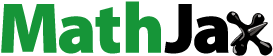
Abstract
Methods for prediction of fluid responsiveness are needed during shock resuscitation. Fluid therapy remains a cornerstone in the treatment of shock and influences the outcomes directly. Excess or insufficient fluid administration is associated with increased morbidity and mortality. Prediction of fluid responsiveness means that a hemodynamic variable is used to determine how likely a patient is to respond to fluid bolus with a significant increase in their cardiac output. Depending on the response to fluids, patients are either responders or non-responders. Clinicians often rely on static indices of preload, like central venous pressure and pulmonary artery occlusion pressure, as a guide for fluid therapy. Unfortunately, whilst easy for use, these indices are of minimal value as predictors of fluid responsiveness. More recent research highlights hemodynamic variables related to cardiopulmonary interactions during mechanical ventilation. These dynamic indices, viz. stroke volume variation and pulse pressure variation, show a significantly better predictive value. To maximize the predictive value of dynamic indices, several conditions must be fulfilled. Another method for prediction of fluid responsiveness is represented by the functional hemodynamic tests: a heterogenous group of bedside tests for preload responsiveness. Fluid challenges remain popular, although repetitive use can be harmful. Hemodynamic tests, like passive leg raising or end-expiratory occlusion, modify the preload without fluid administration. Regardless of the test used, monitoring of cardiac output is needed to evaluate the heart’s response to changes in preload. This review gives an overview of the methods for fluid responsiveness prediction, including those explored in the COVID-19 context.
Introduction
Infusion therapy is a medication; it has a dose, indications and side effects. Unfortunately, fluid therapy has a narrow therapeutic index, which makes over- and under-dosing easy. The higher the patient fragility, the more complex the illness and the more severe the condition, the more likely is for patient harm to ensue due to unintentional fluid overload or restriction. This warrants particular attention to optimizing and individualizing infusion therapy in the perioperative setting. Scientific data show that both restrictive and liberal protocols for fluid therapy are associated with increased rate of complications [Citation1]. Because of this, in the last 20 years, a significant body of research was aimed at developing new methods for fluid responsiveness prediction [Citation2]. The initial work of Marik et al. [Citation3] defined fluid responsiveness as an increase in cardiac output by 10% or more after a fluid bolus. According to these authors, fluid loading should be initialized only if an increase of cardiac output is needed and likely to be acquired. Of course, if there is obvious fluid loss, actual or relative (like initial phase of septic shock or exsanguination), administration of fluids should not be delayed. However, after initial resuscitation there is a phase where it is not evident if and how much more fluid is needed. In such cases fluid prediction is necessary to avoid complications. For instance, the published Marik-Phillips curve [Citation4] shows that administering fluids when the heart functions on the flat slope of the Frank-Starling curve leads to a steep increase in extravascular lung water (EVLW). Research focussed on prediction of fluid responsiveness followed naturally after hundreds of studies stated that static indices of preload like central venous pressure (CVP) have an extremely low predictive value [Citation5–7]. The aim of this review is to look at different available methods for predicting fluid responsiveness, as well as their positive and negative sides.
Static indices of preload
These are the central venous pressure (CVP), pulmonary artery occlusion pressure (PAOP), left ventricle end-diastolic pressure (LVEDP), left ventricular end-diastolic area (LVEDA), left ventricular end-diastolic volume (LVEDV), global end-diastolic volume (GEDV), inferior vena cava diameter and other related variables indicative of the momentary heart preload.
CVP is the most popular of all, because it is well known, easily obtainable and has a long tradition of use in the operating room OR and intensive care unit (ICU). CVP is used as a preload index of the right ventricle and to a lesser extent of the left ventricle. The following basic physiology principles in the end-diastole CVP equalizes consecutively to the end diastolic pressure in the right ventricle, pulmonary artery, pulmonary capillaries, left atrium and left ventricle. LVEDP is related to LVEDV, which determines the LV preload. Based on the Frank-Starling law, higher preload means higher stroke volume up to a certain point. Having these assumptions in mind it is appealing to conclude that higher CVP means higher cardiac output up to a certain threshold. Official guidelines state a CVP value of at least 8 mmHg should be achieved in resuscitation of septic shock [Citation8]. However, a more complex analysis of the above mentioned warrants some criticism.
The problems with the use of CVP start with its measurement [Citation9]. In order to measure CVP a central venous catheter needs to be placed, which is an invasive manipulation and can lead to complications. The anatomic location for the measurement of CVP is the junction of vena cava and the right atrium, that is where the central venous catheter tip should be positioned. Manipulation of the catheter by staff, passive or active patient repositioning can change the catheter tip position leading to false measurement of CVP [Citation10].
Furthermore, it is known that in patients with heart conditions (valve lesions, pulmonary hypertension, etc.) CVP differs significantly from the LVEDP [Citation11]. More importantly the LVED pressure-volume relationship is unpredictable in different patients and depends on factors such as heart cavity dimensions and compliance. Inter-individual variations in the slope and shape of the LVED pressure-volume curve make clinical interpretation difficult. Another problem when using CVP as a guide for fluid therapy becomes evident if we remember the Gyuton’s concept of venous return [Citation12]. According to EquationEquation (1)(1)
(1) venous return is modified by CVP.
(1)
(1)
Where VR is venous return; MSFP is mean systemic filling pressure and Vres is venous resistance.
Low CVP values increase the venous return to the heart. Because the heart can pump only as much blood as is returned to it, cardiac output is increased by augmentation of venous return. The heart pump has a permissive role on venous return and if CVP rises during loading with fluid that may mean the heart is not capable of taking all the blood that comes [Citation13]. That is why a trend of rising CVP values during fluid loading is not indicative of increasing cardiac output.
A large body of evidence dating since the 80 s, several hundred papers, experimentally show that CVP has a low predictive value for fluid responsiveness [Citation5–7]. It should not be neglected that CVP depends on so many factors (volume status, venous capacitance, venous resistance, inotropic state of the heart, tricuspid regurgitation, mechanical ventilation parameters, etc.) that clinical interpretation becomes challenging [Citation5,Citation6]. In addition, the already mentioned physiologic regards underline that it is generally inappropriate to use filling pressure as an index for filling volume and more importantly as an index for the future reaction of increased preload.
In spite of the presented limitations, CVP has its role in evaluating haemodynamics. Low CVP values (<6 mmHg) are related to increased likelihood for fluid responsiveness [Citation14]. On the other hand, high values (>15 mmHg) increase the risk of peripheral oedema formation and lower the organ perfusion pressure according to EquationEquation (2)(2)
(2)
(2)
(2)
OPP – organ perfusion pressure; MAP – mean arterial pressure.
Therefore, high CVP values may be used as an indicator to hold back volume loading [Citation14]. Unfortunately, the majority of patients have a midrange CVP, which is non-informative. In conclusion, CVP is an important variable and should be used in addition to other data in complex patient evaluation. Nevertheless, CVP is generally a poor predictor of fluid responsiveness.
Pulmonary artery occlusion pressure (PAOP) is measured by catheterization of the pulmonary artery and occluding one of the second division branches. Because of the anatomical proximity to the left heart, PAOP is a better estimate of LVEDP than CVP. However, it shares many of the limitations of CVP and, in addition, the procedure to measure it is highly invasive. PAOP also is confirmed to be a poor predictor of fluid responsiveness [Citation15]. That is not surprising, having in mind that LVEDP is a poor predictor itself [Citation16]. Even LVEDV cannot be used for this purpose because the relationship between left ventricle filling and LVEDV is dependent on heart cavities characteristics and inotropic state, which are different between individuals and at different stages of illness [Citation4]. Static hemodynamic parameters, including vena cava dimensions also have a low predictive value for the response to volume loading, despite being generally informative for preload [Citation17].The predictive value of some popular static parameters is shown in .
Table 1. Predictive value of static preload indices using data from Marik et al. [Citation3].
Dynamic hemodynamic indices
Initial data for respiratory variation of hemodynamic variables and their relation to volume status come from dog experiments in the early 80 s [Citation18]. Thirty years later these papers served as a basis for human studies. The concept for prediction of fluid responsiveness was developed by Marik et al. [Citation3] summarizing available scientific data. Used in septic patients for a start, this concept turned out to be generally applicable to mechanically ventilated patients and gained popularity in the international community of anaesthesiology and intensive care. Fluid responsiveness is defined as an increase in cardiac output by 10% or more after administration of fluid bolus (about 6 mL/kg body weight) [Citation3]. Prediction of fluid responsiveness is conducted by measuring respiratory variation of hemodynamic parameters, such as stroke volume variation (SVV) [Citation19], pulse pressure variation (PPV) [Citation20] and systolic pressure variation (SPV) [Citation21] caused by heart-lung interactions during mechanical ventilation. Intermittent positive pressure lung inflation causes a rise in intrathoracic pressure, which leads to a certain amount of blood being squeezed out of the pulmonary circulation into the left heart with every tidal breath. That amount of blood is believed to be roughly 100–150 mL. According to the Frank-Starling law this is followed by a slight increase in left ventricle’s stroke volume (SV) during inspiration if the ventricle is fluid responsive. Reciprocal changes take place during expiration. Therefore, if LV is on the steep portion of the Frank-Starling curve, SV varies to a higher degree, opposing to the case if LV is on the flat portion of the curve. A person in good health has a stroke volume variation (SVV) of approximately 10–15%.
SVV is automatically calculated by a monitor software using EquationEquation (3)(3)
(3)
(3)
(3)
Respiratory variation of stroke volume causes also respiratory variation in systolic pressure (SPV) and pulse pressure (PPV) because systemic vascular resistance does not change significantly during one breathing cycle. PPV is calculated in a similar fashion to SVV using EquationEquation (4)(4)
(4) .
(4)
(4)
More recent studies suggest that respiratory variation of the plethysmographic curve can also be used for fluid responsiveness prediction. The parameter of interest is called plethysmography variability index (PVi) [Citation22]. Significant scientific effort was aimed at respiratory variation of vena cava diameter, although results came out to disappoint. One big study reports an area under the ROC curve of 0.635 for this parameter [Citation23].
The predictive value for fluid responsiveness of some widely used dynamic parameters is shown in .
Table 2. Predictive value of dynamic hemodynamic indices according to meta-analyses.
Dynamic indices of preload show a very good correlation with fluid responsiveness. That allows for independent application of these indices without cardiac output measurement for confirmation.
The predictive value of dynamic indices is dependent on some well-known factors. In order to maximize the predictive value, a number of conditions must be fulfilled: regular heart rhythm, controlled mechanical ventilation with Vt at least 8 mL/kg predicted body weight (PBW), no spontaneous breaths, closed chest, no mechanical assist devices generating changes in the arterial pressure waveform (ventricular assist device, intra-aortic balloon pump), no abdominal hypertension [Citation3]. Some of these limitations can be overcome using simple tricks. For example, if lung protective ventilation is present, tidal volume can be briefly increased [Citation26]. If there is atrial fibrillation after cardiac surgery, temporary pacing can be instituted on slightly higher rate to achieve a regular rhythm. If there is an implanted intra-aortic balloon pump (IABP), it can be put on standby for one minute [Citation27].
Determining the right threshold of dynamic variables for fluid responsiveness is difficult in clinical practice. The cut off values vary between 8 and 15% in different reports [Citation28–30]. Thus, a grey zone interval must be anticipated where the predictive value is not optimal. Nevertheless, a method for overcoming this limitation has been proposed by Min et al. [Citation31]. They elevated the tidal volume from 8 to 12 mL/kg for individuals with a grey zone PPV and showed that PPV changed to a more informative value – the ‘grey zone approach’.
Overall, the dynamic variables are useful for fluid responsiveness evaluation when some necessary conditions can be met.
Functional hemodynamic tests
These are used to test the position of the heart on the Frank-Starling curve by the bedside. They are divided into two groups: fluid challenge tests and dynamic tests. Both need a pre- and post-intervention measurement of cardiac output to identify fluid responders. Therefore, the method of cardiac output measurement, its accuracy and speed of conduction matter significantly.
The most obvious way to test fluid responsiveness is to apply fluids. This is the standard or ‘maxi’ fluid challenge [Citation32]. Normally about 500 mL or a 6–8 mL/kg fluid bolus is delivered for approximately 10–30 min [Citation33]. Cardiac output is measured before and after the fluid bolus, and if there is an increase of at least 10%, the patient is identified as a fluid responder. Although still popular, the ‘maxi’ fluid challenge has important downsides. In a typical case it is needed to evaluate fluid responsiveness several times during the day and so repeated application of fluid challenge can be harmful [Citation2]. The so-called mini fluid challenge consists of infusion of a small amount of fluid, 100–150 mL for up to10 min [Citation34]. In this case, a smaller change of cardiac output is sought − 5%. The mini fluid challenge has a very good predictive value for fluid responsiveness ().
Table 3. Predictive value of hemodynamic functional tests.
The second group of tests, dynamic tests, modify preload in various ways without fluid loading. Probably most popular is the passive leg raise test (PLR) where the intervention consists of passive leg raising for about 5 min [Citation37]. That leads to an auto transfusion of about 500 mL from the peripheral to the central venous blood volume. Again, cardiac output is measured before and after and an increase of at least 10% is sought. Some authors have reported measuring a change in pulse pressure instead of cardiac output, although that lowers the predictive value of the PLR test [Citation38]. Echocardiographic measurement of cardiac output or its estimates such as left ventricle output tract velocity time integral and mitral inflow velocity have also been reportedly used to follow up the hemodynamic response to PLR [Citation39]. On the downside, PLR is difficult to perform intraoperatively. In many ICUs, beds can be positioned only in Trendelenburg position, which is not recommended because brain perfusion can be compromised. Using staff for passive leg raising can be impractical and is not always possible. Nevertheless, PLR is non-invasive, cheap and shows a very good predictive value for fluid responsiveness. It must be emphasized that PLR can be used in spontaneously breathing patients with irregular rhythm, as well as the fluid challenge tests.
Another functional test is the end-expiratory occlusion test (EEOT). It is performed the same way as measuring auto PEEP: the expiration hold function of the ventilator is used. Expiration hold is continued for 15–30 s (depending on the ventilator brand and model) and leads to a drop in intrathoracic pressure, which allows for an increase in venous return and a blood bolus to the right and left heart. If both ventricles are fluid responsive, the cardiac output will rise briefly. Because the time interval for cardiac output measurement is short, a continuous cardiac output monitoring technique must be used. EEOT was described for the first time by Monnet [Citation40].
Various other tests are proposed in the scientific literature, although studies are small, single-centered and evidence is scarce. Such tests use Valsalva manoeuvre, recruitment of the lungs, etc. for testing the preload reserve of the heart.
In summary, functional fluid responsiveness tests have very high predictive value and can be used in a wider population of patients in comparison to dynamic variables, including spontaneously breathing and arrhythmic patients.
Advanced hemodynamic monitoring platforms
There is a rapidly expanding market for hemodynamic monitoring systems supporting bedside fluid responsiveness evaluation [Citation41]. A number of commercially available devices can be used for measurement of dynamic indices of preload. These platforms usually incorporate the ability to measure cardiac output, too, which is needed not only to calculate SVV, but also to couple with dynamic hemodynamic tests. The monitoring systems can be graded in terms of their invasiveness as invasive (central venous line and arterial line needed), mini-invasive (arterial line needed) and non-invasive (no insertion of vascular catheters needed).
Dynamic variables like PPV and SVV are derived using arterial waveform analysis. Arterial waveform is obtained from an arterial catheter connected to a pressure sensor, which in turn converts the analogous pressure signal to digital and allows for software analysis of parameters of interest [Citation42,Citation43].
The gold standard method for cardiac output measurement is the pulmonary artery thermodilution (PATD) using a pulmonary artery catheter (PAC). A fluid bolus of known volume and temperature is injected in the proximal port of a pulmonary artery catheter. Thermal sensors detect the temperature change at the catheter tip positioned in the pulmonary artery over time. Afterwards software generates thermodilution curve and calculates the blood flow needed to produce it, effectively, that is the right ventricle CO. More recent monitors use transpulmonary thermodilution (TPTD). The same methodology is used, but temperature changes are detected in the femoral rather than in the pulmonary artery. PAC insertion is avoided that way. TPTD devices allow for measurement of useful parameters, such as global ejection fraction and extravascular lung water, which bring important information in sight [Citation44]. Cardiac output can be estimated also mini-invasively using pulse contour analysis of the arterial waveform and software incorporated calculation algorithms. That is referred to as uncalibrated cardiac output [Citation45]. Completely non-invasive measurement of cardiac output is possible in the most recent advancements of technology using the two finger-cuff technology. Arterial waveform is derived without arterial catheter using finger plethysmography [Citation46]. Although appealing, the mini- and non-invasive methods for CO measurement have a high error rate compared with thermodilution CO measurements, which makes them not reliable for the monitoring of critically ill patients. Future innovations improving the accuracy are eagerly awaited. Meanwhile companies have combined intermittent thermodilution and pulse contour analysis derived CO to create the so-called continuous calibrated cardiac output monitoring systems [Citation47].
Some of the well-known and widely used devices in the field of advanced hemodynamic monitoring are manufactured by the companies Edwards Lifesciences, Pulsion-Getinge and Lidco.
Edwards Lifesciences (Irvine, CA, USA) produce a number of clinical platforms for advanced monitoring. Hemosphere® is a device incorporating several monitoring systems, including a PAC and the Edwards FloTrac pressure sensor. Hemosphere® derives SVV, PPV and PATD CO. Continuous calibrated CO is also displayed. The EV1000® platform derives SVV and PPV using the Edwards VolumeView sensor. EV1000® provides also continuous calibrated monitoring of cardiac output using transpulmonary thermodilution (TPTD) coupled with pulse contour analysis of the femoral artery waveform. Vigileo® is another monitor which is dedicated to uncalibrated CO and SVV measurement using the Edwards FloTrac sensor. The Clearsight® system allows non-invasive determination of CO, SVV and PPV using two finger pressure cuffs and infrared light plethysmography.
Pulsion-Getinge (Feldkirchen, Germany) is another company known for a wide spectrum of hemodynamic monitors. Most popular and established is the PiCCO Technology®, which uses TPTD and arterial waveform analysis to deliver continuous calibrated cardiac output, as well as SVV and PPV. PiCCO Technology® is available as an integrated module for IntelliVue® and CMS® Patient Monitors from Philips Medical Systems. The Pulsioflex® monitoring system delivers measurement of SVV, PPV and uncalibrated CO using the Pulsion ProAQT sensor. As an advantage, there is a manual calibration option for cardiac output using a measurement obtained by a different modality, for example echocardiography. The non-invasive device of the company is represented by the NICCI Technology®, which allows for non-invasive estimation of SVV, PPV and uncalibrated CO.
Lidco (London, UK) is the producer of the LIDCO monitor. As opposing to the thermodilution method, LIDCO uses injection of lithium containing solution for CO measurement. The change in the lithium concentration over time is used to calculate the cardiac output. The LIDCO monitor comes in three modalities: a non-invasive (LIDCO Rapid®), a mini-invasive (LIDCO Plus®) and a calibrated one (LIDCO Unity®). Calibration can be done via lithium injection or using a manually entered value.
Massimo (Irvine, CA, USA) offers measurement of PVi® (plethysmography variability index). It is obtained non-invasively using infrared light pulse plethysmography. PVi is obtainable alongside Masimo SET® Pulse Oximetry and Rainbow® Pulse CO-Oximetry.
An interesting addition is the AJL Capstesia Biotablet®. It allows measurement of PPV using a photograph of any arterial waveform displayed on a monitor. It was originally offered as a mobile application, now it is available as a tablet containing the licensed software.
Fluid responsiveness in the COVID-19 era
The COVID-19 pandemic has presented a unique challenge for healthcare around the globe. The quality of care can be jeopardized by staff and resource shortages accompanying high patient volume load on hospitals during virus outbreaks. The methods for fluid responsiveness evaluation are generally valid for the COVID-19 patients, nevertheless specific considerations must be taken into account. Currently guidelines recommend a restrictive fluid strategy in COVID-19 patients with acute respiratory distress syndrome (ARDS) and vasopressor therapy as treatment in COVID-19 circulatory shock [Citation48]. Lack of sufficient nursing staff and resources directs clinicians towards simple, cheap and easy to use methods for fluid responsiveness prediction.
CVP measurement can be first to come into play, as most patients in severe condition already have a central line. The predictive value of very low CVP (below 6 mmHg) shows it is fairly likely that the patient is responsive to fluids. On the other hand, a high CVP value (above 15 mmHg) is a good predictor of fluid non-responsiveness [Citation14]. However, the majority of patients have a midrange CVP. They are often being ventilated with high PEEP or in prone position which makes CVP reliability even lower.
Dynamic hemodynamic variables can be useful in the COVID ICU when criteria can be met. If available, a Vigileo monitor can be set up to monitor SVV. PVi may also be measured using one of the Masimo devices discussed above. Having that in mind, it must be emphasised that many ARDS patients have very low lung compliance, which makes it difficult to ventilate with a tidal volume of 8 mL/kg [Citation49]. A lower predictive value of dynamic indices is to be anticipated in these patients.
Fluid challenges are associated with higher risk for circulatory overload when repeatedly done. The mini fluid challenge is more appealing in the setting of COVID-19 ARDS. Still cardiac output coupling is needed to evaluate response, which is problematic. If available, echocardiography can be used to detect a change in CO or LVOT VTI. Passive leg raise test, or alternatively Trendelenburg position, can be tried in combination with pulse pressure monitoring if CO cannot be measured.
Complex clinical platforms are cumbersome, and their use requires significant personnel effort and skill. Cardiac output thermodilution measurement is difficult in the COVID-19 ICU. An international survey shows that cardiac output is measured predominantly with echocardiography in Europe [Citation50]. A subgroup of patients with COVID-19 have significant myocardial depression due to myocardial injury, septic cardiomyopathy, or right ventricle dysfunction. Interestingly echocardiography revealed hyper-dynamic state (43%), hypovolemia (22%), a left ventricular dysfunction (21%) and a right ventricular dilation (20%) in critically ill COVID-19 patients [Citation50]. It might be appropriate to screen patients with poor response to initial treatment using echocardiography. Previous studies have pointed out ultrasound machine use as potential risk factor for virus spread [Citation51]. That can be minimized by dedicating one US machine for the needs of the COVID-19 area of the hospital. Another subset of COVID-19 patients (about 10%) present with accompanying diarrhoea [Citation52]. Appropriate rehydration must be ensured before application of advanced hemodynamic monitoring.
To summarize, currently no best tool for fluid responsiveness assessment in COVID-19 critical patients can be pointed out. Clinicians must evaluate the clinical context and the characteristics of each method to find the most appropriate solution for each particular case. Large-scale studies are needed in that clinical field.
Discussion
A review of the present methods for fluid responsiveness prediction shows that numerous alternatives are at hand depending on the patient condition, equipment availability and clinical setting. None of these methods has absolute sensitivity and specificity and they all have their positive and negative sides. The use of these methods warrants correct identification of most fluid responsive patients.
In addition, it should be emphasised that being fluid responsive is the normal state of circulation, which is no indication for fluid loading by itself. The methods for prediction of fluid responsiveness should be used together with all other available clinical data. For instance, if cardiogenic lung oedema is present, fluid loading would obviously not be wise even if the patient is fluid responsive.
Criticism has been addressed to the dynamic hemodynamic indices regarding the number of criteria needed for a good predictive value. While it is true that most of the intensive care patients do not meet all criteria according to the initial studies in that field, as stated before, simple techniques can be applied to overcome many of these limitations. These authors have obtained promising results from a study aimed at using stroke volume variation in patients with intra-aortic balloon pump (Getinge) after cardiac surgery [Citation27]. Other groups presented evidence that tidal volumes can be increased (‘tidal volume challenge’ if lung protective ventilation protocol is used) to optimize the SVV predictive value. Transcutaneous or intravenous ventricular pacing can be used to achieve regular rhythm if there is atrial fibrillation. Furthermore, all these conditions are needed for maximizing the predictive value of dynamic indices. These indices can be used to estimate the likelihood for fluid responsiveness without the above-mentioned conditions met, however one must then anticipate a lower predictive value. Thus, if SVV is extremely high or low (>20% or <5%) in a spontaneously breathing patient, it is most probably indicative for fluid responsiveness.
Regarding the functional hemodynamic tests, our research group has interesting preliminary results, not published yet. According to our findings a ‘mini’ fluid challenge has an even better predictive value than stoke volume variation, which confirms data from cited meta-analyses [Citation16,Citation36]. Furthermore, in our opinion, end expiratory occlusion testing is challenging practically, because cardiac output measurement must be done very quickly at the end of the test. Pulmonary artery thermodilution with manual injection for measurement like the Edwards Lifesciences Swan-Ganz catheter cannot be used for that purpose. Three consecutive measurements with similar thermodilution curves are required for accuracy, which is too slow for capturing the brief hemodynamic effect of EEOT. Thus, if EEOT is used in combination with pulmonary artery thermodilution, we would suggest conducting the test three times and measurement of cardiac output after each test. Alternatively and maybe more appropriately, a continuous calibrated cardiac output monitor such as Edwards Lifesciences EV1000 clinical platform or Pulsion – Getinge PICCO Technology could be chosen.
Finally, it cannot be overemphasized that the static indices of preload like CVP and PAOP are usually not informative of fluid responsiveness. That is especially true for mid-range values, which are most common in daily practice.
Conclusions
Based on the available data, dynamic hemodynamic indices and functional tests are good predictors of fluid responsiveness. They should be used in clinical scenarios where the need of fluids is not obvious. Static indices of preload should also be taken into account when determining the hemodynamic state of the circulation. However, their value as predictors of fluid responsiveness is limited.
Acknowledgements
The authors wish to thank Academician Prof. Dr. Venko Aleksandrov for his wise guidance during the writing process. The authors wish to thank all team members of the Cardiac Intensive Care Unit of University Hospital ‘Sveta Ekaterina’ for their dedication and hard work, which have been of enormous help for the present study.
Disclosure statement
The authors would like to declare that no financial interest or benefit has arisen from the direct applications of this review paper. The autohors have no conflicts of interest to declare.
Additional information
Funding
References
- Miller T, Myles P. Perioperative fluid therapy for major surgery. Anesthesiology. 2019;130(5):825–832.
- Monnet X, Marik P, Teboul JL. Prediction of fluid responsiveness: an update. Ann Intensive Care. 2016;6(1):111.
- Marik PE, Cavallazzi R, Vasu T, et al. Dynamic changes in arterial waveform derived variables and fluid responsiveness in mechanically ventilated patients: a systematic review of the literature. Crit Care Med. 2009;37(9):2642–2647.
- Marik P, Lemson J. Fluid responsiveness: an evolution of our understanding. Br J Anaesth. 2014;112(4):620–622.
- Cecconi M, Aya HD. Central venous pressure cannot predict fluid-responsiveness. Evid Based Med. 2014;19(2):63.
- Marik P, Cavalazzi R. Does the central venous pressure predict fluid responsiveness? An updated meta-analysis and a plea for some common sense. Crit Care Med. 2013;41:1774–1781.
- Saugel B, Kirsche S, Hapfelmeier A, et al. Prediction of fluid responsiveness in patients admitted to the medical intensive care unit. J Crit Care. 2013;28(4):537.e1–537.e9.
- Rhodes A, Evans L, Alhazzani W, et al. Surviving sepsis campaign: international guidelines for management of sepsis and septic shock: 2016. Crit Care Med. 2017;45(3):486–552.
- Shah P, Louis MA. 2021. Physiology, central venous pressure. Treasure Island (FL): StatPearls Publishing;
- Farcy D, Jain A, Dalley M, et al. Pitfalls in using central venous pressure as a marker of fluid responsiveness. Emerg Med. 2016;48(1):18–28.
- Weyland A, Grüne F. [Cardiac preload and central venous pressure]. Anaesthesist. 2009;58(5):506–512.
- Guyton AC, Jones C, Coleman T. 1973. Circulatory physiology: cardiac output and its regulation. 2nd ed. Philadelphia (PA): W.B. Saunders Co.; p. 430–431.
- Tansey EA, Montgomery LEA, Quinn JG, et al. Understanding basic vein physiology and venous blood pressure through simple physical assessments. Adv Physiol Educ. 2019;43(3):423–429.
- De Backer D, Vincent JL. Should we measure the central venous pressure to guide fluid management? Ten answers to 10 questions. Crit Care. 2018;22(1):43.
- Kumar A, Anel R, Bunnel E, et al. Pulmonary artery occlusion pressure and central venous pressure fail to predict ventricular filling volume, cardiac performance, or the response to volume infusion in normal subjects. Crit Care Med. 2004;32(3):691–699.
- Michard F, Teboul JL. Predicting fluid responsiveness in ICU patients: a critical analysis of the evidence. Chest. 2002;121(6):2000–2008.
- Eyre L, Breen A. Optimal volaemic status and predicting fluid responsiveness, continuing education in anaesthesia. Crit Care Pain. 2010;10(2):59–62.
- Coyle JP, Teplick R, Long M. Respiratory variations in systolic arterial pressure as an indicator of volume status. Anesthesiology. 1983;59:A53.
- Zhang Z, Lu B, Sheng X, et al. Accuracy of stroke volume variation in predicting fluid responsiveness: a systematic review and Meta-analysis. J Anesth. 2011;25(6):904–916.
- Teboul JL, Monnet X, Chemla D, et al. Arterial pulse pressure variation with mechanical ventilation. Am J Respir Crit Care Med. 2019;199(1):22–31. 1
- Mallat Pironkov JA, Destandau M, et al. Systolic pressure variation (δdown) can guide fluid therapy during pheochromocytoma surgery. Can J Anesth/J Can Anesth. 2003;50(10):998–1003.
- Cannesson M, Desebbe O, Rosamel P, et al. Pleth variability index to monitor the respiratory variations in the pulse oximeter plethysmographic waveform amplitude and predict fluid responsiveness in the operating theatre. BJA: Br J Anaesth. 2008;101(2):200–206.
- Millington SJ. Ultrasound assessment of the inferior vena cava for fluid responsiveness: easy, fun, but unlikely to be helpful. Can J Anaesth. 2019;66(6):633–638.
- Yang X, Du B. Does pulse pressure variation predict fluid responsiveness in critically ill patients? A systematic review and meta-analysis. Crit Care. 2014;18(6):650.
- Liu T, Xu C, Wang M, et al. Reliability of pleth variability index in predicting preload responsiveness of mechanically ventilated patients under various conditions: a systematic review and meta-analysis. BMC Anesthesiol. 2019;19(1):67.
- Myatra SN, Prabu N, Divatia J, et al. The changes in pulse pressure variation or stroke volume variation after a "tidal volume challenge" reliably predict fluid responsiveness during low tidal volume ventilation. Crit Care Med. 2017;45(3):415–421.
- Enev R, Abedinov F, Bakalova N, et al. Stroke volume variation is a good predictor of fluid responsiveness in cardiac surgery patients with implanted intra-arortic balloon pump (submitted for evaluation).
- Cannesson M. The “grey zone” or how to avoid the binary constraint of decision-making. Can J Anaesth. 2015;62(11):1139–1142.
- Biais M, Ehrmann S, Mari A, et al. Clinical relevance of pulse pressure variations for predicting fluid responsiveness in mechanically ventilated intensive care unit patients: the grey zone approach. Crit Care. 2014;18(6):587.
- Michard F, Chemla D, Teboul JL. Applicability of pulse pressure variation: how many shades of grey?Crit Care. 2015;19:144.
- Min JJ, Gil NS, Lee JH, et al. Predictor of fluid responsiveness in the ‘grey zone’: augmented pulse pressure variation through a temporary increase in tidal volume. BJA: Br J Anaesth. 2017;119(1):50–56.
- Vincent JL, Weil MH. Fluid challenge revisited. Crit Care Med. 2006;34(5):1333–1337.
- Toscani L, Aya HD, Antonakaki D, et al. What is the impact of the fluid challenge technique on diagnosis of fluid responsiveness? A systematic review and Meta-analysis. Crit Care. 2017;21(1):207.
- Biais M, Courson H, Lanchon R, et al. Mini-fluid challenge of 100 ml of crystalloid predicts fluid responsiveness in the operating room. Anesthesiology. 2017;127(3):450–456.
- Monnet X, Marik P, Teboul JL. Passive leg raising for predicting fluid responsiveness: a systematic review and meta-analysis. Intensive Care Med. 2016;42(12):1935–1947.
- Messina A, Dell’Anna A, Baggiani M, et al. Functional hemodynamic tests: a systematic review and a metanalysis on the reliability of end-expiratory occlusion test and of the mini-fluid challenge in predicting fluid responsiveness. Cric Care. 2019;23(1):264.
- Monnet X, Teboul JL. M. passive leg raising: five rules, not a drop of fluid!Crit Care. 2015;19:18.
- Lakhal K, Ehrmann S, Runge I, et al. Central venous pressure measurements improve the accuracy of leg raising-induced change in pulse pressure to predict fluid responsiveness. Intensive Care Med. 2010;36(6):940–948.
- El Hadouti Y, Valencia L, Becerra A, et al. Echocardiography and passive leg raising in the postoperative period: a prospective observational study. Eur J Anaesthesiol. 2017;34(11):748–754.
- Monnet X, Osman D, Ridel C, et al. Predicting volume responsiveness by using the end-expiratory occlusion in mechanically ventilated intensive care unit patients. Crit Care Med. 2009;37:951–956.
- Saugel B, Critchley L, Kaufmann T, et al. Journal of Clinical Monitoring and Computing end of year summary 2019: hemodynamic monitoring and management. J Clin Monit Comput. 2020;34(2):207–219.
- Sun S, Peeters W, Bezemer R, et al. On algorithms for calculating arterial pulse pressure variation during major surgery. Physiol Meas. 2017;38(12):2101–2121.
- Derichard A, Robin E, Tavernier B, et al. Automated pulse pressure and stroke volume variations from radial artery: evaluation during major abdominal surgery. Br J Anaesth. 2009;103(5):678–684.
- Argueta EE, Paniagua D. Thermodilution cardiac output: a concept over 250 years in the making. Cardiol Rev. 2019;27(3):138–144.
- Grensemann J. Cardiac output monitoring by pulse contour analysis, the technical basics of less-invasive techniques. Front Med (Lausanne). 2018;5:646.
- Saugel B, Hoppe P, Nicklas JY, et al. Continuous noninvasive pulse wave analysis using finger cuff technologies for arterial blood pressure and cardiac output monitoring in perioperative and intensive care medicine: a systematic review and meta-analysis. Br J Anesth. 2020;125(1):25–37.
- Della Rocca G, Costa MG, Pompei L, et al. Continuous and intermittent cardiac output measurement: pulmonary artery catheter versus aortic transpulmonary technique. Br J Anaesth. 2002;88(3):350–356.
- Alhazzani W, Evans L, Alshamsi F, et al. Surviving sepsis campaign guidelines on the management of adults with coronavirus disease 2019 (COVID-19) in the ICU: first update. Crit Care Med. 2021;49(3):e219–234.
- Gattinoni L, Chiumello D, Caironi P, et al. COVID-19 pneumonia: different respiratory treatment for different phenotypes?Intensive Care Med. 2020;46(6):1099–1102.
- Michard F, Malbrain M, Martin G, et al. Haemodynamic monitoring and management in COVID-19 intensive care patients: an international survey. Anaesth Crit Care Pain Med. 2020;39(5):563–569.
- Hasanin A, Mostafa M. Evaluation of fluid responsiveness during COVID-19 pandemic: what are the remaining choices?J Anesth. 2020;34(5):758–764.
- Wang F, Zheng S, Zheng C, et al. Attaching clinical significance to COVID-19-associated diarrhea. Life Sci. 2020;260:118312.