Abstract
When starving, diploid Saccharomyces cerevisiae yeasts can enter into at least two stable non-dividing states – sporulation or quiescence – and thus survive unfavorable conditions for long periods of time. However, which latent state will be preferred depends on numerous conditions. Here, we showed that budding yeasts can trigger transition into one or the other dormant state depending on the carbon source utilized. When fermentable carbon source (glucose) is present in the growth medium, the diploid S. cerevisiae entered quiescence. On the other hand, when cells were grown in the presence of the energy-rich respiratory carbon source ethanol, yeasts preferably formed ascospores. In both latent states a steady redox balance is maintained. Altogether, these findings strongly suggest that survival strategies in yeasts S. cerevisiae and transition into distinct differentiation programs depend on the cellular metabolic status.
Introduction
Diploid Saccharomyces cerevisiae budding cells can express different differentiation states during nutrient limitation, having distinct biological functions. These various states take place in response to similar environmental conditions, triggering the same signal transduction pathways, and sharing many of the same specific regulators [Citation1]. Thus in response to external stress, growth inhibitory signals, or starvation of one or more nutrients, the yeast cells may leave the mitotic cycle and enter one or another specific state: i) sporulation with haploid spores; ii) switching to “pseudohyphal growth” forming elongated chains of cells; iii) entering a stable non-proliferative G0 state known as “quiescence” or “stationary phase”, where they age or undergo programmed cell death [Citation2–5]. One of the main features of the “dormant” stages is their ability to maintain viability in a state of a minimal life for long periods of time and to start again their mitotic growth in restored growth stimulating conditions [Citation6]. That is why different latent states require coordinated deactivation of the metabolic processes in the cell in response to growth inhibitory signals or the imminent depletion of a major nutrient, rather than just cessation of protein or RNA synthesis.
Up to now, the most studies on quiescent S. cerevisiae yeasts have been performed on stationary cultures when grown with a sole carbon source - glucose [Citation7]. In these experiments, a diauxic shift is observed: cells first utilize the glucose, and then the ethanol formed as a result of the alcoholic fermentation. Upon the complete exhaustion of the carbon source in the medium, they entered in the so-called G0 state [Citation4]. However, the information about the processes provoking the entrance into quiescent state (Q) due to the depletion of other nutrients such as nitrogen or phosphorus, for example, or after growth in the presence of a non-fermentable carbon source, is scarcer. There is no comparative evidence as to whether the mechanisms of entry into the G0 state in cells starving on a given substrate are analogous to those starving for other nutrients. Transcriptomic analysis indicates that during starvation, activation of a common set of stress-response genes is observed regardless of which substrate is limited [Citation8]. However, cells also exhibit nutritionally specific changes in their gene expression, depending on the type of the limiting substrate [Citation9]. Moreover, entry into the quiescent state due to carbon limitation in the stationary phase can easily be overcome by the addition of glucose [Citation10]. At the same time, in cells that have entered G0 cell cycle as a result of nitrogen starvation, the addition of glucose does not stimulate the renewal of growth [Citation11]. Based on this, it can be assumed that latent states are not equal, and there are differences not only between G0 cells and spores, but also between different G0 states. Allen et al. [Citation12] showed that 20 h after cellular inoculation in a liquid glucose-rich medium, two types of adult cells are formed, which differ in a number of properties and can be separated from the liquid culture. These cells are designated as “quiescent/G0 (Q) cells” and “non-quiescent (NQ) cells” [Citation12–14]. When grown on a solid medium, cells of haploid strains S. cerevisiae are differentiated into subpopulations of U cells (metabolically active, stress resistant with extended longevity) and L cells (more sensitive to stress, with reduced viability, and expressing genes encoding proteins involved in hydrolytic reactions) [Citation15]. Contrary, in the colonies of the diploid strains, subpopulations of sporulating (S) and non-sporulating (NS) cells are observed. Moreover, despite many studies over the last 15 years of cellular biology of spore formation, many important issues remain to be elucidated in all aspects of the process. Key regulatory points in the choice of S. cerevisiae between sporulation and quiescence in natural environment are under discussion. Additionally, the genome analysis of many wild yeasts isolated from natural environments showed that they are diploid rather than haploid or polyploid.
Thus, the questions of hоw the ambient factors influence the entrance of diploid S. cerevisiae strains into G0 state or in sporulation and what factors trigger transition into one or another latent state is under investigation.
Materials and methods
Strains and media
The wild diploid strain Saccharomyces cerevisiae NBIMCC 584 was obtained from the National Bank for Industrial Microorganisms (Sofia, Bulgaria). It was grown in standard YEPD medium (1% yeast extract, 2% bacto-peptone, 2% glucose), Yeast Nitrogen Base supplemented with 2% glucose (YNBG) and Yeast Nitrogen Base supplemented with 1% ethanol (YNBE) at 30 °C on a reciprocal shaker (204 rpm) for 168 h.
Dry weight and kinetic parameters measurement
Cell dry weight was determined gravimetrically after drying washed cells to constant weight at 105 °C. Specific growth rate (μ) and cell yield coefficient (Yx/s) were calculated according to Monod kinetics as described by Pirt [Citation16].
Spores staining
The presence of spores was visualized microscopically, by specific Schaefer-Fulton staining with malachite green and safranin [Citation17].
Quiescent cells isolation
The isolation of quiescent cells was performed according to the protocol described by Allen et al. [Citation12].
Cell-free extract preparation
Cell wall disruption of isolated G0 cells was carried out according to the procedure of Nedeva et al. [Citation18]. Obtained supernatants were used to further analyze the intracellular levels of reactive oxygen species (ROS) and nicotinamide adenine dinucleotide and nicotinamide adenine dinucleotide phosphate (NADH/NADPH).
Protein determination
Protein content was determined by the method of Lowry et al. [Citation19]. Bovine serum albumin (Sigma St. Louis, MO, USA) was used as a standard.
Analysis of carbon and nitrogen sources utilization
Glucose concentration was measured by the method of Miller [Citation20]. Ethanol was determined according to Pilone [Citation21]. Organic nitrogen was measured through total ninhydrin protein analysis [Citation22] and inorganic nitrogen concentration was determined according to Leonard [Citation23].
Cellular redox state evaluation
The redox state of isolated latent yeast cells was assessed through measurement of intracellular levels of accumulated ROS [Citation24] and formed NADH/NADPH [Citation25].
Data analysis
The data analysis was performed using Microsoft Office 365 Excel 2016 software. The spectrophotometric data represent the average ± standard deviation (SD) of three independent experiments.
Results and discussion
Influence of carbon source on growth and proliferation
To assess the role of carbon metabolism on the viability of the stationary cultures of diploid strains S. cerevisiae, cells were grown in three different nutrient media: two with fermentable carbon source glucose, YEPD and YNBG (); and one with non-fermentable carbon source, ethanol (YNBE) (). In order to follow the growth dynamics of the studied stationary yeast culture, prolonged cultivation was carried out for 168 h at 30 °C.
Figure 1. Growth dynamics and carbon/nitrogen source utilization by S. cerevisiae NBIMCC 584 during batch cultivation in different nutrient media: YEPD (A), Yeast Nitrogen Base supplemented with 2% glucose (YNBG) (B) and Yeast Nitrogen Base supplemented with 1% ethanol (YNBE) (C).
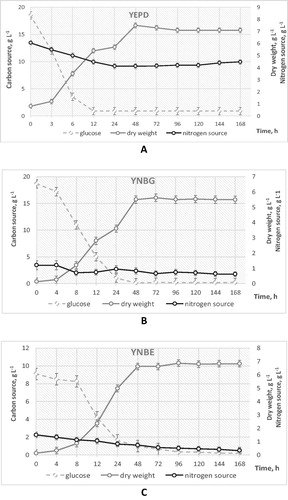
When yeast were grown on YNB supplemented either with glucose or ethanol, intensive cellular proliferation was observed up to the 48th hour (budding cells varied between 64.34 ± 2.21 and 87.36 ± 1.19%). As regards the YEPD medium, the carbon source (glucose) was readily depleted at the 24th hour, cultures entered the stationary phase earlier and cellular growth was slowed considerably (only 19.00 ± 1.20% budding yeasts at the 48th hour). Besides carbon exhaustion, when yeast cells were grown in YEPD medium, the concentration of the complex nitrogen source at the 168th hour was also diminished (), representing 50% of that initially added. At the same time the concentration of NH4SO4 in YNB medium was also reduced up to 14 – 20% (). Such type of nutritive ambient conditions are discussed also by Gray et al. [Citation4], who considered that during this phase, when the carbon source is utilized, cells rearranged their metabolism, gradually ceased their proliferation, differentiated into different populations, and some of them transited into stable, non-dividing state. In fact, all nutrient media used ensured reliable carbon limitation, where the nitrogen compound was in excess. This evidence gives opportunity to elucidate the role of the investigated glucose and ethanol during the latent stage of S. cerevisiae 584 diploid strain.
Further, in order to estimate the efficiency of the microbial growth, which is indicative of the cellular energy status, a monitoring of the kinetic growth parameters was performed. It was found that the specific growth rate measured during cultivation on the three studied media varied between 0.22 ± 0.02 and 0.34 ± 0.02 h−1, being the highest when cells were grown in the rich YEPD. The calculated Yx/s fluctuated between 0.30 ± 0.03 and 0.45 ± 0.01. The highest Yx/s values were measured in YNB medium with ethanol as carbon and energy source. The lowest yields were observed when cells were grown on YNBG (). The specific growth rate and the final biomass yield on YEPD medium were high and comparable to those previously reported [Citation26]. The obtained data showed that, before entering a dormant state, the studied diploid yeast populations were metabolically active with the highest energetic status determined for cells grown in YNBE, followed by YEPD and YNBG.
Table 1. Growth kinetic parameters for S. cerevisiae NBIMCC 584 strain grown in different nutrient media.
Influence of carbon source on entrance in latent state
A well-known fact is that, when starving, yeast cells can enter different latent states – form spores and/or transit into G0 phase [Citation1]. However, the question remains as to what the main factors responsible for a cell to choose one or the other state are. In order to examine the influence of the carbon (glucose and ethanol) sources on the yeast preferred differentiation, samples from each growth condition (YPD, YNBG and YNBE) were both analyzed every 24 h microscopically for the presence of spores and were centrifuged into a high-density percoll gradient to isolate G0 cells.
The results showed that when growing the diploid S. cerevisiae 584 strain in the presence of respiratory carbon source – YNBE medium – cells predominantly sporulate. As opposed to this, when grown on a glucose-containing medium – YEPD and YNBG – spore formation was not observed (). Here, cells preferred to enter into an alternative latent state, namely G0. Evaluation of the percentage of cells entering into the G0 phase of the cell cycle showed that they were the highest (65–68%) between the 144th and the 168th hour of cultivation. Quiescent cells in YNBE were not detected or their concentration was negligible (). The obtained results suggested that, depending on the carbon source used, one of two possible processes for entering a latent state is stimulated. According to Neiman [Citation2], several conditions must be met in order for the yeast cell to be able to undergo meiosis and form spores: carbon and/or nitrogen limitation, as well as mating type MAT A/α (diploid strain). Moreover, Honigberg [Citation1] showed that the S. cerevisiae sporulation program requires large energy input. It is clear that diploid S. cerevisiae cells have two possibilities. While growing on high energetic non-fermentable carbon source, such as ethanol, they can activate a differentiation program that includes meiosis and formation of more resistant ascospores. Here, the sporulation phenomenon observed is a response to the carbon limitation and is probably linked with the ability of yeast cells to maintain significant glycogen stores as they transit to oxidative metabolism [Citation27, Citation28]. Thus, the deprivation for carbon (ethanol) allows abundant energy production in the absence of cell division, and a sporulation program could be triggered. On the other hand, when S. cerevisiae diploid was grown in fermentable carbon source, spores were not formed. It this case, the depletion of glucose coupled with the Crabtree effect, acting in excess of nitrogen in the medium, probably could cause exit from the cell cycle into an alternative resting state – quiescence. Furthermore, the choice of one of the latent states was also related to the cellular redox homeostasis and when the metabolism is limited, ROS and hence oxidative damage are also predictive for the extension of the lifespan [Citation29–31].
Role of the cellular redox status on latent states
While aging, yeast cells undergo various metabolic changes, as a result of which high levels of ROS are accumulated. Consequently, various regulatory mechanisms are activated to support the preservation of intracellular redox homeostasis. It is also believed that in the cells of stationary yeast populations, different types of ROS are formed, and only some of them have a direct role in the transition to the resting state [Citation30]. Therefore, studying the changes in the intracellular concentrations of ROS and NADPH/NADH, as key factors responsible for the redox balance in the yeast cell, is important for establishing such fundamental dependencies as: 1) why do yeast cells pass from a reduced into oxidized state in the different life cycle stages; 2) what is the role of cellular metabolism in maintaining redox homeostasis in the different phases of cell growth and/or 3) how do changes in the redox cellular homeostasis affect the processes associated with entering stable non-dividing states when the nutrients are exhausted from the medium?
In this context, the intracellular concentrations of ROS and NADH/NADPH were investigated in yeast stationary populations isolated at the 144th and 168th hours of cultivation (late stationary phase when the number of cells entering quiescence/sporulating was the highest). The obtained data followed the same pattern regardless of the nutrient medium used (YEPD, YNBG or YNBE) (). At the 144th hour, an imbalance between reactive oxygen species and reduced equivalents was observed. The concentration of ROS was twice higher. The cell status was oxidized. This probably is related to the preparatory processes in cells for entering a stable latent state (spores or G0, depending on the growth conditions). At the 168th hour, a balance was achieved between ROS and NADH/NADPH, their concentrations were comparable and redox homeostasis was reached. Yeast cells had already entered a “sleeping” state. The data obtained were consistent with studies performed by De Virgilio [Citation7], who suggested the presence of specific protection mechanisms in quiescent cells, maintaining the redox balance and limiting intracellular damage.
Conclusions
Although existing knowledge is incomplete and limited, data clearly show that yeast cells can transit through different development stages and the choice of cell fate is probably determined by relatively small differences in nutrient environment. Yeast spores are formed under the specific condition of active respiration and depleted carbon and/or other nutrients. Entrance into quiescence on the other hand, is activated in the absence of at least one essential nutrient and growth on fermentable carbon source. However, the particular developmental program pursued depends on the “history” of cultivation including the growth medium composition, occurrence of nutrient limitations, availability of oxygen and energy. Thus the cellular development can be influenced by various signals that function as quorum-sensing molecules. How do signal molecules on the one hand and nutrients on the other affect the survival, persistence and life expectancy of stationary cells? This is an important issue which is related to the elucidation of the impact of nutrient limitation and starvation on cellular functions and the longevity of yeast. Taking into account all this information, it becomes clear that future research should clarify how the presence/absence of certain nutrients affects gene expression and metabolism, and leads to the unlocking of various differentiation programs. This will throw an additional light both on the mechanisms responsible for the formation of different yeast latent states, and on the signal pathways that trigger such differentiation.
Additional information
Funding
References
- Honigberg SM. Similar environments but diverse fates: Responses of budding yeast to nutrient deprivation. Microbial Cell. 2016;3(8):302–328.
- Neiman AM. Sporulation in the Budding Yeast Saccharomyces cerevisiae. Genetics 2011;189(3):737–765.
- Dickinson JR. Filament formation in Saccharomyces cerevisiae–a review. Folia Microbiol. 2008;53(1):3–14.
- Gray JV, Petsko GA, Johnston GC, et al. Sleeping beauty’: Quiescence in Saccharomyces cerevisiae. Microbiol Mol Biol Rev. 2004;68(2):187–206.
- Sagot I, Laporte D. The cell biology of quiescent yeast – a diversity of individual scenarios. J Cell Sci. 2019;132:1–10.
- Coller HA, Sang L, Roberts JM. A new description of cellular quiescence. PLoS Biol. 2006;4(3):e83. [cited 2019 Jun 12];
- De Virgilio C. The essence of yeast quiescence. FEMS Microbiol Rev. 2012;36(2):306–339.
- Galdieri L, Mehrotra S, Yu S, et al. Transcriptional regulation in yeast during diauxic shift and stationary phase. OMICS 2010;14(6):629–638.
- Gasch AP, Spellman PT, Kao CM, et al. Genomic expression programs in the response of yeast cells to environmental changes. Mol Biol Cell 2000;11(12):4241–4257.
- Granot D, Snyder M. Glucose induces cAMP-independent growth-related changes in stationary-phase cells of Saccharomyces cerevisiae. Proc Natl Acad Sci. 1991;88(13):5724–5728.
- Klosinska MM, Crutchfield CA, Bradley PH, et al. Yeast cells can access distinct quiescent states. Genes & Devel. 2011;25:336–349.
- Allen C, Büttner S, Aragon AD, et al. Isolation of quiescent and nonquiescent cells from yeast stationary-phase cultures. J Cell Biol. 2006;174(1):89–100.
- Wloch-Salamon DM, Tomala K, Aggeli D, et al. Adaptive roles of SSY1 and SIR3 during cycles of growth and starvation in Saccharomyces cerevisiae populations enriched for quiescent or nonquiescent cells. G3 Genes/Genomes/Genetics 2017;7:1899–1911.
- Leonov A, Feldman R, Piano A, et al. Caloric restriction extends yeast chronological lifespan via a mechanism linking cellular aging to cell cycle regulation, maintenance of a quiescent state, entry into a non-quiescent state and survival in the non-quiescent state. Oncotarget 2017;8(41):69328–69350.
- Palkova Z, Devaux F, R˘ic˘icová M, et al. Ammonia pulses and metabolic oscillations guide yeast colony development. Mol Biol Cell. 2002;13:3901–3914.
- Pirt SH. Principles of microbe and cell cultivation. Oxford: Blackwell Scientific Publications; 1975.
- Schaeffer AB, Fulton M. A simplified method of staining endospores. Sci. 1933;77(1990):194.
- Nedeva TS, Petrova VY, Hristozova T, et al. A modified procedure for isolation of yeast mitochondrial DNA. Z Naturforsch. 2002;57c:960–961.
- Lowry OH, Rosebrough NJ, Farr AL, et al. Protein measurement with the Folin phenol reagent. J Biol Chem. 1951;193(1):265–275.
- Miller GL. Use of dinitrosalicylic acid reagent for determination of reducing sugar. Anal Chem. 1959;31(3):426–428.
- Pilone GJ. Determination of ethanol in wine by titrimetric and spectrophotometric dichromate methods: collaborative study. J Assoc Off Anal Chem. 1985;68:188–190.
- Conklin-Brittain NL, Dierenfeld ES, Wrangham RW, et al. Chemical protein analysis: A comparison of Kjeldahl crude protein and total ninhydrin protein from wild, tropical vegetation. J Chem Ecol. 1999;25(12):2601–2622.
- Leonard RH. Quantitative range of Nessler's reaction with ammonia. Clin Chem. 1963;9:417–422.
- Choi HS, Kim JW, Cha Y-N, et al. A quantitative nitroblue tetrazolium assay for determining intracellular superoxide anion production in phagocytic cells. Immunoassay Immunochem. 2006;27:31–44.
- Swamy PM. UV-visible, fluorescence in IR spectroscopy: Absorption spectra In: Laboratory manual in biotechnology, 1st ed. New Delhi, India: Rakesh Kumar Rastogi for Rastogi Publications; 2008. p. 145–147.
- Koleva D, Petrova V, Kujumdzieva A. Comparison of enzymatic antioxidant defense system in different metabolic types of yeasts. Can J Microbiol. 2008;54(11):957–963.
- Wilson WA, Roach PJ, Montero M, et al. Regulation of glycogen metabolism in yeast and bacteria. FEMS Microbiol Rev. 2010;34(6):952–985.
- Cao L, Tang Y, Quan Z, et al. Chronological lifespan in yeast is dependent on the accumulation of storage carbohydrates mediated by Yak1, Mck1 and Rim15 kinases. PLoS Genet. 2016;12(12):e1006458–25.
- Laporte D, Lebaudy A, Sahin A, et al. Metabolic status rather than cell cycle signals control quiescence entry and exit. J Cell Biol. 2011;192(6):949–957.
- Ayer A, Campbell WG, Dawes IW. Cellular redox homeostasis, reactive oxygen species and replicative ageing in Saccharomyces cerevisiae. FEMS Yeast Res. 2014;14(1):60–72.
- Zhang N, Cao L. Starvation signals in yeast are integrated to coordinate metabolic reprogramming and stress response to ensure longevity. Curr Genet. 2017;63(5):839–843.