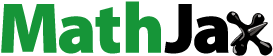
Abstract
Synthesis of ethyl acetate (EA) using lipase as a catalyst, as an environmentally-friendly process, has attracted considerable attention. However, the slow rate of enzymatic reaction and higher cost limit its applications. Ultrasound irradiation (UI) is known as an effective tool for accelerating the rate of enzymatic reaction under mild conditions. To attain maximum yield, it is crucial to understand the effect of UI on EA synthesis and the underlying mechanism. Therefore, the present study investigated the effect of UI on lipase-catalyzed ethyl acetate synthesis. The results clearly demonstrated that UI could remarkably accelerate the process of lipase-catalyzed synthesis of ethyl acetate compared with mechanical shaking. The optimal conditions of ultrasound-assisted reaction by using Novozyme 435® were 6% enzyme loading, 4:1 molar ratio of ethanol to acetic acid, 150 W & 28 kHz ultrasonic power and frequency, respectively. Bi-bi ping-pong model was employed to give a deep insight into the underlying mechanism of the beneficial effect of UI. It was found that Vmax under UI was elevated by 143% compared with that under mechanical shaking. Application of UI could decrease Kacetic acid and Ki ethanol while increase Kethanol and Ki acetic acid. In conclusion, the present study addresses that UI could remarkably accelerate the process of lipase-catalyzed synthesis of EA compared with mechanical shaking, and kinetic model results explained why ultrasound can significantly speed up lipase catalyzed synthesis of ethyl acetate, which may help to improve the industrial application potential of ethyl acetate biosynthesis.
Introduction
Ethyl acetate (EA) has been used as a versatile solvent for countless applications [Citation1–3]. Specifically, EA can be utilized as a food additive due to its pear-like and banana-like aromas in food industry [Citation4–6]. The current industrial production of EA involves esterification of acetic acid with ethanol using strong acids as catalysts, dehydrogenation of ethanol and so on, which are typically energy intensive and environmentally-unfriendly processes [Citation7]. In recent years, increased awareness of environmental sustainability has directed the focus on biotechnological production of chemicals. Synthesis of esters using lipase as a catalyst has attracted considerable attention [Citation8,Citation9]. The advantages of using lipase as a catalyst include mild reaction conditions, high substrate specificity and enantioselectivity [Citation10]. However, the relatively low reaction rate of the enzymatic reaction limits the potential application in industry [Citation11].
Ultrasound has been considered as one of the feasible ways to accelerate the process of enzymatic reaction [Citation12]. Accumulative evidence has suggested that the rate of the lipase-catalyzed reaction in the presence of ultrasound irradiation (UI) is significantly increased compared to that in the absence of UI [Citation13,Citation14]. The beneficial effect of ultrasound on the enzymatic reaction is known as cavitational effect, which involves the following two steps: first, violent mechanical vibration of the medium caused by compression and rarefaction cycle of ultrasonic waves generates bubbles or cavities, which subsequently develop and implode [Citation15]. It was reported that the intensity and frequency of UI have a great impact on the formation, development and implosion of bubbles [Citation16]. Second, elevated temperature and pressure generated by the collapse of bubbles intensify the mass transfer and homogeneity of substrates to enzyme in the microenvironment, resulting in an increase in the rate and conversion of reaction [Citation17]. It is reported that this phenomenon is largely dependent on the molar ratio of the substrates and enzyme loading [Citation18,Citation19]. Although the effects of ultrasonic treatment on the esterification of several esters have been evaluated, the ultrasound-assisted lipase-catalyzed EA has been scarcely explored.
The kinetic characteristics of enzyme reactions provide insights into the underlying mechanism of enzyme–substrate interactions and inhibitory effects of substrates, which can further help to find the most effective strategy to improve the conversion [Citation20,Citation21]. Interestingly, the kinetic parameters under UI were significantly altered compared with those under mechanical shaking. A previous study has shown that the Vmax and km values of esterification of D-isoascorbyl palmitate under ultrasound was pronouncedly elevated by 2.85 and 1.50 times, respectively, compared with those under mechanical shaking [Citation22]. The Bi-bi ping-pong model is extensively used in estimating parameters of lipase-catalyzed reaction kinetics since it was built for the reaction with two or more substrates and products [Citation20]. A recent study found that ultrasonic assistance significantly altered the kinetic parameters of enzymatic synthesis of isobutyl propionate by using the Bi-bi ping-pong model [Citation23]. However, it still remains unclear how ultrasonic treatment affect the kinetics of EA synthesis.
The present study was therefore conducted to investigate the beneficial effects of UI on lipase-catalyzed synthesis of EA. The effects of power and frequency of UI, enzyme loading as well as the molar ratio of the substrates on the conversion and rate of reaction were studied. The changes in the reaction kinetics in the presence or absence of ultrasound were also explored.
Materials and methods
Materials
Novozyme 435® (lipase B from Candida antarctica, immobilized on microporous polyacrylic resin beads), Lipozyme TL IM® (lipase from Thermomyces lanuginose) and Lipozyme RM IM® (lipase from Rhizomucor miehei) were supplied by Novozymes (Novo Nordisk, Denmark). Acetic acid, ethanol, n-hexane and acetonitrile were purchased from MREDA (Meridian Medical Technologies, USA). Ethyl acetate (>99%) was obtained from GmbH (Dr. Ehrenstorfer GmbH, Germany).
Ultrasound equipment
The experiments were conducted in an ultrasonic instrument DTD5200S (Beijing Hongxianglong Biotechnology Co., Ltd., Beijing, China) equipped with a thermostatic water bath, a microtip probe (diameter of 10 mm) and an ultrasound generator. The power of the ultrasonic equipment could be adjusted ranging from 0 to 300 W, and the frequency could be set at 28, 40, 50, 135 kHz.
Esterification reaction
Reactions were conducted by mixing ethanol, acetic acid, n-hexane, deionized water and immobilized lipase in a round-bottom flask. The effect of ultrasound was investigated by placing the flask in ultrasound equipment at certain power and frequency (). The aliquot fractions were periodically withdrawn and injected into an Agilent 1260 series high performance liquid chromatography (HPLC) system equipped with a ZORBAX Eclipse Plus C18 column (4.6 mm × 250mm, 5 µm particle size; Agilent, USA) and a UV–visible detector. The conversion of EA was analyzed with an isocratic elution of acetonitrile:water = 60:40 at 1 mL/min and identified at 210 nm. All the analyses were performed in triplicate and the data were expressed as mean values with standard deviations (±SD).
Kinetics of esterification reaction
To determine the kinetic constants of the reaction, the initial rate of reaction was estimated from the time course of EA concentration by calculating the initial slope of the tangent to the curve. The kinetic mechanism of the enzyme-catalyzed esterification of EA was considered based on the Bi-bi ping-pong mechanism as depicted in EquationEquation (1)(1)
(1) :
(1)
(1)
where and
are the corresponding Michaelis–Menten parameters, respectively.
EquationEquation (1)(1)
(1) represents the formation of EA by esterification of acetic acid and ethanol. Acetic acid firstly binds to the lipase giving out acyl-lipase and water. Then ethanol binds to acyl-lipase, producing ethyl acetate and releasing free enzyme. Ethanol can competitively bind to lipase to form a dead-end product lipase·ethanol, while acetic acid can bind to acyl-lipase to form a dead-end product acyl-lipase·acetic acid. These mechanisms are depicted in EquationEquations (2)
(2)
(2) and Equation(3)
(3)
(3) .
(2)
(2)
(3)
(3)
where and
were the corresponding Michaelis–Menten inhibition parameters, respectively.
The initial rate equation is given as follows:
(4)
(4)
where is the initial rate of reaction,
is the maximum rate of reaction, CA and CE are the concentrations of acetic acid and ethanol, respectively. The fitting of the experimental kinetic data was carried out by means of the non-linear multiparametric EquationEquation (4)
(4)
(4) .
Data analysis
All the experiments were conducted in triplicate. Data are representative images or mean values with standard deviation (±SD). Statistical analysis was done using the Wilcoxon texts with SPSS software 19.0 version (SPSS Inc., Chicago, IL, USA). Differences were considered statistically significant at p < 0.05.
Results and discussion
Effect of ultrasonic treatment on the lipase-catalyzed synthesis of EA
To examine the effect of ultrasonic treatment, EA was synthesized in the lipase-catalyzed reaction in the presence or absence of UI. As shown in , although the final conversion of EA in the absence or presence of UI were similar, this yield was achieved in 100 min under ultrasonic treatment while it took 180 min under mechanical shaking. Similar results were reported by Liu et al. [Citation24] and Bansode et al. [Citation13]: the former found that the hydrolysis reaction rate of triacylglycerides under UI was about 2-fold higher than that under shaking bath process, while the latter found that the maximum time required for isoamyl butyrate conversion was shortened to 3 h in sonication compared with 10 h of stirred reactor. The improvement of reaction efficiency was mainly attributed to the cavitational effect generated by UI [Citation15]. It is well-known that bubbles are recurrently formed and collapsed under acoustic wave, facilitating the mass transfer of the substrates and resulting in accelerated reaction rate. In addition, the increase in the rate of conversion may partially be ascribed to the conformational changes of lipase induced by UI [Citation25]. The present results suggested that ultrasonic treatment could improve the reaction rate of esterification of EA.
Figure 2. Conversion of EA as a function of time (min) with UI (150 W ultrasonic power and 28 kHz ultrasonic frequency) and without UI (mechanical shaking mixing at 150 rpm).
Note: The reactions were catalyzed by Novozyme® 435 with 6% enzyme loading and 4:1 molar ratio of ethanol to acetic acid. Readings were taken in triplicates and values were expressed as mean ± SD.
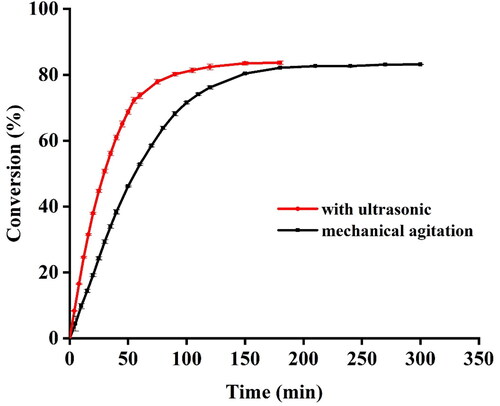
Effect of ultrasonic power on the conversion of EA
To evaluate the impact of ultrasonic power on the rate and conversion of EA synthesis, reactions were conducted under different power ranging from 60 W to 180 W. For the conversion of EA synthesis, an increase in the degree of conversion was observed as the power of ultrasound increased from 60 W to 150 W, and gained optimal conversion at 150 W. However, the final conversion of EA decreased as the power of ultrasound further increased to 180 W (). For the rate of reaction, the rate of EA synthesis slightly increased with increase in power from 60 W to 180 W. The results were consistent with those of previous studies, suggesting that an increase in ultrasonic power supply to an extent can lead to an increase in the rate and conversion, while further increase will slow down the progress of reaction [Citation26,Citation27]. Since the magnitude of the cavitation effect depends on the degree of intensity, ultrasound at high power will trigger more effective collapse of bubbles and produce a sufficient shock wave, thus improving mass transfer of substrates [Citation15]. The phenomenon of decrease in the rate and yield of the reaction at higher power were probably ascribed to the extreme temperature and pressure generated by the violent implosion of bubbles, resulting in deactivating the enzyme [Citation28].
Effect of ultrasonic frequency on the conversion of EA
Ultrasonic waves are typically defined as the frequency above 20 kHz which cannot be detected by human hearing. When ultrasonic waves pass through the reaction medium, the periodic compression and rarefaction cause mechanical vibration of the liquid, leading to formation of voids or bubbles, which subsequently grow and further implode [Citation15]. The frequency of UI has a significant effect on the duration of compression and rarefaction cycle which will affect the size and shape of bubbles, ultimately impact the cavitational effect [Citation29].
The effects of ultrasonic frequency on the rate and conversion of EA synthesis were explored. For the rate of synthesis, the initial rate of reaction decreased as the frequency increased from 28 kHz to 135 kHz. For the degree of conversion, the final EA yield under 28 kHz was greater than those under 50 kHz and 135 kHz (). The present study indicated that an increase in ultrasonic frequency will hamper the efficiency of enzyme-catalyzed EA synthesis. Similar results were also observed in ultrasound-assisted production of troxerutin ester [Citation30] and methyl caffeate [Citation14]. It was reported that the expansion of alternative compression and rarefaction cycle was reduced under higher frequency, and such shorter expansion time resulted in the formation of small, intense and stable bubbles which were ineffectively imploded and generated a weak cavitation effect in the reaction [Citation31]. Taken together, we selected 28 kHz as the appropriate frequency for the subsequent experiments.
Effect of enzyme source on the conversion of EA
The structure of enzymes under UI tends to be flexible, which will lead to changes in the catalytic performance [Citation15]. It was reported that lipases produced from Burkholderia cepacia and Pseudomonas fluorescens, which have been immobilized onto Novozyme 435® and LipozymeTL IM®, respectively, were subjected to conformational changes under lower-intensity ultrasonic treatment, including increase in random coil fraction and decrease in α-helix and β-sheets structures [Citation32]. Shan and Gupta found that the secondary structure of UI pre-treated lipase was slightly changed while the tertiary structure of that was perturbed [Citation33].
To examine the effects of different enzyme sources on the ultrasound-assisted enzymatic reaction, EA was synthesized with three commercial immobilized enzymes: Novozyme 435®, Lipozyme TL IM® and Lipozyme RM IM®, respectively (). The EA yield with Novozyme 435® as catalyst was higher than those with Lipozyme TL IM® and Lipozyme RM IM® as catalysts, indicating that the Novozyme 435® is an appropriate immobilized enzyme for the synthesis of EA under UI. It is speculated that the catalytic performance of the immobilized enzyme is altered due to the conformational changes induced by ultrasonic treatment, which is different from that of free lipase [Citation15]. Such structural changes deserve further study.
Effect of enzyme loading on the conversion of EA
Apart from the slow reaction rate, another drawback of using enzymes as catalysts is the high cost of enzymes [Citation34]. Although numerous strategies have been attempted to reduce the cost of enzyme, the most direct way is to optimize the enzyme loading. To gain the optimal enzyme concentrations, EA was synthesized by using immobilized enzyme at 1–7% (w/v) with ultrasonic power of 150 W and ultrasonic frequency of 28 kHz. The yield of EA gradually increased as the lipase loading increased from 1% to 6%. In contrast, an obvious decrease in conversion of EA was detected when the lipase loading was increased to 7% (). A similar result was reported by Zhao et al. [Citation35], who found that the degree of polymerization was improved with 15% lipase loading, while lowered with 20% lipase loading. The possible explanation for this phenomenon was steric hindrance induced by excessive enzyme supplementation. It was reported that crowding of enzyme tends to cause aggregation thus limiting the diffusion of substrate and reducing the mass transfer at the surface of the enzyme [Citation15]. Another possible explanation was that the large amount of the carrier brought by the immobilized enzyme will scatter the ultrasonic wave and therefore lead to energy loss [Citation15].
Effect of molar ratio of substrates on the conversion of EA
Although the molar ratio of ethanol to acetic acid of 1:1 is stoichiometric requirement of EA synthesis, an excess of ethanol was always pursued to drive the equilibrium towards EA formation in view of esterification being a reversible reaction [Citation1]. In the present study, the molar ratio of ethanol to acetic acid ranging from 1:1 to 5:1 was investigated so as to maximize the conversion of EA under UI. For the yield of EA, it can be seen that the formation of EA increased as the molar ratio of ethanol to acetic acid increased from 1:1 to 4:1, whereas decreased when the molar ratio was 5:1 (). The results are in agreement with those previously reported by Khan et al. [Citation36], in which conversion of cetyl oleate with 2:1 cetyl alcohol:oleic acid molar ratio was significantly higher than that with 4:1 molar ratio. The decrease in the conversion of EA synthesis could be due to the excessive ethanol accumulated in the vicinity of the enzyme, reaching a concentration level sufficient to cause enzyme inactivation [Citation37].
Kinetic study
A series of experiments were conducted by varying the concentration of acetic acid ranging from 0.10 to 0.74 mol/L, with keeping the concentration of ethanol at 0.9 or 1.2 mol/L with or without UI to estimate the parameters of enzyme kinetics. depicts plots of reciprocal initial rates of esterification versus reciprocal acetic acid concentrations. An increase in the initial rate of conversion was observed when the acetic acid concentration increased from 0.1 to 0.4 mol/L (). As the concentration of acetic acid further increased above 0.4 mol/L, a significant decrease in the initial reaction rate was observed, indicating that there is an inhibitory effect at higher concentrations of acetic acid. It could be perceived from that the slope and the minimum value of curve (A) decreased compared with those of curve (B), suggesting that UI treatment could increase the rate of reaction. When the fixed ethanol concentration increased to 1.2 mol/L, a similar trend was observed ( (II)).
Figure 8. Reciprocal initial rates of EA synthesis as a function of reciprocal acetic acid concentrations.
Note: Lipase catalyzed EA synthesis was conducted under UI at fixed ethanol concentration of 0.9 mol/L (A) or 1.2 mol/L (A′), or under mechanical shaking at fixed ethanol concentration of 0.9 mol/L (B) or 1.2 mol/L (B′). Conditions: 150 W ultrasonic power, 28 kHz ultrasonic frequency, 6% enzyme loading. Readings were taken in triplicates and values were expressed as mean ± SD.
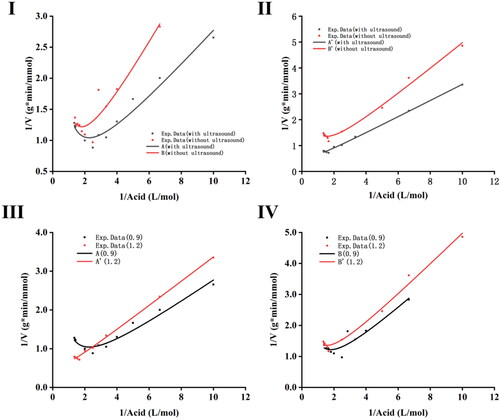
(III and IV) depict the comparative analysis of the results obtained with or without UI at 0.9 or 1.2 mol/L fixed ethanol concentration studies. The reaction rates under mechanical shaking or UI at 1.2 mol/L ethanol concentration were lower compared with those at 0.9 mol/L ethanol concentration, suggesting that the rate of EA synthesis was inhibited by ethanol. The present results clearly demonstrated that not only the substrate acetic acid, but also the substrate ethanol had an inhibitory action on the reaction.
It is generally recognized that esterification catalyzed by lipase follows the Bi-bi ping-pong mechanism [Citation38,Citation39], which includes the following steps: firstly, the enzyme reacts with acetic acid to form an acyl-enzyme complex and release water; secondly, the acyl-enzyme complex interacts with ethanol to yield ester and release enzyme [Citation23]. It was reported that ethanol may competitively bind to enzyme to inhibit the formation of acyl-enzyme complex [Citation39,Citation40]. The interaction of an acid with the acyl-enzyme complex could lower the yield of esters by forming a dead-end acid-enzyme-acyl complex [Citation23]. In this regard, modified formulation modeled from the Ping-Pong Bi-Bi mechanism with substrates inhibition was employed to evaluate the kinetic constants. shows the kinetic parameters calculated by using EquationEquation (4)(4)
(4) . The Vmax under UI was increased by 143% compared with that under mechanical shaking, suggesting that ultrasound could elevate the rate of reaction. It was speculated that the wave shock generated by the cavitational effect of ultrasound could increase the mass transfer and decrease the activation energy, resulting in improving the interaction of substrate and enzyme and the formation of intermediate complex [Citation16]. Such effect was evidenced by a decrease in Kacetic acid and increase of Kethanol under UI compared with those under mechanical agitation, implicating that the enzyme was prone to form an acyl-enzyme complex rather than a dead-end ethanol-enzyme complex. In addition, the higher value of Ki acetic acid and lower value of Ki ethanol under UI showed that the inhibitory effect of acetic acid was elevated, while that of ethanol was reduced [Citation36]. This was possibly attributed to the greater affinity of lipase toward acetic acid under UI, which may lead to accumulation of acetic acid at the microenvironment of enzyme thereafter exert inhibitory effect on the enzyme [Citation41].
Table 1. Comparison table of enzymatic kinetic parameters under study.
Conclusions
The present study showed that ultrasound irradiation could significantly shorten the time of lipase-catalyzed synthesis of ethyl acetate compared with mechanical shaking. The optimal conditions of ultrasound-assisted reaction by using Novozyme 435® were 6% enzyme loading, 4:1 molar ratio of ethanol to acetic acid, 150 W & 28 kHz ultrasonic power and frequency, respectively. The kinetic study indicated that ultrasound accelerated the rate of reaction and this was mostly mediated by enhancing the affinity of acetic acid toward the enzyme and alleviating the inhibitory action of ethanol.
Disclosure statement
No potential conflict of interest was reported by the authors.
Data availability statement
The data supporting the findings reported in this study are available from the corresponding author upon reasonable request.
Additional information
Funding
References
- LÖser C, Urit T, Bley T. Perspectives for the biotechnological production of ethyl acetate by yeasts. Appl Microbiol Biotechnol. 2014;98(12):5397–5415.
- Fan GS, Teng C, Xu D, et al. Improving ethyl acetate production in Baijiu manufacture by Wickerhamomyces anomalus and Saccharomyces cerevisiae mixed culture fermentations. Biomed Res Int. 2019; 2019:1470543.
- Fu ZL, Sun BG, Li XT, et al. Isolation and characterization of a high ethyl acetate-producing yeast from Laobaigan Daqu and its fermentation conditions for producing high-quality Baijiu. Biotechnol Biotec Eq. 2018;32(5):1218–1227.
- Fan GS, Teng C, Xu D, et al. Enhanced production of ethyl acetate using co-culture of Wickerhamomyces anomalus and Saccharomyces cerevisiae. J Biosci Bioeng. 2019;128(5):564–570.
- Fan GS, Fu ZL, Teng C, et al. Comprehensive analysis of different grades of roasted-sesame-like flavored Daqu. Int J Food Prop. 2019;22(1):1205–1222.
- Xu YQ, Sun BG, Fan GS, et al. The brewing process and microbial diversity of strong flavour Chinese spirits: a review. J Inst Brew. 2017;123(1):5–12.
- Kruis AJ, Levisson M, Mars AE, et al. Ethyl acetate production by the elusive alcohol acetyltransferase from yeast. Metab Eng. 2017;41:92–101.
- Kovalenko GA, Perminova LV, Beklemishev AB. Catalytic properties of recombinant Thermomyces lanuginosus lipase immobilized by impregnation into mesoporous silica in the enzymatic esterification of saturated fatty acids with aliphatic alcohols. Reac Kinet Mech Cat. 2019;128(1):479–491.
- Waghmare GV, Mudaliar C, Rathod VK. Optimization of the enzyme catalyzed ultrasound assisted synthesis of cinnamyl butyrate using response surface methodology. Reac Kinet Mech Cat. 2020;129(1):421–441.
- Cui JD, Jia SR. Organic–inorganic hybrid nanoflowers: a novel host platform for immobilizing biomolecules. Coordin Chem Rev. 2017;352:249–263.
- Prajapat AL, Subhedar PB, Gogate PR. Ultrasound assisted enzymatic depolymerization of aqueous guar gum solution. Ultrason Sonochem. 2016;29:84–92.
- Liu SL, Dong XY, Wei F, et al. Ultrasonic pretreatment in lipase-catalyzed synthesis of structured lipids with high 1,3-dioleoyl-2-palmitoylglycerol content. Ultrason Sonochem. 2015;23:100–108.
- Bansode SR, Rathod VK. Ultrasound assisted lipase catalysed synthesis of isoamyl butyrate. Process Biochem. 2014;49(8):1297–1303.
- Wang J, Wang S, Li Z, et al. Ultrasound irradiation accelerates the lipase-catalyzed synthesis of methyl caffeate in an ionic liquid. J Mol Catal B Enzym. 2015;111:21–28.
- Bansode SR, Rathod VK. An investigation of lipase catalysed sonochemical synthesis: a review. Ultrason Sonochem. 2017;38:503–529.
- Bansode SR, Rathod VK. An intensified technique for lipase catalysed amide synthesis. Chem Eng Process. 2019;143:107605.
- Yao Y, Pan Y, Liu SQ. Power ultrasound and its applications: a state-of-the-art review. Ultrason Sonochem. 2020;62:104722
- Bhangu SK, Gupta S, Ashokkumar M. Ultrasonic enhancement of lipase-catalysed transesterification for biodiesel synthesis. Ultrason Sonochem. 2017;34:305–309.
- Murillo G, Ali SS, Sun JZ, et al. Ultrasonic emulsification assisted immobilized Burkholderia cepacia lipase catalyzed transesterification of soybean oil for biodiesel production in a novel reactor design. Renew Energ. 2019;135:1025–1034.
- Vasic-Racki D, Kragl U, Liese A. Benefits of enzyme kinetics modelling. Chem Biochem Eng Q. 2003;17:3–14.
- Lan WB, Chen S. Chemical kinetics, thermodynamics and inactivation kinetics of dextransucrase activity by ultrasound treatment. Reac Kinet Mech Cat. 2020;129(2):843–864.
- Cui FJ, Zhao HX, Sun WJ, et al. Ultrasound-assisted lipase-catalyzed synthesis of D-isoascorbyl palmitate: process optimization and Kinetic evaluation. Chem Cent J. 2013;7(1):180
- Kuperkar VV, Lade VG, Prakash A, et al. Synthesis of isobutyl propionate using immobilized lipase in a solvent free system: optimization and kinetic studies. J Mol Catal B Enzym. 2014;99:143–149.
- Liu Y, Jin Q, Shan L, et al. The effect of ultrasound on lipase-catalyzed hydrolysis of soy oil in solvent-free system. Ultrason Sonochem. 2008;15(4):402–407.
- Fernandez-Lafuente R. Lipase from Thermomyces lanuginosus: uses and prospects as an industrial biocatalyst. J Mol Catal B Enzym. 2010;62(3-4):197–212.
- Waghmare GV, Rathod VK. Ultrasound assisted enzyme catalyzed hydrolysis of waste cooking oil under solvent free condition. Ultrason Sonochem. 2016;32:60–67.
- Guo PM, Zheng C, Huang FH, et al. Ultrasonic pretreatment for lipase-catalyzed synthesis of 4-methoxy cinnamoyl glycerol. J Mol Catal B Enzym. 2013;93:73–78.
- Özbek B, Ülgen KÖ. The stability of enzymes after sonication. Process Biochem. 2000;35(9):1037–1043.
- Patist A, Bates D. Ultrasonic innovations in the food industry: from the laboratory to commercial production. Innov Food Sci Emerg. 2008;9(2):147–154.
- Xiao Y, Yang L, Mao P, et al. Ultrasound-promoted enzymatic synthesis of troxerutin esters in nonaqueous solvents. Ultrason Sonochem. 2011;18(1):303–309.
- Price GJ. Recent developments in sonochemical polymerization. Ultrason Sonochem. 2003;10(4-5):277–283.
- Nadar SS, Rathod VK. Sonochemical effect on activity and conformation of commercial lipases. Appl Biochem Biotechnol. 2017;181(4):1435–1453.
- Shan S, Gupta MN. The effect of ultrasonic pre-treatment on the catalytic activity of lipases in aqueous and non-aqueous media. Chem Cent J. 2008;2:1.
- Wang Y, Zhao D, Triantafyllidis KS, et al. Microwave-assisted catalytic upgrading of bio-based furfuryl alcohol to alkyl levulinate over commercial non-metal activated carbon. Mol Catal. 2020;480:110630.
- Zhao X, Bansode SR, Ribeiro A, et al. Ultrasound enhances lipase-catalyzed synthesis of poly (ethylene glutarate). Ultrason Sonochem. 2016;31:506–511.
- Khan NR, Jadhav SV, Rathod VK. Lipase catalysed synthesis of cetyl oleate using ultrasound: optimisation and kinetic studies. Ultrason Sonochem. 2015;27:522–529.
- Musa N, Latip W, Abd Rahman RNZ, et al. Immobilization of an antarctic Pseudomonas AMS8 lipase for low temperature ethyl hexanoate synthesis. Catalysts. 2018;8(6):234.
- Gupta SM, Kamble MP, Yadav GD. Insight into microwave assisted enzyme catalysis in process intensification of reaction and selectivity: kinetic resolution of (R,S)-flurbiprofen with alcohols. Mol Catal. 2017;440:50–56.
- Bhavsar KV, Yadav GD. Process intensification by microwave irradiation in immobilized-lipase catalysis in solvent-free synthesis of ethyl valerate. Mol Catal. 2018;461:34–39.
- Aguieiras ECG, de Barros DSN, Sousa H, et al. Influence of the raw material on the final properties of biodiesel produced using lipase from Rhizomucor miehei grown on babassu cake as biocatalyst of esterification reactions. Renew Energ. 2017;113:112–118.
- Devi NA, Radhika GB, Bhargavi RJ. Lipase catalyzed transesterification of ethyl butyrate synthesis in n-hexane – a kinetic study. J Food Sci Technol. 2017;54(9):2871–2877.