Abstract
Elevated CO2 can stimulate plant growth in greenhouses, but the molecular mechanism behind this response is unclear, especially in eggplants. In this study, we evaluated the physiological and photosynthetic response of the eggplant variety ‘Shenyuan 3’ under elevated CO2, and the candidate genes related to this response were identified by transcriptomic analysis. The results showed that elevated CO2 can promote eggplant growth and development in greenhouses; plant height and stem diameter were maximally increased by 28.62% and 5.20%, respectively. Net photosynthetic rate, light saturation point and intercellular CO2 concentration of eggplant leaves increased by 86.11%, 49.94% and 102.06%, respectively, whereas light compensation point, dark breathing rate and stomatal conductance decreased by 35.90%, 27.30% and 26.03%, respectively. A total of 169 differentially expressed genes (DEGs) were screened, of which 99 were up-regulated and 70 were down-regulated. Gene Ontology (GO) function enrichment analysis suggested that these proteins can be classified into 11 molecular functions, including transcription factors, phosphoenolpyruvate carboxylase and oxidoreductase. Pathway analysis indicated that elevated CO2 affects plant growth and development by affecting carbon metabolism, carbon fixation, chlorophyll and porphyrin metabolism. Twelve genes were further detected by qRT-PCR, and 11 genes showed similar dynamics with the transcriptomic data. Bilirubin dehydrogenase Sme2.5_04464.1_g00001 and the malate dehydrogenase gene Sme2.5_03383.1_g00002, which are involved in the chlorophyll porphyrin pathway and carbon metabolism, respectively, were up-regulated. Transcription factors bHLH (Sme2.5_01232.1_g00025) and GATA (Sme2.5_00372.1_g00006) were also up-regulated under elevated CO2 treatment. Further research should focus on cloning these genes and identifying their response mechanism under elevated CO2.
Introduction
As the raw material of photosynthesis, CO2 is essential for plant growth and development [Citation1]. The rates of plant growth and development can be stimulated by elevated CO2 [Citation2,Citation3]. The shoot and root dry weight of wheat varieties were increased by an average of 17% and 36%, respectively, at high CO2 concentration [Citation4]. Both the stem elongation and leaf area index were also increased by 21%, which resulted in a 26% increase in grain yield [Citation5]. The length, leaf area and dry weight of yams were also significantly increased in elevated CO2 [Citation6]. In two red leaf lettuce cultivars, a higher CO2 level (1000 μmol·mol−1) not only increased head mass and yields but also stimulated the accumulation of most flavonoid glycosides and sugar [Citation7]. Elevated CO2 environments with 800 ± 50 μmol·mol−1 in the daytime (T1, 7:00–19:00) or morning (T2, 7:00–12:00) increased the soluble sugar, starch, and chlorophyll contents, as well as the number and size of flowers in Gerbera jamesonii [Citation8]. Overall, elevated CO2 has a certain impact on plant growth, development and yield.
Elevated CO2 may facilitate photosynthesis by increasing plant photosynthetic rate and photosynthetic yield. For C3 plants, short exposure to high CO2 concentration could increase the net photosynthetic rate by 10%–50% [Citation9]. At a moderately increased CO2 concentration of 500–600 μmol·mol−1, the net photosynthetic rate was increased by more than 1.8 times in tomato seedlings [Citation10]. Under elevated CO2, the photosynthesis rate and water use efficiency of two mangrove species increased by 30% and 70%, respectively, but the transpiration rate decreased by more than 8% [Citation11]. The photosynthesis of Isatis indigotica Fortune increased under elevated CO2; as a result, the biomass increased by 36.8% [Citation12]. Conversely, high CO2 concentration may have a negative effect on stomatal conductance, which decreased by 17% in a previous study and might lead to an enhancement of the photosynthetic rate [Citation13]. In another study, the stomatal conductance of two sugarcane varieties reduced by 40% and 30%, respectively [Citation14]. The stomatal conductance of European beech seedlings grown under elevated CO2 concentration (770/950 μmol·mol−1) was significantly lower than that of seedlings grown in ambient air [Citation15]. Seedlings of four deciduous broad-leaved tree species showed down-regulation of photosynthesis, and the trees with different succession traits showed different stomatal and non-stomatal responses to elevated CO2 concentration [Citation16]. In short, elevated CO2 can facilitate photosynthesis but stomatal conductance is affected based on the different plants and varieties.
In a closed environment such as a greenhouse, the dynamic of CO2 concentration typically shows an irregular ‘U’ or ‘W’ curve. Especially in winter and spring in North China, a CO2 deficit often occurs in the morning after sunrise, which restricts plant growth and development [Citation17]. Therefore, supplying elevated CO2 could provide raw materials for plant photosynthesis, which would promote plant growth and increase biomass in the greenhouse [Citation18]. Eggplant (Solanum melongena L.) has been cultivated worldwide. China has a long growing history with this species and the acreage of eggplant cultivated in greenhouses has risen very quickly, but the molecular mechanism of eggplant growth and photosynthesis with elevated CO2 is still unclear.
In this study, we evaluated the effects of elevated CO2 (800 ± 50 μmol mol−1) on the growth and photosynthesis of the eggplant variety ‘Shenyuan 3’ in a greenhouse. Transcriptome sequencing technology was also employed to discover the molecular mechanism of eggplant response to elevated CO2. These results will provide a theoretical basis for eggplant cultivation in greenhouses.
Materials and methods
Plant material and experimental treatments
The experiment was conducted from 2016 to 2017 in a greenhouse located at the College of Horticulture in Shanxi Agricultural University. The tested seeding of the eggplant variety ‘Shenyuan 3’ with 6 true leaves planted in 5*10 cell trays was provided by Xinjiang Meixia Seedling Company, and planting with wider spacing of 30 cm × 60 cm in the soil of the greenhouse. The temperature of the greenhouse was kept at about 25–28 °C in the daytime and 15–18 °C at night. Water support and other activities were carried out using normal management practices.
The experiment included three independent growth areas of 20 m2 each, which were separated by a polyethylene film. The treatments for the growth areas included treatments 1 and 2 with an elevated CO2 concentration of 800 ± 50 μmol·mol−1, and a control (CK) with ambient CO2 (approximately 400 ppm). CO2 concentration was automatically monitored and recorded by a CO2 detector (GMM220, Vaisala, Vantaa, Finland) every 10 min [Citation19,Citation20]. Elevated CO2 was automatically controlled and transported into the treatment areas by a circulation exhauster from 8:00 to 10:00 a.m. on sunny days. The purity of the applied CO2 was 99.9%, which was supported by Jinqiao Gas Company in Taigu County.
Plant growth and photosynthesis determination
During the elevated CO2 treatment, stem height (from stem base to the first branch) and stem diameter were measured in the morning every 7 days. The photosynthetic characteristics of net photosynthetic rate (Pn), stomatal conductance (Gs), intercellular CO2 concentration (Ci), transpiration rate (Tr) and water use efficiency (WUE) were also detected by a LI-6400 photosynthesis system (LI-COR, Lincoln, NE, USA), of which the leaf cellule was 6 cm2, gas flow rate was set as 500 μmol s−1, and temperature was 25 °C. Blue and red light were used to take measurements at varying photosynthetically active radiation (PAR) intensities: 0, 50, 100, 150, 200, 400, 600, 800, 1000, 1200, 1400, 1600, 1800, 2000, 2200 μmol m−2 s−1. Then, the light response curve was fitted by the nonlinear regression function of SPSS, and we further deduced the apparent yield of CO2 fixation (AQY), light saturated photosynthetic rate (Pnmax), dark respiration rate (Rd), light compensation point (Lcp) and light saturation point (Lsp). Mature leaves were chosen for these analyses, which were conducted in triplicate.
Transcriptome analysis and DEGs identification
After four weeks of treatment, the healthy and mature leaves from five individuals that possessed a high photosynthetic rate were cut off, mixed and quickly frozen in liquid nitrogen, then stored at −80 °C for further experiments. Two biological replicates were prepared. Total RNA was isolated from yellow and orange petals using the TRIzol reagent (Invitrogen, Carlsbad, CA, USA) in accordance with the manufacturer’s instructions. RNA quality and purity were assessed using 1.0% agarose gels and a NanoDrop 8000 spectrophotometer (Thermo Scientific, Waltham, MA, USA), and RNA integrity was evaluated with an Agilent 2100 Bioanalyzer (Agilent Technologies, Santa Clara, CA, USA).cDNA libraries were constructed following the manufacturer’s instructions (Illumina, San Diego, CA, USA), and sequenced through the Illumina HiSeq high-throughput sequencing platform. The original reads obtained by sequencing were evaluated, and the reads sequence was compared with the eggplant reference gene sequence (ftp://ftp.kazusa.or.jp/pub/eeggplant/). After cleaning out the low quality reads, the gene function was described according to the following databases: GO (Gene Ontology), COG (Clusters of Orthologous Groups of proteins), Swiss-Prot (A manually annotated and reviewed protein sequence database), Kyoto Encyclopedia of Genes and Genomes (KEGG) and Nr annotation (NCBI non-redundant protein sequences). Subsequently, the FPKM method [Citation21] was used to normalize gene expression, and fold change ≥1.5 and FDR <0.05 were set for selection of the differentially expressed genes (DEGs) by DESeq [Citation22]. These DEGs were further mapped to the international Gene Ontology database (http://www.geneontology.org/) and the KEGG database, with the corrected p value ≤0.05 and q-value ≤0.05 defined as the parameter of significant enrichment, respectively.
Based on the selected reference genome sequence, the mapped reads were spliced using Cufflinks software and compared with the original genomic annotation information to find the unannotated transcribed region, and new transcripts and new genes in eggplant were discovered and supplemented.
Quantitative real-time polymerase chain reaction (qRT-PCR) analysis of DEGs of interest
By analyzing their GO function, pathway annotation and Nr annotation, we determined that 12 DEGs may be related to CO2 response; therefore, they were selected for expression verification by qRT-PCR. The 18s rRNA was used as the reference gene. Total RNA was extracted from eggplant leaf tissue exposed to CK and elevated CO2 levels using an RNA isolation kit (Tiangen Biotech Co., Ltd., Beijing, China). Then, qRT-PCR was performed using an ABI7500 Real Time PCR System (Applied Biosystems, Foster City, CA, USA) with SYBR® Premix Ex TaqTM II (Tli RNaseH Plus) (RR820A, Takara Bio, Kusatsu, Japan). The primer sequences are shown in . Amplification was achieved by the following PCR program: 94 °C for 5 min, then 40 cycles of denaturation at 95 °C for 30 s, and annealing and extension at 55 °C. Finally, the relative levels of target genes were calculated by the 2−ΔΔCt method [Citation23].
Table 1. Primers used for qRT-PCR analysis.
Results and discussion
Effect of elevated CO2 on the growth of eggplant
High CO2 level has a significant effect on the growth and development of plants [Citation24]. When the concentration of CO2 in the atmosphere is increased, most plants, such as wheat [Citation25], soybean [Citation26] and cotton [Citation27], grow faster, the growth cycle is shorter, the total dry matter increases, and the root–shoot ratio increases. Under high CO2 conditions, the number of leaves and biomass all increased, and the physiological adaptability was enhanced in Withania somnifera [Citation28]. In this study, the dynamics of eggplant height and stem diameter were studied continuously for 28 d. The results showed that the plant height increased quickly during the 4 weeks under elevated CO2 and showed the maximum difference quantity, about 24.08 cm compared to the control, at 21 d (). Although the stem diameter maximum increased by 5.20% after 7 d under elevated CO2 treatment, there was no distinctive difference between elevated and atmospheric CO2 concentration in these four weeks (). These results suggested that high CO2 concentration can promote eggplant growth; this may be due to the enhanced photosynthesis in leaves in the presence of elevated CO2, which increased the output [Citation29].
Figure 1. Effect of elevated CO2 on eggplant growth. Dynamics of plant height (A), stem diameter (B), plant width (C) and leaf numbers (D) of eggplant.
Note: Bars represent mean values ± SD (n = 3) of triplicate repeats. Upper case letters in each panel indicate significant differences among samples by Student’s t-test at p < 0.01 and lower case letters indicate significant differences at p < 0.05.
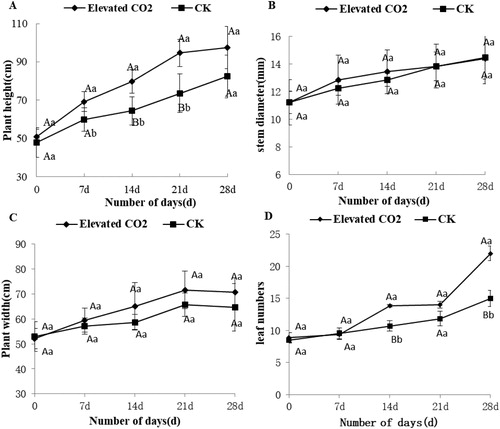
Effect of elevated CO2 on the photosynthesis in eggplant
Elevated CO2 can increase the net photosynthetic rate in most plants. The net photosynthetic rate of leaves increased with high CO2 concentration in soybean and oak species [Citation30,Citation31]. Increased intercellular CO2 concentration and decreased stomatal conductance were found in Sedum alfredii and mulberry under an elevated CO2 environment [Citation32,Citation33]. In a previous study, the total rate of photosynthesis in white birch seedlings increased by 69%, but the stomatal conductance of the leaves decreased [Citation34]. Here, the relative parameters of photosynthesis were also assessed (). Pn of eggplant leaves under elevated CO2 increased by 86.11% compared to that in the ambient CO2 concentration, indicating that the eggplant leaves under elevated CO2 could fix more organic carbon, which may promote eggplant growth. Lsp of eggplant plants increased by 49.94% and Lcp decreased by 35.90%, which suggested that the light adaptability of eggplant leaves was higher under elevated CO2. AQE of eggplant increased by 58.00%, which meant the light energy conversion efficiency, was higher under elevated CO2 than that under the natural CO2 concentration. Under elevated CO2, Rday reduced by 27.30%, which indicates that the lower physiological activity reduced breathing and indirectly increased the accumulation of dry matter in eggplant leaves. We also found that Gs of the leaves decreased by 26.03%, and Ci increased by 102.06% under the elevated CO2 environment, which meant that the increased net photosynthetic rate was not restricted by stomata but was due to the enhanced photosynthetic activity of mesophyll cells in eggplant leaves. Summarily, the application of exogenous CO2 in the greenhouse stimulated plant growth, mainly due to the increased net photosynthetic rate combined with high intercellular CO2 concentration and the decreased stomatal conductance in photosynthesis.
Table 2. Effect of elevated CO2 on photosynthesis in eggplant.
Transcriptome analysis of elevated CO2 on eggplant
As the growth and photosynthesis of eggplant were promoted by elevated CO2, the relative response genes were further investigated by transcriptome analysis. A total of 28.73 Gb of clean data from 4 samples were detected by RNA sequencing (RNA-seq), of which 81.44% to 82.54% of the reads were mapped to the annotated eggplant genome (Supplemental Table S1); the percentage of GC content was about 43.18%, and the Q30 base was more than 90.09% (Supplemental Table S2). Furthermore, a total of 1,461 new genes were discovered by comparing our results with the annotation information of the original eggplant genome and removing sections to encode short peptide chains (less than 50 amino acid residues) (Supplemental Table S3).
Using fold change ≥1.5 and FDR <0.05 as a threshold, a total of 169 unique DEGs were found between the elevated and natural CO2 environments, of which 99 were up-regulated and 70 were down-regulated under elevated CO2 (Supplemental Table S4). By GO annotation, these DEGs could be divided into 53 functional groups that include biological processes (20 terms), cellular components (16 terms), and molecular functions (17 terms). In these classifications, the terms ‘metabolic process’, ‘cell process’, ‘cell part’, ‘cell’, ‘binding’ and ‘catalytic activity’ annotated the most DEGs. In addition, the terms ‘single biological process’, ‘response to stimulus’ and ‘biological regulation’ applied to relatively more DEGs in the biological process category; the terms of ‘organelle’, ‘membrane’ and ‘organelle part’ were highly presented in the cellular component category; and the terms ‘transporter activity’ and ‘electronic carrier activity’ were annotated with more DEGs than other terms in the molecular function category. However, DEGs with the terms ‘locomotion’, ‘membrane-enclosed lumen’ and ‘nutrition reservoir activity’ were the least represented in the three main categories ().
Figure 2. Gene ontology(GO) analysis of differential expressed genes (DEGs) from eggplant. A total of 169 DEGs were categorized into 53 function items.
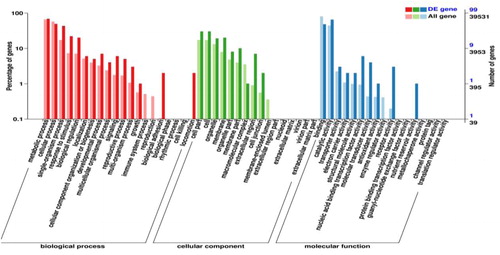
These genes were further annotated to 49 pathways in the KEGG database, including metabolism (66 DEGs), genetic information processing (8 DEGs), environmental information processing (8 DEGs) and cellular processes (1 DEGs) (). Seventeen DEGs were found to be involved in the plant hormone signal transduction pathway, which can regulate the whole plant development process. There were also 4 and 3 DEGs annotated to the pathways of carbon metabolism and carbon fixation in reference to photosynthetic organisms, respectively. All these annotations indicated that elevated CO2 has a significant impact on plant growth and photosynthesis.
Figure 3. KEGG analysis of differential expressed genes(DEGs). A total of 49 pathways were identified.
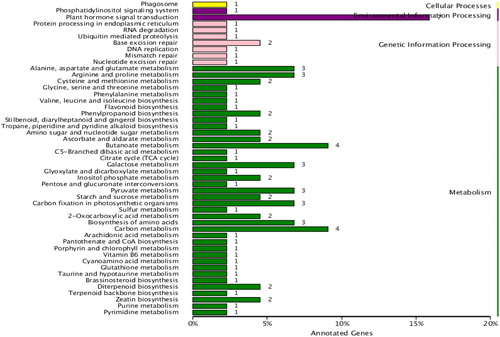
Using Nr annotations (Supplemental Table S4), the predicted proteins of DEGs were further classified into hormones, transcription factors, chloroplasts and carbon metabolism. Seventeen DEGs (13 up-regulated and 4 down-regulated) encoded hormone-related proteins, including the ethylene receptor gene (Sme2. 5_02344.1_g00010, Sme2.5_06157.1_g00004 and Sme2.5_00457.1_g00010), gibberellin β oxygenase gene (Sme2.5_00183.1_g00010, Sme2.5_00145.1_g00009 and Sme2.5_00145.1_g00010) and auxin binding protein gene (Sme2.5_00209.1_g00018). Nine DEGs (6 up-regulated and 3 down-regulated) encoded transcription factors including the transcription factor bHLH87 gene (Sme2.5_01232.1_g00025), GATA transcription factor (Sme2.5_00372.1_g00005) and ABC transporter C family member gene (Sme2.5_01812.1_g00005). Twelve DEGs (8 up-regulated and 4 down-regulated) encoded chloroplast pigments including cytochrome protein genes (Sme2.5_00037.1_g00011, Sme2.5_07385.1_g00001) and the porphobilinogen deaminase gene (Sme2.5_04464.1_g00001). Four DEGs (1 up-regulated and 3 down-regulated) belonged to the carbon metabolism class, including the phosphoenolpyruvate dehydrogenase gene (Sme2.5_13003.1_g00001, Sme2.5_00502.1_g00014) and malate dehydrogenase gene (Sme2.5_03383.1_g00002).
GO enrichment analysis of DEGs
By screening significant enrichment of GO terms with a corrected p value ≤0.05 as the standard, all DEGs were further divided into 13 terms of biological processes and 11 terms of molecular functions but without terms of cellular components. Among the biological processes (Supplemental Table S5), 11 DEGs were enriched in the oxidation–reduction process and 2 DEGs in the carbon fixation terms, which suggested that eggplant produced many stress responses in addition to photosynthesis under elevated CO2 environment. Among these molecular functions (Supplemental Table S6), ATP binding had the most unigenes (13 DEGs), followed by protein serine/threonine kinase activity (8 DEGs). Oxidoreductase activity (6 DEGs) was also involved in the response to the elevated CO2 environment. This analysis suggested that photosynthesis-related biological processes and enzymes in eggplant were affected by the elevated CO2 environment. Protein synthesis metabolism and energy metabolism were also involved in these reactions. All these reactions may stimulate the growth and photosynthesis of eggplant under an elevated CO2 environment.
Validation of DEGs by qRT-PCR
Twelve DEGs were selected to examine their different expression levels by qRT-PCR. These DEGs included genes related to carbon metabolism, such as Sme2.5_00502.1_g00014, Sme2.5_13003.1_g00001 and Sme2.5_03383.1_g00002; chlorophyll-hormone metabolism-related genes Sme2.5_04464.1_g00001 and Sme2.5_00037.1_g00011, starch and sucrose metabolism gene Sme2.5_00497.1_g00006 and ABA transporter gene Sme2.5_01812.1_g00005; hormone metabolism-related genes Sme2.5_00003.1_g00016, Sme2.5_00457.1_g00010 and Sme2.5_02344.1_g00010; and transcription factors Sme2.5_01232.1_g00025 and Sme2.5_00372.1_g00006. The results showed that the expression trends of these 11 genes were consistent with the RNA-seq results (), while Sme2.5_13003.1_g00001 showed obvious expression trends, which may suggest the complexity of carbon metabolism under elevated CO2 treatment.
Effect of elevated CO2 on photosynthesis metabolic pathways
Transcriptome analysis has been widely used to investigate and provide a fundamental insight into biological processes [Citation35]. Thousands of genes showed different expression under high CO2 concentration. In sea buckthorn varieties, these DEGs were involved in photosynthesis, the spermidine biosynthetic process and chitin metabolism. Some transcription factors, such as WRKY, MYB and NAC, were also involved in the response to high CO2 concentration [Citation36]. Under elevated CO2, the lignin level of celery petioles increased, and the transcript abundance of 12 genes involved in lignin metabolism were also changed under high CO2 concentration [Citation37]. Elevated CO2 had a more significant effect on hormone metabolism, cell division and expansion, and the Ras signaling pathway, which could increase the division and expansion of cell membranes and xylem cells, resulting in a significant accumulation in biomass in perennial poplar trees [Citation16]. The different expression of these genes also depended on the age of Populus leaves; most DEGs were up-regulated in young leaves, but most were down-regulated in early-ripening leaves [Citation38]. Under elevated CO2, there were 5 genes involved in photosynthesis metabolic pathways that were found to be expressed differently in eggplant leaves, including the carbon metabolism pathway, photosynthetic carbon fixation pathway, porphyrin and chlorophyll metabolism pathway. Subsequently, the genes related to transcription factors also showed different expressions.
Malate dehydrogenase (EC 1.1.1.37) is the most important enzyme in the C4 pathway of plant carbon metabolism. In the cytoplasm of plant mesophyll cells, phosphoenolpyruvate immobilizes HCO3− to form oxaloacetate under the catalysis of phosphoenolpyruvate carboxylase. Then, this oxaloacetate is catalyzed by malate dehydrogenase and reduced to malic acid. Malic acid is a key metabolite in plants, and can enter the vascular bundle and decompose to release CO2 and a molecule of pyruvic acid, after which CO2 enters the Calvin cycle to complete photosynthesis. Overexpression of the cytosolic malate dehydrogenase gene (MdcyMDH) promoted the growth of transgenic lines of apples, and significantly increased the root weight, total fresh weight and photosynthetic rate of transgenic lines [Citation39]. The bolting was advanced, and plant height and the number of rosette leaves were significantly increased in transgenic Arabidopsis and in Chinese cabbage with overexpressed BraMDH [Citation40]. In this study, the gene Sme2.5_03383.1_g00002 encoding malate dehydrogenase was up-regulated, which may increase photosynthesis and thus promote plant growth under elevated CO2.
Bilirubin deaminase (EC2.5.1.61), also known as porphobilinogen deaminase, participates in the synthesis of precursors of chlorophyll synthesis; porphobilinogen synthesizes hydroxymethyl cholanogen and then polymerizes to form uroporphyrinogen III. The mutation of camouflage1 (cf1) which encodes porphobilinogen deaminase shows the nonclonal, yellow-green sectors in its leaves [Citation41]. We found the gene Sme2.5_04464.1_g00001, which encodes bilirubin dehydrogenase, was up-regulated in eggplant, which may promote the synthesis of hydroxymethyl bilirubin and chlorophyll synthesis precursors, and therefore further promotes the synthesis of plant chlorophyll and facilitation of photosynthesis.
Transcription factors BHLH and GATA may be involved in the reaction to elevated CO2
The bHLH and GATA family proteins play a key role in plant responses to abiotic stress. The transcription factors of the bHLH family have been shown to play important roles not only in the response to abiotic stress but also in photoresponse [Citation42] and hormonal signals [Citation43]. Overexpression of bHLH30 caused an upward curl in Arabidopsis leaves [Citation44]. Overexpression of the MdbHLH104 gene in apples showed a high tolerance to iron deficiency [Citation6]. In this study, the expression of the bHLH gene Sme2.5_01232.1_g00025 was up-regulated under elevated CO2 treatment, which suggested that this gene may take part in the eggplant response to elevated CO2. However, this requires further research.
The GATA family also plays a role in the secondary metabolism of plants involved in the process of chlorophyll synthesis and regulation of carbon and nitrogen metabolism. The GATA members in Arabidopsis are widely involved in chlorophyll levels, chloroplast size, photosynthetic efficiency and regulation of carbon and nitrogen metabolism [Citation25,Citation45]. In a previous study, the expression of AmZFPG, which belongs to the GATA family, increased over continued drought treatment or low temperature treatment at 4 °C for 2 h [Citation46]. In this study, the expression level of the GATA gene Sme2.5_00372.1_g00006 was up-regulated and affected by high CO2 concentration. This may be related to increased photosynthesis in eggplant plants, which suggested that it may regulate some metabolic process in eggplant under elevated CO2 treatment.
Conclusions
In this study, we evaluated the response of the eggplant variety ‘Shenyuan 3′ under elevated CO2, and the candidate genes related to this response were selected by transcriptomic analysis combined with qRT-PCR. The results showed that plant height and stem diameter increased rapidly in the early stage, and the light saturation point and net photosynthetic rate of leaves increased, but the light compensation point decreased. Further transcriptome analysis suggested that the change in the expression levels of the genes which encode malate dehydrogenase and other related enzymes in plant carbon metabolism and chlorophyll metabolism may have resulted in increased eggplant growth and photosynthesis under elevated CO2. Two transcription factors, Sme2.5_01232.1_g00025 and Sme2.5_00372.1_g00006, were also involved in this response, and require further study.
Supplemental Material
Download MS Excel (239.5 KB)Disclosure statement
No potential conflict of interest was reported by the authors.
Data availability
The authors confirm that the data supporting the findings of this study are available within the article and its supplementary materials.
Additional information
Funding
References
- Kazemi S, Eshghizadeh HR, Zahedi M. Responses of four rice varieties to elevated CO2 and different salinity levels. Rice Sci. 2018;25(3):142–151.
- Xiao L, Liu GB, Sha X. Elevated CO2 concentration and drought stress exert opposite effects on plant biomass, nitrogen, and phosphorus allocation in bothriochloa ischaemum. J Plant Growth Reg. 2016;35(4):1–10.
- Meng GT, Li GX, He LP, et al. Combined effects of CO2 enrichment and drought stress on growth and energetic properties in the seedlings of a potential bioenergy crop Jatropha curcas. J Plant Growth Regul. 2013;32(3):542–550.
- Eshghizadeh HR, Zahedi M, Mohammadi S. Differential growth responses of wheat seedlings to elevated CO2. Not Sci Biol. 2018;10(3):400–409. [cited 2020 Jun 30]
- Verrillo F, Badeck F-W, Terzi V, et al. Elevated field atmospheric CO2 concentrations affect the characteristics of winter wheat (cv. Bologna) grains. Crop Pasture Sci. 2017;68(8):713. [cited 2020 Jun 30]
- Wang R, Zhao P, Kong N, et al. Genome-wide identification and characterization of the potato bHLH transcription factor family. Genes. 2018;9(1):54. [cited 2020 Jun 30]
- Becker C, Kläring H-P. CO2 enrichment can produce high red leaf lettuce yield while increasing most flavonoid glycoside and some caffeic acid derivative concentrations. Food Chem. 2016;199:736–745.
- Xu S, Zhu X, Li C, et al. Effects of CO2 enrichment on photosynthesis and growth in Gerbera jamesonii. Sci Hortic Amsterdam. 2014;177:77–84.
- Cohen I, Rapaport T, Berger RT, et al. The effects of elevated CO2 and nitrogen nutrition on root dynamics. Plant Sci. 2018;272:294–300.
- Thongbai P, Kozai T, Ohyama K. CO2 and air circulation effects on photosynthesis and transpiration of tomato seedlings. Sci Hortic. 2010;126(3):338–344.
- Jacotot A, Marchand C, Gensous S, et al. Effects of elevated atmospheric CO2 and increased tidal flooding on leaf gas-exchange parameters of two common mangrove species: Avicennia marina and Rhizophora stylosa. Photosyn Res. 2018;138(2):249–260.
- Li P, Li H, Zong Y, et al. Photosynthesis and metabolite responses of Isatis indigotica Fortune to elevated CO2 Crop J. 2017;5(4):345–353.
- Xu Z, Shimizu H, Yagasaki Y, et al. Interactive effects of elevated CO2, drought, and warming on plants. J Plant Growth Regul. 2013;32(4):692–707.
- Souza APD, Gaspar M, Silva EAD, et al. (2010. Elevated CO2 increases photosynthesis, biomass and productivity, and modifies gene expression in sugarcane. Plant Cell Environ. 2008;31(8):1116–1127.
- Lotfiomran N, Kohl M, Fromm J. Interaction effect between elevated CO2 and fertilization on biomass, gas exchange and C/N ratio of European beech (Fagus sylvatica L.). Plants (Basel). 2016;5(3):38. [cited 2020 Jun 30]
- Wei H, Gou J, Yordanov Y, et al. Global transcriptomic profiling of aspen trees under elevated [CO2] to identify potential molecular mechanisms responsible for enhanced radial growth. J Plant Res. 2013;126(2):305–320.
- Jiang YQ, Li T, Zhang M, et al. WSN-based control system of CO2 concentration in greenhouse. Intell Autom Soft Co. 2015;21(3):285–294.
- Sun J, Gibson KM, Kiirats O, et al. Interactions of nitrate and CO2 enrichment on growth, carbohydrates, and rubisco in Arabidopsis starch mutants. Significance of starch and hexose. Plant Physiol. 2002;130(3):1573–1583.
- Zheng S, Chen Z, Nie H, et al. Identification of differentially expressed photosynthesis- and sugar synthesis-related genes in tomato (Solanum lycopersicum) plants grown under different CO2 concentrations. Biotechnol Biotechnol Equip. 2020;34(1):84–92. [cited 2020 Jun 30]
- Song H, Li Y, Xu X, et al. Analysis of genes related to chlorophyll metabolism under elevated CO2 in cucumber (Cucumis sativus L.). Sci Hortic. 2020;261:108988. [cited 2020 Jun 30]
- Florea L, Song L, Salzberg SL. Thousands of exon skipping events differentiate among splicing patterns in sixteen human tissues. F1000Res. 2013;2(188):188–188.
- Anders S, Huber W. Differential expression analysis for sequence count data. Genome Biol. 2010;11(10):R106–2011.
- Yuan Q, Song C, Gao L, et al. Transcriptome de novo assembly and analysis of differentially expressed genes related to cytoplasmic male sterility in onion. Plant Physiol Biochem. 2018;125:35–44.
- Becklin KM, Walker SM, 2nd, Way DA, et al. CO2 studies remain key to understanding a future world. New Phytol. 2017;214(1):34–40.
- Yi-Hsuan C, Zubo YO, Wiebke T, et al. Functional characterization of the GATA transcription factors GNC and CGA1 reveals their key role in chloroplast development, growth, and division in Arabidopsis. Plant Physiol. 2012;160(1):332–348.
- Ainsworth EA, Davey PA, Bernacchi CJ, et al. A meta‐analysis of elevated CO2 effects on soybean (Glycine max) physiology, growth and yield. Global Change Biol. 2002;8(8):695–709.
- Zhang S, Fu W, Zhang Z, et al. Effects of elevated CO2 concentration and temperature on some physiological characteristics of cotton (Gossypium hirsutum L.) leaves. Environ Exp Bot. 2017;133:108–117.
- Sharma R, Singh H, Kaushik M, et al. Adaptive physiological response, carbon partitioning, and biomass production of Withania somnifera (L.) Dunal grown under elevated CO2 regimes. 3 Biotech. 2018;8(6):267. [cited 2020 Jun 30]
- Wang Y‐P, Rey A, Jarvis PG. Carbon balance of young birch trees grown in ambient and elevated atmospheric CO2 concentrations. Global Change Biol. 1998;4(8):797–807.
- Killi D, Bussotti F, Gottardini E, et al. Photosynthetic and morphological responses of oak species to temperature and CO2 increased to levels predicted for 2050. Urban Urban Gree. 2018;31:26–37.
- Li Z, Zhang Y, Yu D, et al. The influence of precipitation regimes and elevated CO2 on photosynthesis and biomass accumulation and partitioning in seedlings of the rhizomatous perennial grass Leymus chinensis. PloS One. 2014;9(8):e103633. [cited 2020 Jun 30]
- Wu K, Li J, Luo J, et al. Effects of elevated CO2 and endophytic bacterium on photosynthetic characteristics and cadmium accumulation in Sedum alfredii. Sci. Total Environ. 2018;643:357–366.
- Madhana Sekhar K, Rachapudi VS, Mudalkar S, et al. Persistent stimulation of photosynthesis in short rotation coppice mulberry under elevated CO2 atmosphere. J Photochem Photobiol B Biol. 2014;137:21–30.
- Cao B, Dang QL, Zhang S. Relationship between photosynthesis and leaf nitrogen concentration in ambient and elevated CO2 in white birch seedlings. Tree Physiol. 2007;27(6):891–899.
- Li w, Liu y, Zeng s, et al. gene expression profiling of development and anthocyanin accumulation in kiwifruit (Actinidia chinensis) based on transcriptome sequencing. PLoS One. 2015;10(8):e0136439. [cited 2020 Jun 30]
- Zhang G, Zhang T, Liu J, et al. Comprehensive analysis of differentially expressed genes reveals the molecular response to elevated CO2 levels in two sea buckthorn cultivars. Gene. 2018;660:120–127.
- Liu JX, Feng K, Wang GL, et al. Elevated CO2 induces alteration in lignin accumulation in celery (Apium graveolens L.). Plant Physiol Biochem. 2018;127:310–319.
- Taylor G, Street NR, Tricker PJ, et al. The transcriptome of Populus in elevated CO2. New Phytol. 2005;167(1):143–154.
- Yao YX, Li M, Zhai H, et al. Isolation and characterization of an apple cytosolic malate dehydrogenase gene reveal its function in malate synthesis. J Plant Physiol. 2011;168(5):474–480.
- Li QF, Zhao J, Zhang J, et al. Ectopic expression of the chinese cabbage malate dehydrogenase gene promotes growth and aluminum resistance in Arabidopsis. Front Plant Sci. 2016;7:1180. [cited 2020 Jun 30]
- Huang M, Slewinski TL, Baker RF, et al. Camouflage patterning in maize leaves results from a defect in porphobilinogen deaminase. Mol Plant. 2009;2(4):773–789.
- Huq E, Quail PH. PIF4, a phytochrome-interacting bHLH factor, functions as a negative regulator of phytochrome B signaling in Arabidopsis. EMBO J. 2002;21(10):2441–2450.
- Sumin L, Seunghee L, Ki-Young Y. Overexpression of PRE1 and its homologous genes activates Gibberellin-dependent responses in Arabidopsis thaliana. Plant Cell Physiol. 2006;47(5):591. [cited 2020 Jun 30]
- An R, Liu X, Wang R, et al. The over-expression of two transcription factors, ABS5/bHLH30 and ABS7/MYB101, leads to upwardly curly leaves. PloS One. 2014;9(9):e107637.[cited 2020 Jun 30]
- Bi YM, Zhang Y, Signorelli T, et al. Genetic analysis of Arabidopsis GATA transcription factor gene family reveals a nitrate-inducible member important for chlorophyll synthesis and glucose sensitivity. Plant J. 2005;44(4):680–692.
- Zhi GH, Shi JN, Zhao XX, et al. overexpression of a zinc-finger protein gene AmZFPG from Ammopiptanthus mongolicus confers tolerance to cold, drought and salt stress in transgenic tobacco. Acta Hort Sinica. 2013;40(4):713–723. (in Chinese)