxidant assays were used to accurately evaluate the antioxidant properties of the grape samples, including DPPH free radical-scavenging activity, cupric-reducing antioxidant capacity (CUPRAC), and hydroxyl radical-scavenging activity (HRSA). In this study, DPPH assays were analysed according to Brandwilliams [Citation17], CUPRAC according to Apak [Citation18], and HRSA according to Sroka [Citation19].
Statistical analysis
The data were reported as the mean ± standard error (SE) from three replications, and were analysed using SPSS 19.0 (SPSS, USA). Principal component analysis (PCA) of anthocyanins during growth stages were analysed using SPSS 19.0 (SPSS, USA).
Results and discussion
Anthocyanin identification
Anthocyanins are water-soluble pigments that consist of an anthocyanidin aglycone and a sugar moiety. Most common sugars (galactose, glucose, arabinose, xylose, and rhamnose) bond to anthocyanidins in mono-, di-, or trisaccharide forms, together with their acylated (acetyl and coumaryl) derivatives. There are hundreds of different anthocyanins in red wine [Citation20,Citation21]. However, the 3-O-glucosides, malvidin, delphinidin, petunidin, peonidin (Pn), pelargonidin, and cyaniding (), are the most frequently detected in grapes [Citation22,Citation23].
All the anthocyanin components were detected from the early growth (P1) to maturity (P5) in grape skin ((A–C)). Mv was also present in coloured grapes (D2, V2, and V4) from veraison (P3) to maturity (P5). It was the most abundant anthocyanin component at P5 in black skin grapes, accounting for 47.8% and 49.5% of total anthocyanins in D2 and V2. However, in V4 red skin grapes, the highest Mv level was only 8.4%. There were the significant differences (p < 0.05) in Mv concentrations between the different grape cultivars and types. At P5, D2 contained 7.14 ± 0.28 mg kg−1 fresh weight (FW) Mv, and total anthocyanins amounted to 14.94 ± 0.28 mg kg−1 FW. However, V2 and V4 contained 180.79 ± 5.52 and 0.90 ± 0.03 mg kg−1 FW of Mv, respectively, and the total anthocyanin contents were 365.94 ± 11.84 and 9.17 ± 0.29 mg kg−1 FW, respectively. Furthermore, the Mv concentration in the skin increased sharply as the grapes matured. These results are in agreement with previous studies [Citation24,Citation25]. Anthocyanin pigments in grapes are mainly restricted to the coloured types and accumulate between veraison and maturity. However, changes in anthocyanin type and concentration in grapes during the early growth stages have not been reported.
The most abundant anthocyanin components in skin were Cy and its derivatives, whose contents were highest during P5 in red skinned grapes, accounting for 71.4% of the total anthocyanin content in V4, and more than 80% in white grapes. There were large differences in Cy and its derivative contents between the different types of grapes. From stages P1 to P5, the Cy content always increased, except for D2 and V4, whose skin Cy content began to decrease at veraison (P3), a critical period for grape development. The Cy contents of the white grape cultivar, D1 were always higher than that in its black skinned counterpart, D2, but Cy in the white skinned V3 Muscat cultivar was lower than in its red skinned counterpart, V4. The contents in the V1 grape peel were higher than in V2 before veraison (P3), and then lower than V2 until P5. The CyG contents in the V. vinifera coloured grapes (V2 and V4) was several-fold higher than in the non-coloured grapes (V1 and V3) from stages P3 to P5, and the Cy2G contents were significantly lower than the CyG contents. In contrast, in the Chinese wild grapevine V. davidii, the Cy2G contents in the black skinned cultivar (D2) were higher than in the counterpart white skin grapes (D1), and Cy2G was significantly higher than CyG.
Another abundant anthocyanin component was Dp, whose content was several-fold higher than the other compounds in peel at stage P1 for both the black/red skinned grapes and the white skinned grapes. The Dp contents increased as the grapes developed, except for D2 and V4, which decreased slightly at P5. Between stages P1 and P5, the skin Dp content of the white skinned grapes was significantly lower than in the black/red skinned grapes. The Pt content trends were similar to the Dp, contents, especially in V2. In addition, Pn was detected in all the grape types, but the coloured grape contents were significantly higher than white grape contents, and they significantly increased from the P3 stage onwards.
Interestingly, Pg was not detected in any of the grape skin samples, but Pg2G was detected in all the grape types. It was strange that the Pg2G content hardly changed as grape development progressed, and that there were no differences between the white skinned grapes and the black/red skinned grapes. The mean concentration was 0.100–0.200 mg kg−1 FW.
It is commonly accepted that the concentration varies greatly, especially between different grape species and cultivars, and that the anthocyanin profile of a given cultivar is related to its genetic background [Citation26]. Although many grape varieties have coloured or white skinned berries, only few genotypes have both. Presently, there are only limited reports of mutant strains of grape skin colour, for example, Italia and Benitaka [Citation27], Pinot Noir and Pinot Blanc [Citation15], and Muscat Rouge and Muscat Blanc [Citation16]. V. davidii was the only species detected with a white skinned berry genotype in Chinese wild grape [Citation14]. In this study, coloured and white skinned grapes had relatively similar genetic backgrounds. Moreover, all grape berries were harvested from the same vineyard, and there were relatively consistent environmental and microclimatic factors. Thus, these results showed the differences of grape-berry skin colour.
From the early growth stages to maturity, no anthocyanin components were detected in the pulp of either white skin grapes or their coloured counterparts. This study's results are consistent with previous articles [Citation24].
The anthocyanin components of grape seeds were detected in the same way as they were in the skin samples. The total anthocyanin levels in seeds are shown in (D)–2(F). Generally, total anthocyanin levels decreased sharply from the early development stage (Z1) to the veraison stage (Z3), and then increased slightly until they reached the mature stage (Z5). There were no significant differences in seed anthocyanin contents between the V. vinifera coloured grape cultivars and their white grape counterparts, but the total anthocyanin levels in Chinese wild V. davidii D2 were significantly higher (p < 0.05) than in D1 before the veraison stage (Z3). The main anthocyanin compounds in the seeds were Cy and CyG. The Cy and CyG contents were several-fold higher than other compounds during early development. Total anthocyanin contents decreased sharply after veraison, and were much lower at maturity than they were during early growth. Mv was only detected in seeds of black skinned grapes during the mature stage, which was similar to the peel results, but the seed contents were far lower than the peel contents. The contents of other individual anthocyanin compounds, such as Dp, Pn, and Pt, were all lower than Cy and CyG, especially at the early grape development stage. We were surprised that PgG was detected in seeds, but it was only detected at Z1, and only in V1 and V2, and black V. davidii (D2). The Pg2G results were similar to the skin results.
Identification of non-anthocyanin phenolics
In addition to the anthocyanins, non-anthocyanins phenolics, such as flavonoids, stilbenes, phenolic acids, and tannins, are also considered to be major contributors to antioxidant activity in grapes [Citation28]. Therefore, we used HPLC-ESI-MS/MS to accurately characterize and evaluate several major flavonoid compounds and phenolic acids in the coloured and white skin grapes ().
The compounds present and the total phenolic contents in grape skin at the different growth stages are shown in (A)–3(C). The total phenolic contents of both the white and coloured grapes increased slightly from the early growth stage (P1) to the ripening stage (P5). There were no significant differences for total phenolics between the white and coloured grape cultivars and there were no significant differences among the individual grape cultivars. However, there were significant differences (p < 0.05) in individual phenolic compounds between coloured grapes and their white skin counterparts. Moreover, there were also compound differences among the cultivars. The first major compound was EC, which was the most abundant phenolic compound, and accounted for 37.08%–56.67% and 38.42%–66.15% of the total phenolics in V1 and V2 peels. However, in other cultivars, the highest proportion was no more than 30%. Furthermore, only V2 was higher than V1 from early growth stage to maturity, especially during the early stages. In the other cultivars, white skinned grapes contained slightly more than the coloured grapes. The next most abundant phenolic compound was MC, whose contents increased as grape development progressed, except D2 and V4, where it decreased slightly at the ripening stage. From early growth to maturity, the MC content in D2 black skinned grape skin was higher than in their counterpart D1 white skinned grapes. However, for V1 and V2, the results were reversed, and there were no any differences between V3 and V4. Another abundant phenolic component was CO, whose content increased as grape development progressed. There was no difference between growth stages, except for V1 and V2, where CO decreased gradually from the early growth stage onwards until no CO could be detected from the veraison stage (P3) and beyond in V1. There were no differences in CA, a major phenolic acid in grapes, between coloured skinned grapes and white skinned grapes for all growth stages, but there were differences among cultivars. In Chinese wild V. davidii, the contents remained at 2.1 ± 0.08–3.1 ± 0.11 mg kg−1 FW from early growth to the ripening stage. However, the CA contents decreased from 0.6 ± 0.02 and 1.5 ± 0.05 mg kg−1 FW to 0.1 ± 0.01 and 0.5 ± 0.02 mg kg−1 FW in V1 and V2, and V3 and V4, respectively. In addition, PO and QC were detected in all grape cultivars, and the contents similarly increased slightly from early growth (0.1 ± 0.01 mg kg−1) FW to the ripening stage (0.5 ± 0.02 mg kg−1) FW. There were no differences between coloured grapes or white grapes. GC gradually increased in V1 and V2, and the GC contents were higher in V2 than in V1 after the veraison stage, but were lower in other cultivars, especially in D1, where it was not detected at all at veraison. Taxifolin (TO), a plant flavonoid that modulates hepatic lipoprotein synthesis and secretion, was detected [Citation29], but at a low level. The contents were no more than 0.1 mg kg−1 FW, and it was only detected at the ripening stage in D2 and during all growth stages in V2.
Although there were no anthocyanin compounds detected, phenolic compounds were abundant in the pulp of grape berries. (D–F) shows that the main phenolic compound was EC, which was the same as in skin. In D2, EC contents decreased from 14.08 ± 0.48 mg kg−1 FW at the early growth stage (R1) to 2.01 ± 0.08 mg kg−1 FW at ripening (R5), and accounted for 87.8%–63.7% of the total phenolics present. In D1, it decreased from 4.73 ± 0.18 to 1.96 ± 0.07 mg kg−1 FW, and accounted for 43.0%–33.4% of the total phenolic content. In contrast, in V2, the EC contents increased from 2.92 ± 0.09 mg kg−1 FW at R1 to 13.70 ± 0.41 mg kg−1 FW at R4, and then decreased to 10.32 ± 0.38 mg kg−1 FW at R5. However, its proportion of the total phenolics remained at around 88% throughout all the growth stages in D1. In V1, it increased from 2.88 ± 0.08 mg kg−1 FW (R1) to 17.34 ± 0.58 mg kg−1 FW (R4), but then decreased to 6.61 ± 0. mg kg−1 FW (R5). It accounted for 75.0%–96.0% and 89.1%, respectively, of the total phenolic content. There were no significant differences in the EC contents for V3 and V4, but the EC contents insignificantly decreased from early growth to ripening, which was the same as D2.
In addition to EC, the other phenolics detected in peel were almost all detected in the pulp, but the contents were far below EC levels, except for CO contents in D1, which were as high as EC in general, and higher than EC after the veraison stage. This may suggest that there are differences between the phenolic compound contents in the Chinese wild V. davidii red and white types.
(G–I) shows that the EC contents were much higher than the other compounds in seeds. Other phenolic compounds were detected at all the grape growth stages, including CO and taxifolin, which were not detected in the pulp. From early growth (Z1) to ripening (Z5), the EC content change trends were similar for all cultivars. There were much higher levels during early growth (Z1), which fell sharply after the veraison (Z3) stage. However, it should be pointed out that the EC contents of the seeds among the different cultivars were different. Not all of the coloured grape seeds contained higher EC contents than the white grape seeds.
Additionally, anthocyanin and non-anthocyanin phenolics, such as flavonoids, stilbenes, phenolic acids, and tannins, are also considered to be primary contributors to grape antioxidant activity [Citation28]. However, no correlations were found between the total phenolic content in grape skins and their antioxidant capacities [Citation30]. Therefore, although the antioxidant capacity was determined for the grape extract, the different phenolic compounds between white skinned grapes and their coloured genotypes could not be determined using the total phenolic content. It is, therefore, necessary to confirm the phenolic composition. Most research reports on the phenolic composition of grapes indicate that the most abundant monomeric flavonoids are catechin, EC, GC, and CA [Citation1,Citation10], also CO, PO, quercetin, and MC were closely related to the biosynthesis of plant anthocyanins [Citation31]. To accurately determine phenolic composition of the different grape genotypes, HPLC-ESI-MS/MS was performed. In contrast to the anthocyanin content, the non-anthocyanin content of grape berries was high from the early growth stages, including in the skin, pulp, and seeds (). Significant differences of both anthocyanin and non-anthocyanin contents were noted in different cultivars, especially in the skin. These results confirmed recent reports that anthocyanins are abundant in coloured grapes [Citation6,Citation12]. However, no significant differences were noted in the phenolic composition and content at any growth stages between white skin grapes and their coloured genotypes. Therefore, despite having the same skin colour, significant differences were noted in the antioxidant properties of different cultivars. However, if the grapes had different skin colours, the results may suggest that the differences were due to skin colour and not based on the cultivar. As a result, these coloured grapes have become more popular, but it is unfair for white skin grapes.
Antioxidant assays
The HPLC-ESI-MS/MS results indicated that Mv was not detected in white skin grapes. Its content was highest in the black skin grapes at the ripening stage, and it was the main contributor to colour in grape skins. Antioxidant assays have traditionally been used to test the antioxidant capacity of selected common vegetables [Citation32]. shows that only the coloured V2 antioxidant capacity was significantly higher than white V1 in skin after veraison. Furthermore, antioxidant capacities in the coloured D2 and V4 cultivars were no higher at any growth stages than in their white skinned counterparts, D1 and V3. This included skin, pulp, and seeds. These results indicated that not all coloured grapes had higher antioxidant capacities than white grapes. The DPPH, CUPRAC, and HRSA results were similar to each other when the antioxidant capacities in different growth stages were compared. Antioxidant capacities were significantly higher (p < 0.05) at maturity than during early growth in skin. However, there were no differences between the different coloured grapes for pulp, and only the CUPRAC antioxidant results for seeds were significantly higher after veraison. This antioxidant capacity results were slightly different from the results produced by previous studies [Citation33]. We speculate that this dissimilarity was due to the differences of cultivars and conditions, such as environmental and microclimatic factors, climate [Citation34,Citation35], soil conditions, and irrigation [Citation36,Citation37], which can be responsible for the variability seen in pigment concentrations.
Table 1. Antioxidant activity determined by the DPPH, CUPRAC, and HRSA assays of grapes sampled at different growth stages.
It is well known that grape extracts and wine have high antioxidant capacities, and that the antioxidant capacities correlate well with the anthocyanin contents in grapes and wine [Citation1]. There have been many reports on the antioxidant capacities of grape. However, these results were mainly antioxidant capacity comparisons between different cultivars [Citation38]. Therefore, we selected grape varieties that have both coloured and white skin types. There are many antioxidant systems that may be involved in a multitude of complex interactions, including synergistic or antagonistic reactions [Citation39]. In fact, there is no universal assay that accurately reflects all the antioxidants in a complex system, and different antioxidant activities are analysed using different methods. In order to evaluate the antioxidant capacity in vitro, at least two complementary methods are often used at the same time [Citation33]. In this study, three antioxidant assays were applied to accurately evaluate the antioxidant properties of coloured grapes and white skin grapes: DPPH, CUPRAC, and HRSA. However, it is strange that the results for antioxidant capacity showed that not all coloured grape varieties had higher antioxidant capacities than the white grapes. However, in some individual grape varieties, the antioxidant capacity at ripening was significantly higher than during the early growth stages, especially in the skin. The white grapes also contained anthocyanin compounds, and their antioxidant capacity was no lower than the coloured grapes, which suggests that antioxidant capacity is all due to anthocyanin content. It is known that in addition to anthocyanins, the antioxidant capacity of fruits correlates well with total phenolic contents, including non-anthocyanin compounds [Citation40].
Principal component analysis
PCA was used to analyse the data for individual anthocyanins in coloured grape peel and seeds at the five different growth stages (). The results for skin were shown in (A), the first two PCs accounted for 82.5% of the total variance. PC1 and PC2 explained a relatively high percentage of the total variance (68.0% for PC1, 14.6% for PC2). PC1 was highly positively associated with higher contents of individual anthocyanins and the total anthocyanin contents during ripening, and had a positive value for all anthocyanin compounds. PC2 was positively associated with lower contents of individual anthocyanins at all stages. The PCA results showed that the main contributors to total variance were Mv and Dp contents, followed CyG and Pn contents. (B) shows the results for seeds. The first three PCs accounted for 86.5% of the total variance. PC1, PC2, and PC3 explained a relatively high percentage of the total variance (57.8% for PC1, 16.2% for PC2, and 12.5% for PC3). According to the PCA results, the main contributors to total variance were CyG, Pn, and Dp.
Figure 4. PCA of anthocyanin coloured grape profiles during growth stages for skin (A) and seeds (B).
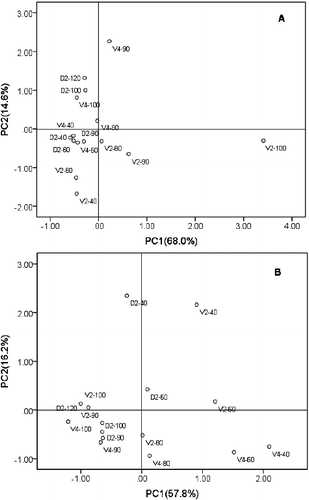
The additional evaluation of the HPLC-MS/MS data by PCA () clearly indicated that the Mv relative content variation had a key role in shaping the phenotypic distribution of grape skin colour. However, in the skin, Mv was only detected in coloured grape varieties from veraison to maturity. In contrast, although the contents were lower, the others anthocyanin compounds could be detected in all the grape cultivars and at all growth stages. For example, the Dp content in white grapes was almost the same as in coloured grapes. Furthermore, Mv was the most abundant anthocyanin component at the ripening stage in black skin grapes, accounting for 47.8% and 49.5% of the total anthocyanins in D2 and V2, respectively, However, in V4, a red skinned grape, the Mv levels only reached 8.4%, and no Mv was detected in white grapes or during the early growth stages. Thus, we tentatively suggest that the Mv content was the main factor affecting grape skin colour. In addition, the anthocyanin contents of seeds during the early growth stages were higher than at maturity, and there were differences between individual varieties. To our knowledge, this result has not been reported previously.
Conclusion
White grapes contain all the anthocyanins that are found in coloured grape berries, except for Mv. However, the anthocyanin contents were several to hundreds-fold lower in white grapes than in coloured grapes. There were no anthocyanins detected in the pulp from either white or coloured grapes, but anthocyanins were detected in the skin and seeds during the early growth stages. Meanwhile, the antioxidant capacity of grapes was not always lower in white grapes than in coloured grapes. In every grape variety, the antioxidant capacity of the various tissues at the maturity was significantly higher than during the early growth stages. The all showing that the antioxidant capacity was not always correlation with skin colour of grape berries. In short, these results will not only provide some new insights and stimulate interest in the biosynthesis and regulation of anthocyanins and flavonoids in the grape cultivars but also help us to make use of these biological resources and to exploit their production potential in grape breeding and wine making.
Acknowledgements
We wish to thank Chengkui Qiao and Chao Wang for helpful anthocyanins detected in this work.
Disclosure statement
No potential conflict of interest was reported by the authors.
Additional information
Funding
References
- Nicolas L, Patrick P, Patrice R, et al. Antioxidant capacities and phenolics levels of French wines from different varieties and vintages. J Agric Food Chem. 2001;49:3341–3348.
- Ghosh D. Potential role of polyphenol – fortified foods and beverages on vascular health. Agro Food Ind Hi-Tech. 2009;20:25–26.
- Wang LS, Stoner GD. Anthocyanins and their role in cancer prevention. Cancer Lett. 2008;269:281–290.
- Zafra-Stone S, Yasmin T, Bagchi M, et al. Berry anthocyanins as novel antioxidants in human health and disease prevention. Mol Nutr Food Res. 2007;51:675–683.
- Veberic R, Slatnar A, Bizjak J, et al. Anthocyanin composition of different wild and cultivated berry species. LWT - Food Sci Technol. 2015;60:509–517.
- Cooper-Driver GA. Contributions of Jeffrey Harborne and co-workers to the study of anthocyanins. Phytochemistry. 2001;56:229–236.
- Fritz J, Kern M, Pahlke G, et al. Biological activities of malvidin, a red wine anthocyanidin. Mol Nutr Food Res. 2006;50:390–395.4-5
- Revilla E, García-Beneytez E, Cabello F. Anthocyanin fingerprint of clones of Tempranillo grapes and wines made with them. Aust J Grape Wine Res. 2009;15:70–78.
- Arapitsas P, Oliveira J, Mattivi F. Do white grapes really exist? Food Res Int. 2015;69:21–25.
- Dietmar K, Achim C, Reinhold C, et al. Polyphenol Screening of Pomace from Red and White Grape Varieties (Vitis vinifera L.) by HPLC-DAD-MS/MS. J Agric Food Chem. 2004;52:4360–4367.
- Liang Z, Owens CL, Zhong GY, et al. Polyphenolic profiles detected in the ripe berries of Vitis vinifera germplasm. Food Chem. 2011;129:940–950.
- Mamiko S, Keiko F, Hironori K, et al. Pink-colored grape berry is the result of short insertion in intron of color regulatory gene. Plos One. 2011;66:21308–21318.
- Gholami M, Coombe BG. Occurrence of anthocyanin pigments in berries of the white cultivar Muscat Gordo Blanco (Vitis Vinifera L.). Aust J Grape Wine Res. 1995;1:67–70.
- Jiao J, Fu X, Liu C, et al. Study of the relationship between the cultivars of Vitis vinifera and the white-fruited and hermaphrodite Chinese wild grapes. Mol Breed. 2014;34:1401–1411.
- Yakushiji H, Kobayashi S, Goto-Yamamoto N, et al. A skin color mutation of grapevine, from black-skinned Pinot Noir to white-skinned Pinot Blanc, is caused by deletion of the functional VvmybA1 allele. Biosci Biotechnol Biochem. 2006;70:1506–1508.
- Kong QS. The grape of China. BeiJing: China Agricultural Scientech Press; 2004.
- Brand-Williams W, Cuvelier ME, Berset C. Use of a free-radical method to evaluate antioxidant activity. LWT - Food Sci Technol. 1995;28:25–30.
- Apak R, Guculu KG, Ozyurek M, et al. Novel total antioxidant capacity index for dietary polyphenols and vitamins C and E, using their cupric iron reducing capability in the presence of neocuproine: CUPRAC method. J Agric Food Chem. 2004;52:7970–7981.
- Sroka Z, Cisowski W. Hydrogen peroxide scavenging, antioxidant and anti-radical activity of some phenolic acids. Food Chem Toxicol. 2003;41:753–758.
- Prior RL, Wu XL. Anthocyanins: structural characteristics that result in unique metabolic patterns and biological activities. Free Radic Res. 2006;10:1014–1028.
- Szajdek A, Borowska EJ. Bioactive compounds and health-promoting properties of berry fruits: a review. Plant Foods Hum Nutr. 2008;63:147–156.
- Arapitsas P, Perenzoni D, Nicolini G, et al. Study of Sangiovese wines pigment profile by UHPLC-MS/MS. J Agric Food Chem. 2012;60:10461–10471.
- Lätti AK, Kainulainen PS, Hayirlioglu-Ayaz S, et al. Characterization of anthocyanins in Caucasian blueberries (Vaccinium arctostaphylos L.) native to Turkey. J Agric Food Chem. 2009;57:5244–5249.
- He JJ, Liu YX, Pan QH, et al. Different anthocyanin profiles of the skin and the pulp of Yan73 (Muscat Hamburg × Alicante Bouschet) grape berries. Molecules. 2010;15:1141–1153.
- Liang Z, Wu B, Fan P, et al. Anthocyanin composition and content in grape berry skin in Vitis germplasm. Food Chem. 2008;111:837–844.
- Ortega-Regules A, Romero-Cascales I, López-Roca JM, et al. Anthocyanin fingerprint of grapes: environmental and genetic variations. J Sci Food Agric. 2006;86:1460–1467.
- Azuma A, Kobayashi S, Goto-Yamamoto N, et al. Color recovery in berries of grape (Vitis vinifera L.) ‘Benitaka’, a bud sport of ‘Italia’, is caused by a novel allele at the VvmybA1 locus. Plant Sci. 2009;176:470–478.
- Pérez-Trujillo JP, Hernández Z, López-Bellido FJ, et al. Characteristic phenolic composition of single-cultivar red wines of the Canary Islands (Spain). J Agric Food Chem. 2011;59:6150–6164.
- Andre TQW, Stephen CVI, Biao C, et al. Modulation of hepatic lipoprotein synthesis and secretion by taxifolin, a plant flavonoid. J Lipid Res. 2000;41:1969–1979.
- Cruz AAD, Hilbert G, Mengin V, et al. Anthocyanin phytochemical profiles and anti-oxidant activities of Vitis candicans and Vitis doaniana. Phytochem Anal. 2013;24:446–452.
- Cravero MC, Guidoni S, Schneider A, et al. Morphological and biochemical characterization of coloured berry-muscat grapevine cultivars. Vitis. 1994;33:75–80.
- Ismail A, Marjan Z, Foong C. Total antioxidant activity and phenolic content in selected vegetables. Food Chem. 2004;87:581–586.
- Meng JF, Fang YL, Qin MY, et al. Varietal differences among the phenolic profiles and antioxidant properties of four cultivars of spine grape (Vitis davidii Foex) in Chongyi County (China). Food Chem. 2012;134:2049–2056.
- Ryan JM, Revilla E. Anthocyanin composition of Cabernet Sauvignon and Tempranillo grapes at different stages of ripening. J Agric Food Chem. 2003;51:3372–3378.
- Yamane T, Jeong ST, Goto-Yamamoto N, et al. Effects of temperature on anthocyanin biosynthesis in grape berry skins. Am J Enol Viticult. 2006;57:54–59.
- Esteban MA, Villanueva MJ, Lissarrague JR. Effect of irrigation on changes in the anthocyanin composition of the skin of cv Tempranillo (Vitis vinifera L) grape berries during ripening. J Sci Food Agric. 2001;81:409–420.
- Haselgrove L, Botting D, Heeswijck R, et al. Canopy microclimate and berry composition the effect of bunch exposure on the phenolic composition of Vitis vinifera L cv. Shiraz grape berries. Aust J Grape Wine Res. 2000;6:141–149.
- Correia AC, Jordão AM. Antioxidant capacity, radical scavenger activity, lipid oxidation protection analysis and antimicrobial activity of red grape extracts from different varieties cultivated in Portugal. Nat Prod Res. 2015;5:438–440.
- Lutz M, Jorquera K, Cancino B, et al. Phenolics and antioxidant capacity of table grape (Vitis vinifera L.) cultivars grown in Chile. J Food Sci. 2011;76:C1088–C1093.
- Yvonne K, Konrad O, Volker B. Processing strawberries to different products alters contents of vitamin C, total phenolics, total anthocyanins, and antioxidant capacity. J Agric Food Chem. 2005;53:5640–5646.